Chapter 3 Designing a STEM Project-Based Lesson.pdf
Document Details
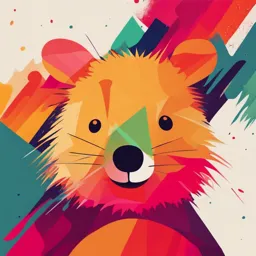
Uploaded by CuteBromeliad
Tags
Related
- Chapter 1 The STEM Project-Based Learning Paradigm Robert M. Capraro Department of Teaching, Learning & Culture Aggie STEM Texas A&M University.pdf
- Chapter 6 Classroom Management PDF
- Chapter 8 STEM Project-Based Learning in Inclusive Settings: Students With and at Risk of Disabilities.pdf
- Affordances of Virtual Worlds and Virtual Reality for STEM Project-Based Learning PDF
- Chapter 12 Using Free and Open-Source Hardware and Software With STEM Project-Based Learning PDF
- STEM Project-Based Learning - Aggie STEM PDF
Full Transcript
Chapter 3 Designing a STEM Project-Based Lesson Robert M. Capraro Mary Margaret Capraro Department of Teaching, Learning & Culture Department of Teaching, Learning & Culture Aggie STEM Aggie STEM Texas A&M Uni...
Chapter 3 Designing a STEM Project-Based Lesson Robert M. Capraro Mary Margaret Capraro Department of Teaching, Learning & Culture Department of Teaching, Learning & Culture Aggie STEM Aggie STEM Texas A&M University Texas A&M University Jamaal Young Luciana R. Barroso Department of Teaching, Learning & Culture Zachry Department of Civil and Environmental Aggie STEM Engineering Texas A&M University Aggie STEM Texas A&M University Do you remember learning how to skate? Or do you remember teaching someone how to skate? Learning to skate or teaching someone to skate is an iterative process where oftentimes the learner wants to “experiment” too quickly and the teacher tries to impart his/her wisdom so the learner does not make the same mistakes the teacher made when she or he first learned to skate. In the end, the learner probably has to repeat many of those same mistakes regardless, and, most importantly, no one is likely to brand any of their early attempts a failure just because the learner was not able to skate in the Olympics, play hockey, excel at Roller Derby, or even manage to stand up. Learning to teach project-based learning (PBL) effectively requires that an individual practice some of the patience and techniques needed to teach someone to skate. Specifically, they must have the patience to allow the learner to take control and to become more familiar with the techniques that build upon their expanding experience and knowledge base as a catalyst for accelerated learning. Science, technology, engineering, and mathematics (STEM) PBL has many applications, so learning to do it well can open up many venues one never imagined at the beginning of the journey. Just as in learning to skate—or learning to let the learner learn on his/her own—is not an all-or-nothing process. Learning to learn and learning to teach in a STEM PBL environment are not all-or-nothing propositions. 32 Designing a STEM Project-Based Lesson Chapter Outcomes When you complete this chapter, you should better understand and be able to explain how implementing PBL in the classroom occurs in stages and over time the ways in which PBL is informed by research on the design of learning environments and the learning sciences When you complete this chapter, you should be able to implement PBL components into your teaching use the Teacher PBL Checklist and understand the components of a PBL lesson discuss the underpinnings of PBL with other teachers and administrators Chapter Overview This chapter first discusses the theories that form the foundation of STEM PBL. We then explain which aspects of the learning environment influence the success of a STEM PBL lesson. Different stages of learning in STEM PBL are then discussed as well as how the learner functions during each of these. Finally, we explore the different components of a STEM PBL lesson and provide an example of how a teacher might construct each of these using the Teacher PBL Checklist Theoretical Underpinnings of STEM PBL STEM PBL is a special case of inquiry. Therefore, it is comprised of several theoretical frameworks that all interplay in the design, delivery, and assimilation of the content learned through STEM PBL. Primarily, all inquiry models are deeply rooted in constructivism and to some degree brain-based learning (Jumaat et al., 2017; Kokotsaki et al., 2016). However, STEM PBL adds enactivism, embodied cognition, social constructivism, and behaviorism. These theoretical models all interact to build a complex yet learner friendly experience to maximize learning while honoring individual students’ differences. The two independent, yet related, theories of constructivism and brain-based learning work symbiotically as the umbrella under which the other theories come into play through various components. Constructivism can be simplified as the act of allowing the learner to build their own knowledge, while brain-based learning theory is the understanding that everyone learns differently and that how each individual learn is inextricably connected to brain development, typically governed by age. Building from these two theories, STEM PBL makes use of learning activities where the learner is charged with individual accountability. The activities include tasks to accomplish that are in symbiosis with the learner’s stage of development and are brain friendly. For example, activities incorporate visuals, various ways to demonstrate student Designing a STEM Project-Based Lesson 33 knowledge, scaffolded learning tasks, and regular and predictable formative feedback. These two theories are active to greater and lesser degrees throughout each STEM PBL activity. The four theories that are activated as parts of various components are enactivism, embodied cognition, social constructivism, and behaviorism. Enactivism and embodied cognition are closely related ideas. Enactivism in its simplest form is the active cognitive process that occurs from engagement with the learning environment. This encompasses many aspects of STEM PBL, including the purposeful and unintentional actions of the teachers, students’ peers, and even the types of materials or activities presented. While this more passive theory puts a great deal of emphasis on the environment, it is paired with the purposeful theory of embodied cognition. The theoretical model of embodied cognition is applicable when a person’s entire body, beyond that of just the brain, plays a significant causal or physically constitutive role in cognitive processing. Therefore, the learning in this case is dependent on both the environment in which the body is active and the physical movements necessary to complete the tasks presented in that environment. If constructivism and brain-based learning are the umbrella under which STEM PBL’s key components are gathered, then social constructivism is the keystone that binds them together. None of the other learning theories incorporate the need for children to interact and learn from each other. This is complimentary in the classroom and can come from static observation, conversation, or the recursive listening/explaining process whether these are situated in an active or passive setting with or without any physical engagement. Finally, behaviorism is an essential and complimentary learning theory all too often vilified and therefore not incorporated into pedagogical practices. However, behaviorism is the crux of informal assessment and can be used effectively to bring about powerful social changes without punishments. Behaviorism is the use of external environmental factors to bring about changes in an individual. Most often, behaviorism is activated by using rubrics, either formatively or summatively, to guide individuals toward clearly articulated and agreed upon goals. Design of Learning Environments The following principles impact the design of STEM PBL activities: starting with the end product in mind making content accessible across the learner spectrum, which includes formal, informal, face-to-face, and distance learning making thinking visible, which includes using visual elements to help the learner and using learner-constructed visual elements to assess learning helping students learn from others promoting autonomy and lifelong learning 34 Designing a STEM Project-Based Lesson Although these five lesson design principles are presented separately for discussion purposes, they are integrated in practice. Starting With the End in Mind Knowing what you want the learner to do, create, build, or accomplish is the first step in building an outstanding academic learning activity. For example, to address the Common Core State Standard (CCSS) dealing with irrational numbers (see Figure 1), starting with the end in mind necessitates that one think about how students would demonstrate their knowledge. For this CCSS objective, one might lead an activity requiring students to collect diameter and circumference measurements from objects (i.e., paper plates, canned goods, lids, buckets, etc.) with the following end in mind: Students will create a table that clearly labels rational and irrational numbers and include a three-minute presentation, video, or audio explanation of what they learned and explain how to differentiate between rational, irrational, and repeating decimal numbers. Although the proposed activity does not specify teaching students about repeating decimal numbers as required by the CCSS objective or the end in mind, this should part of the larger lesson and purposefully integrated into the activity where ratios are converted. Figure 1. CCSS for Learning Irrational Numbers CCSS.MATH.CONTENT.8.NS.A.1 Know that numbers that are not rational are called irrational. Understand informally that every number has a decimal expansion; for rational numbers show that the decimal expansion repeats eventually, and convert a decimal expansion which repeats eventually into a rational number. Design Principle — Making Content Accessible Content is made accessible by allowing learners to engage in problems, examples, and contexts that connect new ideas to personally relevant prior knowledge and is grounded in three pragmatic pedagogical dimensions: building on student ideas, use of personally relevant problems, and scaffolding inquiry (Lynn et al., 2004). Thus, effective instruction should provide opportunities for students to ask their own questions, refine those questions through the design and conducting of personally relevant investigations, evaluate data and scientific evidence according to their own personal understanding, verbalize their own theories and explanations, and participate in active science learning. Scaffolding and feedback are essential supports for inquiry. Scaffolding allows the learner to refine their thinking (Krajcik et al., 1998), which allows them to more deeply participate in the inquiry process. Examples of scaffolds include modeling, coaching, sequencing, interacting with more knowledgeable people, reducing or gradually building complexity, highlighting critical features, modeling/prompting, and using visual tools (Krajcik et al., 1999; van de Pol et al., 2010). Timely feedback is essential Designing a STEM Project-Based Lesson 35 to helping students analyze their own reasoning, making them less dependent on the teacher to diagnose their problems. Together, these design elements are fundamental to making content accessible. Design Principle — Making Thinking Visible Making thinking visible is grounded in how ideas are connected (Bransford et al., 2000) and includes three pragmatic pedagogical dimensions: modeling scientific thinking, scaffolding students to make their thinking visible, and providing multiple representations (Lynn et al., 2004). Science is often taught as a body of knowledge with little understanding of the true nature of science. Students are frequently frustrated when their designs are unsuccessful. Scaffolding students to make their thinking visible provides opportunities for students to explicitly monitor their own learning, which encourages reflection and more accurately models the scientific process (Bell, 2010; Bryan & Slough, 2009). Providing multiple representations is essential to allow students to actively participate in the interpretive process of science (Lynn et al., 2004). Computer animations, modeling programs, dynamic representations, and scientific visualizations represent the cutting edge of science and make abstract concepts more accessible to the learner. Furthermore, recall of one type of representation can support recall of another type of representation of the same material (Baddley & Longman, 1978; Brunner, 1994). Consequently, making thinking visible makes scientific thinking visible to the learner and thus more accessible. Moreover, making thinking visible also makes student thinking visible, and this affords opportunities for students to actively build metacognitive skills, facilitates more effective scaffolds and feedback from the teacher, and makes use of multiple representations, thereby facilitating student interaction between the two worlds of science and learning. Design Principle — Helping Students Learn From Others Helping students learn from others is grounded in social constructivism (Vygotsky, 1978), cooperative learning (Johnson et al., 2000), and communities of learners (Fong & Slotta, 2018) and includes four pragmatic pedagogical dimensions: encouraging listening to others, design discussions, highlighting cultural norms, and employing multiple social structures (Lynn et al., 2004). Students must be trained to listen to others and to think before responding or acting. Reciprocal teaching (Mulyono et al., 2018) emphasizes communities of learners observing and learning from role models. Design is often a central component to PBL lessons. When students design, they must discuss. In a design discussion, students must have time to “reflect, incorporate the ideas of others, and compose their contributions carefully rather than formulating imperfect arguments” (Lynn et al., 2004, p. 62). It is especially important that these design discussions overtly establish the cultural norms of science, which requires the inclusion of all ideas—including ideas that are ultimately rejected, justification for ideas/designs/rejections, and attribution to experts or evidence. Students must be allowed to 36 Designing a STEM Project-Based Lesson establish criteria for scientific explanations, to evaluate their own progress, to analyze the progress of others, to describe the connections between their ideas and those of others, and to critique connections proposed by others. These processes are facilitated by the creation of social interactions and norms that enable learners to hear ideas in the words of peers, experts, and members of diverse cultural groups. This principle is important to creating engaging and inclusive STEM PBL activities. Design Principle — Promoting Autonomy and Lifelong Learning Promoting autonomy and lifelong learning is grounded in metacognition and inquiry and includes four pragmatic pedagogical principles: encouraging monitoring, providing complex projects, revisiting and generalizing inquiry processes, and scaffolding critique (Lynn et al., 2004). One misconception about student-centered instruction is that teachers do nothing when in fact teachers in student-centered instruction are more active than in most teacher- centered, didactic, presentation-style instruction. Too little or too much monitoring and feedback deters student learning (Anderson, 1982), while “optimal instruction balances feedback with opportunities for students to evaluate their own ideas” (p. 66). Complex projects lend themselves specifically to complex learning and generally to the inquiry process. Through these processes, students devise personal goals, seek feedback from others, interpret comments, and adjust behavior accordingly. Students must be encouraged to organize ideas, construct arguments, add new evidence, and revisit phenomena in new contexts. Teachers are encouraged to design ways to scaffold students as they devise new explanations and arguments in the context of inquiry. By incorporating the aforementioned processes and design features, teachers can foster learner autonomy and lifelong learning. Summarizing Foundations for Learning and Design Principles Changes in conceptual understanding(s) occur as teachers engage and problematize students’ preexisting knowledge. Inquiry and PBL allows the teacher an opportunity to engage the prior knowledge, skills, concepts, and beliefs students bring with them to the learning environment. In order for thinking to become visible and therefore shaped, students must be given the opportunity to expose their own thinking through feedback, revision, and reflection with themselves, teachers, and other students. Inquiry and PBL can be structured in such a way as to provide students with these opportunities. Inquiry and PBL also promote teaching for understanding by allowing teachers to make available many examples of the same concept at work in different conditions. Metacognition, the awareness of and reflection upon one’s own thinking, is a skill that allows people to distinguish when they comprehend and when they need more information. Inquiry and PBL may afford students the opportunity to take control of their own learning by situating learning goals and monitoring their progress—both academically and cognitively. Designing a STEM Project-Based Lesson 37 Changes in conceptual understanding(s) are facilitated by overt design decisions that build on the foundations for learning. Making content accessible is facilitated by building on preexisting knowledge and student discourse, and it scaffolds feedback by allowing learners to engage in problems, examples, and contexts that connect new ideas that are personally relevant. Together, using visual elements in instruction and encouraging student construction of visual elements promote making thinking visual. As students learn from others, they have the opportunity to learn the cultural norms of science—including the notion that ideas are accepted or rejected based on evidence. Promoting autonomy and lifelong learning occurs as students learn to devise personal goals, seek feedback from others, interpret comments, adjust behavior accordingly, and evaluate their own ideas. Project-Based Learning Curriculum Traditional Hands-On Lab (Verification of Facts) The emphasis in the traditional hands-on lab is on the verification of facts already presented to the learner. The teacher controls the assessment, topic, task, resources, procedure/design, artifacts/analysis, and often even the outcomes. This type of experience is often dominated by worksheets and fill-in-the blank forms. Novice (Factual Knowledge) The differences between traditional hands-on and novice are subtle. Instead of verifying factual knowledge previously learned, the student is generating factual knowledge that is novel to them. Although the lab and its components have been determined by the teacher, this constructivist approach allows the learner to analyze the data and determine the outcomes. It is important to note that at this novice level, the outcomes and determinations by the student are only factual in nature. For example, if I drop a ball, it falls to the ground. At the traditional hands-on level, this lab would verify previous teachings that when a ball is dropped, it falls to the ground. At this novice level, it is the student who constructs the factual understanding. Informed Novice (Understand Facts/Ideas in Context of Conceptual Framework) At the informed novice level, chunks of factual knowledge are connected to build a conceptual understanding. Students rationalize the relationships and connections between multiple pieces of knowledge. In the previous example, students determined that when they drop a ball, it falls to the ground. Perhaps in another lab, students also learned Newton’s Law of Gravity. At the informed novice level, the purpose of the lab is to connect these two pieces of factual knowledge to form a conceptual understanding. If I drop a ball, it falls to the ground. Newton’s Law of Gravity states that objects with larger mass attract objects of a smaller mass. Therefore, 38 Designing a STEM Project-Based Lesson the ball drops to the ground because it has a smaller mass than the ground (Earth). Students analyze relationships between facts to develop more complex conceptual understandings. At this level, the idea of community becomes vital. Students must be given opportunities for discourse with each other, with experts, and with the teacher. These include dialogues about ideas and naïve theories, to determine what information is valid and reliable, and to decide how factual information is connected to form a conceptual understanding, all of which should be community centered. The community of learners ultimately decides which naïve theories become appropriate knowledge and understanding. The importance of community continues to deepen as the levels of complexity increase. Expert (Adapts Conceptual Frameworks Through Transfer) In general, experts are capable of applying their knowledge, their expertise, to new or novel situations. The ability to transfer knowledge into new situations successfully is a crucial assessment component when teaching for understanding. At the expert level, the goal is for the student to be able to transfer his or her understanding(s) of the material to novel situations. There is usually more than one method for solving problems. The student and/or the community must be given more freedom of choice when determining 1) how to approach the problem, 2) what acceptable resources to use, 3) how the data is analyzed, and 4) how the results are interpreted. The teacher and the student must both have experience and success operating with fewer constraints. Therefore, the expert level not only requires deep factual knowledge and a solid conceptual framework but also the ability to work more independently than in the past. Researcher (Creation of New Knowledge and/or Conceptual Frameworks) At the researcher level, the learner is in control of his or her learning. Students are capable of choosing the topic of interest and are well equipped to make learning happen. This level requires many years of practice, and the learner must be scaffolded at each step. Reaching the researcher level is analogous to obtaining a terminal degree—you have been given the tools to learn independently. This should be the goal of education regardless of subject matter. One cannot expect a student or teacher to effectively operate at this level without proper training and experience. To expect either to move from any previous level to the researcher level without this training and experience is irresponsible—movement must be slow and thoughtful. Time Time is often the forgotten dimension in today’s fast-paced environment, but research has shown that it takes three to five years for meaningful changes in curriculum and instructional practices to occur following a professional development experience (Guskey, 2002). This time Designing a STEM Project-Based Lesson 39 (and the time following the experience) must be spent consistently advocating for and pursuing significant change in teacher, student, and community behavior. In short, significant change in teacher, student, and community behavior takes more than resources; it takes time. This has implications on effective implementation of PBL strategies. If a teacher enters a professional development seminar at the most teacher-centered level of PBL, this educator should not be expected to operate at the more sophisticated student-centered levels of PBL immediately. Students, from kindergarten to post-secondary levels enter the learning environment at various levels of sophistication and experience with PBL, resulting in an “unresolved tension between the practical doing and the content learning” (Kanter, 2009, p. 527). They too should not be expected to work completely outside of their comfort levels. Growth towards a more sophisticated level of PBL should be incremental and within the appropriate zone of proximal development (Vygotsky, 1978) of all participants—teachers, students, and the community. If teachers and students operate beyond their zone of proximal development, failure is likely. Mistakenly, this failure may be blamed upon the PBL lesson itself or on the inability of teachers and students to work within the PBL framework. In actuality, success or failure depends as much on understanding levels of PBL and working within the appropriate zone of proximal development as it does on the teacher’s actual ability and knowledge to implement this new technique. Components of PBL We use a checklist (i.e., the Teacher Project-Based Learning Checklist; see following page & Appendix X) to determine if teachers have included all the necessary components in their PBL lessons. Examples of STEM PBL lessons can be found in our Companion Book (Capraro et al., 2016). We explain each of the categories within the checklist below and provide an example of how they might be filled out when developing a STEM PBL lesson about constructing a tiny house. 40 Designing a STEM Project-Based Lesson Teacher Project-Based Learning Checklist Yes No PBL Headings 1) Project Title: 2) Teacher Names: 3) Grade Level: 4) PBL Dates: 5) Teacher Introduction: 6) Objectives: Does this section include… Yes No Rigor Interdisciplinary Link 7) Connections (How does this PBL connect to other units in your subject?): 8) Introduction: Does this section include… Yes No Relevance 9) Well-defined Outcome: Rigor 10) Materials used: 11) Engagement: Does your plan address… Yes No 1.Problem Identification 2. Ideation Grouping Questioning (Indicate the number) Open-ended… Large Probing… Small Guiding… 12) Exploration: Does your plan address Yes No. 1. Problem Identification 2. Research 3. Ideation 4. Analysis of Ideas 5. Testing and Refinement 1. Communication and Metacognition 13) Explanation (Explain the subject Does your plan address matter knowledge (theory) and other issues 1. Problem Identification you’d like to make clear): 2. Research 3. Ideation 4. Analysis of Ideas 5. Testing and Refinement 6. Communication and Metacognition 14) Extension (How can you extend this PBL for students who are more able and/or those who have finished before the others?): 15) Evaluation (Includes the formative and summative rubric, as well as multiple choice): 16) References (websites); Guest Speaker information; Ordering information: Designing a STEM Project-Based Lesson 41 1) Project Title: Your tile should capture the essence of the content of the PBL. 2) Teacher: Place your name here. 3) Grade Level: Place your grade level here and also include any other grade level that it may be appropriate for. 4) Schedule at a Glance: This is a quick overview of each day’s activities. Generally, it is placed in a table (i.e., the one below outlining the 14 days of building a tiny house: Schedule at a Glance Day 1 Day 2–3 Day 4–5 Day 6–8 Day 9–12 Day 13–14 Read article, Explanation of Research on Begin Begin working Give show video, surface area. tiny house working on on three- presentations. and discuss elements and two- dimensional tiny houses. environmentally dimensional designs and friendly designs. presentation. elements. 5) Well-Defined Outcome (WDO) and Ill-Defined Task (IDT): This is what students will know and be able to do by the end of the project. The WDO (underlined in the example below) provides sufficient information to know what the finished product is. It includes the deliverable, constraints, and expected learning outcomes. The IDT contains basic information. The IDT (italicized below) provides sufficient information to know how the content (WDO) comes together but way too little information to ensure that every attempt would look exactly the same and or the process for getting to the WDO would be exactly the same. It is important here that there is too little specificity so that students can bring their own backgrounds and learning rates, resulting in final deliverables that are distinct, show group and individual growth, and foster students as they learn at their own rates and depth while mastering the specified outcome. Example: Each student will use geometric constructions and assembly techniques that will lead to creating the tallest self-standing structure and authentic measurement strategies to evaluate your and your peers’ constructions choosing 6 of the 7 materials provided within 6½ minutes. To complete the project, your structure must be transported to the measuring stand and be free standing at the time it is set. Once the structure is placed on the judging stand, it will be judged based on the greatest vertical perpendicular distance from its first contact with the judging stand to its tallest vertical point. To qualify for judging, each team must provide their design notebooks, sketches, assembly instructions, a construction narrative (700 words max), materials list, and materials budget and expense report. 42 Designing a STEM Project-Based Lesson 6) Teacher Introduction: This is the narrative for you to help familiarize stakeholders with the task at hand. It is the big idea and background. It is the sales pitch to other teachers whose assistance you might need to be successful with the project. It will be the catchy pitch to get your principal or department chair to provide the necessary materials to accomplish the task or the budget for you to acquire the materials. It is also the place for you to describe your classroom environment: how many students are there, how long are your class periods, how often will students work on the project, what are students’ prior knowledge, or what skills do they lack in general that you might need to focus on for them to be successful? This is not for you—you know your classroom. In addition to this being your sales pitch, it is for others, some of whom may want to implement or adapt your idea. Think about important background information for the substitute teacher who you want to help carry out your idea in your absence. 7) Objectives: It is very important that teachers cover the state and/or district standards for their grade level. Generally, you are not implementing a project because you think it will be “cool” or “fun.” You as the teacher must plan with the end in mind. Ask yourself the following: What skills and objectives do I need to cover with this project? What objectives have the students worked on and you feel they have not yet mastered? Which skills, if taught through a different approach, would help students master the concepts and enable them to truly own their learning? When writing your PBL plan, you can list national standards (i.e., National Council of Teachers of Mathematics standards, Next Generation Science Standards, Common Core State Standards, etc.) or your own state or district standards (i.e., Texas Essential Knowledge and Skills), whatever your school principal expects. 8) STEM Connections: Projects, just like real-world situations, should be interdisciplinary (see Chapters 13 & 15). When designing a STEM PBL lesson, you should create PBL connections to objectives in other STEM areas. Consult your district, state, or national objectives or other teachers in your school. An example is shown below of how the tiny house project connects STEM objectives within a real-world project. Do not expect to address objectives in every aspect of the STEM discipline, but be sure to capture the big ones for your school, district, or program. Science: Identify environmentally friendly materials and determine impacts on an ecosystem’s stability. Technology: Use digital applications to create 2D and 3D designs. Engineering: Develop a plan using various layouts and materials to obtain a sense of creating a design of a structure. Mathematics: Calculate perimeter, area, and surface area of various shapes. Designing a STEM Project-Based Lesson 43 9) Student Introduction: In this section, you are introducing the project to the students. This is generally part of the engagement time. This is your sales pitch to your students. It should contain how the project is situated within the real world, the issues that need to be solved, the well-defined outcome and the ill-defined task, and the time and material constraints. Example: The earth has been around for a long time and has gone through many changes. One thing we know is that the earth’s resources are not infinite. We are at a time where the resources we use could run out on us. We must be aware that we could run out of water, natural gases, oil, and wooded areas. The housing market is at a boom, and the average size of a single-family house has gone up in square footage. Rethinking how we use these natural resources and designing more environmentally friendly houses is a step in the right direction to help slow down our declining resources. In this PBL activity, you will have the opportunity to become an architect and design your own tiny house. You can be as creative and inventive as you want, but remember that you need to stay within 100 to 400 square feet and that the tiny house must fit a full-size refrigerator and have a washer and dryer unit as well as sleeping, eating, and bathroom area(s). 10) Materials: List all the materials you will need for the students to engage in the project. Be as specific as possible. Many students like loopholes, and advanced STEM PBL activities might list things that cannot be used in order to challenge students to be resourceful in locating and determining the materials they can use. For example, students may not be allowed to use glue, glue sticks, paper clips, or staples in their construction. Otherwise, if you want to ensure a more even playing field for your students, you can provide the materials and set of budgeting costs that students must account for when picking up materials. For example, every six inches of string or part thereof is $5.00. You would then develop pricing for each material and explain that the best construction that costs the least would be the most cost-efficient submission. 11) Engagement: In this section, you are describing how you are going to tap into students’ interests in your particular project. For the tiny house project, you could watch a clip from an episode of Tiny House on HGTV, for a bridge building project show the collapse of the Takoma Narrows Bridge, during a recycling project have students walk back into the classroom after lunch where all the trash has been collected in a large pile or show pictures of the Pacific Garbage Patch, and in a water pollution STEM PBL perhaps show a video of the dangers of water pollution. Another approach for engaging students is to have them fill out a KWL sheet on the topic: (K) What do I know about ____, (W) What do I want to learn about ____, and (L) What I learned about ____. Regardless of the approach, it must match the grade level and composition 44 Designing a STEM Project-Based Lesson of the students described earlier. For example, you would not watch a video on the collapse of the Takoma Narrows Bridge with students in the 5th grade who had limited understanding of Bernoulli’s Principle and waves but instead a video that shows types of bridges, such as suspension, pontoon, cantilever, truss, beam, arch, and so on. 12) Explanation: In this section, you describe what the teacher needs to explain to his/her students about a particular topic within the project. This is the place where you list all those small tidbits of knowledge you want to make sure you introduce and that will help you differentiate your instruction for those students across the learning spectrum in your class. It is usually presented to a whole group as a total lesson. You might already know a concept that students do not possess. Or perhaps as you are circulating around the classroom and realize that three of the five groups all do not understand something, so you need to stop and explain to either the three groups or the entire class. Some possible topics include how to calculate surface area, the steps in using a graphing calculator, how to design a graph, how to interpret data, how to structure a commercial, or how write a press release. 13) Exploration: In this section, you discuss how students are going to self-direct their learning about the project (potentially while using pre-screened books, newspapers, videos, internet websites, or other sources). After students’ interests are piqued from the engagement phase, the teacher needs to provide opportunities for students to explore the new topic more deeply to understand both the problem and possible solution strategies. This is an important step before their brainstorming about what each group will actually do to address the deliverable. During this exploration phase, students begin to think more deeply about the topic, ask more questions, and begin to build a list of possible solution strategies. They start to ask questions and explore their understanding, and the teacher’s role is clear. Do not answer questions unless the question deals with a part of the project already addressed in the project brief. In this case, tell them where to find the information in the project brief or materials that have been provided. Encourage their questions, and use questions that can direct them to finding the answers for themselves. Here is an example of an exchange that encourages students to direct their own investigation: Student: “Teacher, what construction structures will be strongest against torque?” Teacher: “Great question. Have you tried using a Google Search? What search terms could you use?” Designing a STEM Project-Based Lesson 45 14) Extension: In this section, you describe activities that students can engage in after the project is complete. These activities can be directly related to the project or closely focused on one of the other areas of STEM and tangentially related to the project. In this section, the teacher designs activities that involve higher level thinking skills. These activities can assist in developing student thinking beyond what they did in the project phase, allowing them to gain even deeper conclusions. This is especially important if your groups perform at very different levels. This section can help you to create a high-quality differentiated learning experience that allows students who learn faster to take the lesson farther. 15) Evaluation: Refer to Chapter 7, which discusses assessment strategies, for more details for this section. Assessment must be planned and explicit and available for the learner at the beginning of the lesson. These assessments should govern both group and individual responsibility. In STEM PBL, the group is responsible but the individuals are accountable. Therefore, various formative assessments should be included, such as checklists and exit tickets, to name but a few common ones that can readily be used across multiple projects. You should also make use of summative assessments that provide graded feedback at critical stages. One at the end of the design stage where the design notebooks are graded, one at the end of the next stage, one for the presentation, and one for the quality of the deliverable are all reasonable points for summative assessments. Occasionally during the project, it will be necessary to develop new checklists, rubrics, and multiple-choice questions to guide learning and foster a deeper sense of both group responsibility and individual accountability. 16) References and Resources: Include in this section any websites you may need to use either personally or for students. It is important to review these sites prior to listing them to make sure they are educationally appropriate and align with your learning goals. It is also a place to include links to professional organizations, videos, content lessons (think Khan Academy, Udemy, or Yale Open Courses) along with information about guest speakers or ordering information for yourself as you progress through the development and delivery of the project. 46 Designing a STEM Project-Based Lesson Conclusion STEM PBL encourages deep and thoughtful learning on the part of the student. Such learning cannot occur without the careful and balanced guidance of the teacher, however. The designing of a STEM PBL lesson thus requires careful thought to the purpose and goals of the lesson as well as to the environment in which the learning is to take place. The use of the Teacher PBL Checklist provides teachers with an easy way to organize the many aspects of a STEM PBL lesson when designing one so that students can successfully engage in inquiry-based learning supported by a prepared and knowledgeable instructor. Reflection Questions and Activities 1. What theories of learning inform STEM PBL instruction, and what two main ones encompass the others? 2. What STEM PBL design principle do you think would most easily be addressed in your classroom? Which would be the most challenging? 3. Think about your students. What level of learning do you think they are currently at? How do you think the STEM PBL example give in this chapter might need to be changed to fit their level of learning? 4. Consider a district, state, or national learning standard that your students may struggle with. Beginning with the end in mind, develop a STEM PBL lesson that would engage students in actively building their own knowledge and learning as you guide them to meet this standard of learning. References Anderson, J. R. (1982). Acquisition of cognitive skill. Psychological Review, 89, 369– 406. https://doi.org/10.1037/0033-295X.89.4.369 Baddley, A. D., & Longman, D. J. (1978). The influence of length and frequency of training session on the rate of learning to type. Ergonomics, 21, 627–635. https://doi.org/10.1080/00140137808931764 Bransford, J. D., Brown, A. L., & Cocking, R. R. (2000). How people learn: Brain, mind, experience, and school. The National Academies Press. Bell, S. (2010). Project-based learning for the 21st century: Skills for the future. The Clearing House, 83, 39–43. https://doi.org/10.1080/00098650903505415 Brunner, D. D. (1994). Inquiry and reflection: Framing narrative practice in education. SUNY Press. Bryan, J., & Slough, S. W. (2009). Converging lens simulation design and image predictions. Physics Education, 44, 264–275. Capraro, M. M., Whitfield, J. G., Etchells, M. J., & Capraro, R. M. (Eds.). (2016). A companion to interdisciplinary STEM project-based learning: For educators by educators (2nd ed.). Sense. Designing a STEM Project-Based Lesson 47 Fong, C., & Slotta, J. D. (2018). Supporting communities of learners in the elementary classroom: The common knowledge learning environment. Instructional Science, 46, 533–561. https://doi.org/10.1007/s11251-018-9463-3 Guskey, T. R. (2002) Professional development and teacher change. Teachers and Teaching: Theory and Practice, 8(3), 381–391. https://doi.org/10.1080/135406002100000512 Johnson, D. W., Johnson, R. T., & Stanne, M. E. (2000). Cooperative learning methods: A meta-analysis. Cooperative Learning Center. Jumaat, N. F., Tasir, Z., Halim, N. D. A., & Ashari, Z. M. (2017). Project-based learning from constructivism point of view. Advanced Science Letters, 23(8), 7904–7906. https://doi.org/10.1166/asl.2017.9605 Kanter, D. E. (2009). Doing project and learning the content: Designing project-based science curricula for meaningful understanding. Science Education, 94, 525–551. Kokotsaki, D., Menzies, V., & Wiggins, A. (2016). Project-based learning: A review of the literature. Improving schools, 19(3), 267–277. https://doi.org/10.1177/1365480216659733 Krajcik, J. S., Blumenfeld, P. C., Marx, R. W., Bass, K. M., Fredricks, J., & Soloway, E. (1998). Inquiry in project-based science classrooms: Initial attempts by middle school students. The Journal of the Learning Sciences, 7, 313–350. https://doi.org/10.1080/10508406.1998.9672057 Krajcik, J. S., Czerniak, M. C., & Berger, C. (1999). Teaching children science: A project-based approach. McGraw-Hill. Lynn, M. C., Davis, E. A., & Eylon, B. S. (2004). The scaffolded knowledge integration framework for instruction. In M. C. Lynn, E. A. Davis, & P. B. Bell (Eds.), Internet environments for science education (pp. 47–72). Erlbaum. Mulyono, D., Asmawi, M., & Nuriah, T. (2018). The effect of reciprocal teaching, student facilitator and explaining and learning independence on mathematical learning results by controlling the initial ability of students. International Electronic Journal of Mathematics Education, 13(3), 199–205. https://doi.org/10.12973/iejme/3838 van de Pol, J., Volman, M. & Beishuizen, J. (2010). Scaffolding in teacher–student interaction: A decade of research. Educational Psychology Review, 22, 271–296. https://doi.org/10.1007/s10648-010- 9127-6 Vygotsky, L.S. (1978). Mind in society: The development of higher psychological processes. Harvard University Press. 48 Designing a STEM Project-Based Lesson