Cell Structure & Function (Cytology) PDF
Document Details
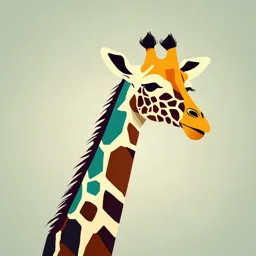
Uploaded by LucrativeThallium7340
Tags
Summary
This document is a chapter on cell structure and function, describing the components of cell membranes, different types of cellular transport, and the structure of eukaryotic cells.
Full Transcript
CHAPTER III CELL STRUCTURE & FUNCTION (CYTOLOGY) During the previous chapter we have examined the macromolecules of life (carbohydrates, lipids, proteins and nucleic acids), however, all those events are not taking place in the open, instead, they take place in cells in which are like containe...
CHAPTER III CELL STRUCTURE & FUNCTION (CYTOLOGY) During the previous chapter we have examined the macromolecules of life (carbohydrates, lipids, proteins and nucleic acids), however, all those events are not taking place in the open, instead, they take place in cells in which are like containers in varying sizes and shapes. Similar to any container, the first noticeable thing is the presence of boundaries around the cells or in biological terminology; MEMBRANES. MEMBRANE STRUCTURE When biological membrane structure is concerned, the first thing spring into mind is the basic component of this membrane: Lipids or more specifically PHOSPHOLIPIDS (see CHAPTERIII.PPT file: Slide 2). When the structure of a phospholipid is examined, one can easily noticed the charge separation in the molecule; the “head” portion is (+) charged and therefore HYDROPHILIC (likes water), and the “tail” portion is (-) charged and therefore HYDROPHOBIC (hates water) (Slide 3A). When these phospholipids made contact with water, they form a “theoretical monolayer” (Slide 3B), however they soon assume a BILAYER structure (Slide 4). This bilayer tends to form a closed bubble (Slide 4) (lipid vesicle) in water. A spherical bubble is the shape that minimizes the contact between water and the hydrophobic tails and is energetically the most stable configuration. Biological membranes consist of phospholipid bilayers with various sorts of PROTEINS interspersed in them and located on the membrane surfaces according to the widely accepted FLUID MOSAIC MODEL of membrane structure (Slide 5). MOVEMENT OF MATERIALS ACROSS MEMBRANES All materials (including the cellular solvent: H2O) move across membranes through highly regulated mechanisms. Of these mechanisms, three shall be mentioned here; 1) Osmosis 2) Simple Diffusion 3) Active Transport 1) OSMOSIS All types of membrane activities involve H2O and substances (solutes) dissolved in it. A membrane is said to be “permeable” to a substance if it allows free passage of that substance. The living cell membranes “is not openly permeable” to any substance, including, H2O, although H2O may cross more freely than most other substances. It is often stated that cellular membranes are SEMIPERMEABLE, meaning solvents but not solutes may cross. When a solute is more concentrated on one side of a membrane than the other, a CONCENTRATION GRADIENT exists. If a solvent is capable of traversing a membrane it will move from the side where the solute is more dilute (HYPOTONIC) (or alternatively speaking, where the free water is more), to the side of greater solute concentration (HYPERTONIC) (or alternatively speaking, where the free water is less) and will continue to move until the concentrations are equal across both sides of the membrane (ISOTONIC) (Slide 6). The process by which a solvent crosses a membrane in response to a concentration gradient is known as OSMOSIS and the force with which the solvent is drawn from dilute solute side to the concentrated side is the OSMOTIC PRESSURE. By using a biological system the process of osmosis can easily be observed (Animated Slide 7). If red blood cells (erythrocytes) are placed in solutions with different concentrations of ions; HYPERTONIC SOLUTION: Red blood cells lose water and shrink. HYPOTONIC SOLUTION: Red blood cells gain water and explode. ISOTONIC SOLUTION: Red blood cells remain unchanged. 2) SIMPLE DIFFUSION Many solutes (dissolved in the cellular solvent: H2O) cross membranes by simple diffusion, moving “DOWN” a concentration gradient from a higher concentration of solute side to the lower concentration side by the involvement of special membrane proteins (Animated Slide 8). Different kinds of molecules diffuse across membranes at different rates, depending on the properties of the molecules. For example, weak acids and weak bases cross membranes faster than the strong ones. In general the greater the charge on an ion, the less readily it will cross a membrane (e.g. Na+ and K+ pass easily than Ca+2 and Mg+2). The rate of diffusion through a membrane is known as FLUX. 3) ACTIVE TRANSPORT Unlike diffusion, active transport is able to achieve a net movement of a solute “UPHILL” against a concentration gradient. To accomplish this task the cell must use ATP as the energy source (Slide 9). Pushing substances out of the cell or pulling them in against a concentration gradient does not come cheap; in a resting human body, for example, 30-40% of all energy used goes in to active transport and the percentage may be much higher in certain organs such as brain and kidneys. Nearly all cells (either prokaryotic or eukaryotic) use active transport to pull valuable molecules like sugars. Expenditure of 1 ATP molecule to pull one molecule of glucose, for example, yields 38 ATP molecules, which is an extremely efficient trade. EUKARYOTIC CELL STRUCTURE All organisms except those in the Monera kingdom are composed of eukaryotic cells. Eukaryotic cells are characterized by internal membrane systems and by compartmentalization that subdivides the chemical activities. These membrane bound structures within eukaryotic cells are called ORGANELLES. Let’s first analyze a typical animal cell (Slide 10). NUCLEUS First noticeable organelle in animal cells (actually in all eukaryotic cells) is the NUCLEUS (nuclei; plural) (Slide 11). The cell’s nucleus is surrounded by the NUCLEAR ENVELOPE which consists of two layers of membranes with a narrow space between them. Macromolecules (mRNA, rRNA, nuclear proteins etc) can exit or enter the nucleus through PORES, which provide routes for communication with the surrounding cytoplasm. Within the nucleus, DNA is organized in linear arrays of NUCLEOSOMES (Slide 12), particles about 11 nm in diameter separated by stretches of DNA double helix. A nucleosome contains a relatively fixed length of DNA wrapped up with 8+1 sets of special proteins called HISTONES. The most obvious visual evidence of DNA organization within the nucleus is the CHROMOSOMES (Slide 12) which are further coiled (super coiled) DNA + Histone proteins which become visible during cell division. Within the nucleus, are one or more masses called NUCLEOLI (nucleolus; singular), (Slide 11) where rRNA is synthesized and stored prior to leaving the nucleus and combining with ribosomal proteins to form the ribosomes; the sites for protein synthesis. ENDOPLASMIC RETICULUM Immediately surrounding the nucleus is a network of flattened tubes called ENDOPLASMIC RETICULUM (Slide 13). When examined in close detail, this organelle is actually of two types: 1) ROUGH ENDOPLASMIC RETICULUM (RER) and 2) SMOOTH ENDOPLASMIC RETICULUM (SER). 1) RER This type of endoplasmic reticulum is called “rough” because its surface is studded with ribosomes. This is the place where proteins are synthesized by translation of the mRNA. Following their synthesis the proteins are modified and transported to other parts of the cell or outside the cell within small carrier vesicles (Slide 14). 2) SER In contrast to RER, the SER do not contain ribosomes and do not function in protein synthesis. When the content of the SER is examined, phospholipids, neutral fats, sterols and other lipids can easily be observed. This composition reflects the fact that SER is the site for lipid biosynthesis. GOLGI APPARATUS In most eukaryotic cells, one or more GOLGI BODIES (Apparatus) are present in the form of membranous sacs (Slide 15). Membranous sacs (transfer vesicles) from RER and SER bring proteins and lipids to the golgi body, where they are repackaged into secretory vesicles. These vesicles then move to the plasma membrane where their contents are expelled from the cell (Exocytosis) (Slide 14). Thus, actively secreting cells, such as pancreas gland, often have prominent golgi bodies which often go by the term GOLGI APPARATUS. In plant cells golgi bodies (usually called DICTYOSOMES) are involved in formation of the polysaccharide cell wall. Golgi bodies also carry on synthesis. In them, proteins from the RER are converted to glycoproteins, polysaccharides of various sorts and mucoproteins which form the mucus are synthesized. LYSOSOMES Lysosomes are membrane-bound organelles that contain a variety of powerful hydrolytic enzymes which catalyze the breakdown of large biological molecules (Slide 16). The separation of hydrolytic enzymes from the cytoplasm by the lysosome’s membrane protects the normal working components of a living cell from random destruction (digesting itself). Lysosomes vary in size and appearance, and probably derived either from the ER or Golgi bodies (or from both). MITOCHONDRIA Mitochondria act as power plants in the energy system of eukaryotic cells. The output of this energy production is the high compound called ATP (Adenosine triphosphate) (Slide 17). Mitochondria have double membrane system; the outer one is fairly typical, unspecialized UNIT MEMBRANE, but the inner one is highly specialized for ATP production. Elaborate folds of this inner membrane (called CRISTA) extend into the mitochondria’s interior gel-like matrix (called STROMA) (Slide 17). All the ATP synthesizing enzymes are located on the inner surfaces of crista. There are certain strange characteristics mitochondria displays. To start with, mitochondria have their own molecules of DNA but the amount is only enough to provide information for the synthesis of limited number of proteins; most mitochondrial proteins are actually come from the cytoplasm and are coded for by the DNA in the nucleus. Also mitochondria contain a protein synthesizing apparatus of their own complete with ribosomes. However, not only the DNA (gene structure and arrangement) but also the protein synthesizing apparatus with the ribosomes resembles more to bacterial systems rather than eukaryotic systems. Due to its similarity with bacteria both in terms of structure and composition, a theory called ENDOSYMBIOSIS THEORY is proposed to explain the origins of mitochondria (Slide 18, 19). According to this theory, mitochondria are the remnants of bacteria that were internalized by organisms less proficient at energy production early in the evolution of eukaryotic cells. And during the time course lost their bacterial properties. CHLOROPLASTS Chloroplasts are green organelles present only in photosynthetic cells of plants and in the cells of eukaryotic protists capable of photosynthesis (Slide 20). Each chloroplast is surrounded by an envelope consisting of two membranes. While the outside membrane is a typical unit membrane, inside, an internal membrane system is made up of specialized THYLAKOIDS elaborately organized into stacks of flat membrane sacs called GRANA (granum; singular). Embedded in the thylakoid membranes are enzymes and pigments essential for photosynthesis. Chloroplasts have their own DNA as well as protein synthesis apparatus complete with ribosomes. The ENDOSYMBIOSIS THEORY is also applicable to the explanation of the origins of chloroplasts (Slide 18, 19). Chloroplasts, like mitochondria, may have evolved from prokaryotic cells, possibly from cyanobacteria, which entered a non-photosynthetic cell and took up permanent residence and during the time course lost their bacterial properties. OTHER INTRACELLULAR ORGANELLES In addition to those organelles covered so far, a wide variety of other structures may be found in eukaryotic cells. Of these a few will be mentioned below. STORAGE GRANULES Unit membranes surround the storage granules found in many cells. Cells that export substances – the hormone producing cells of the endocrine glands, for example – often store their products in granules until something causes their release through the plasma membrane. In plants, we see AMYLOPLASTS and CHROMOPLASTS which store starch and non-photosynthetic pigments respectively (Slide 21). MICROBODIES Microbodies are similar in structure to lysosomes but are smaller. There are two major types: PEROXISOMES and GLYOXISOMES. Peroxisomes are found in both animal and plant cells and contain the enzyme called CATALASE. Certain cellular reactions that produce the highly toxic oxidizing agent hydrogen peroxide (H2O2) are confined to Peroxisomes, with catalase functioning to breakdown 2H2O2 2H2O + O2 Glyoxysomes are found in plant cells and also contain catalase which in this case split the H2O2 formed during the conversion of lipids to sugars specifically. CYTOSKELETON (Slide 22) Within the cytoplasm a network of protein fibers support cell shape and anchor organelles. Within these proteins ACTIN filaments are responsible for cell movement whereas MICROTUBULES (tubular polymers of the “tubulin” protein) not only involves in the cell division event but also assemble the pair of cylindrical 9X3 structures (CENTRIOLES) (Slide 23) located at right angles to each other. And finally the INTERMEDIATE FILAMENTS provide physical support network for the cell. CELLS AND TISSUES Cells within a multicellular eukaryotic organism are not simply packed together. They rather form layers and assemblies referred to as TISSUES of which all organs and larger structures are made. Organs of multicellular plants and animals are composed of one or more tissues, which in turn are composed of specific types of cells and extracellular materials. Although there are numerous tissues (some universal, some specific only to some organisms) three examples are given below. The cells of the outer surfaces of animals and those lining the small intestines make up the EPITHELIAL tissues (Slide 24). The cells that line the heart chambers and blood vessels make up the ENDOTHELIAL tissues (Slide 25). CONNECTIVE tissues (Slide 26) consist of both cells and intracellular materials. Cells called FIBROBLASTS produce the protein COLLAGEN, which forms a though but flexible organic framework in BONE CARTILAGE and TENDONS (where muscles connected to the bone). During development, different tissues chemically signal each other and join to construct a given organ. For example, skin (Slide 27) with its variety of cell types and structures is formed from the mutual interactions between the surface epithelium (the EPIDERMIS) and a deeper layer of connective tissue called DERMIS. Similar assemblies of cell types into tissues also occur in plants. Important structures in the development of plant tissues are the MERISTEMS (Slide 28A). Two types of meristems: Apical meristems are located in tips of roots and buds of shoots and responsible in Primary Growth; growth in length, whereas Lateral meristems are cylinders of dividing cells along the length of roots and shoots and responsible in Secondary Growth; growth in girth. Meristems are also responsible for the development of VASCULAR TISSUES (Slide 28B). Vascular tissues are composed of specialized tissues and cells of Xylem which are responsible for the movement of water and dissolved minerals from the soil up, and the Phloem which are responsible for the distribution of photosynthetic products within the plant and its movement to storage areas (sinks). -END OF CHAPTER III-