Chapter-Two Electromagnetic Energy and Remote Sensing PDF
Document Details
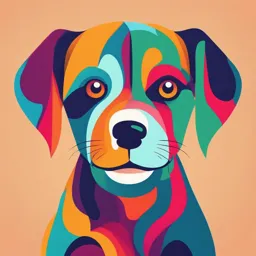
Uploaded by ArdentArcticTundra6277
Tags
Summary
This document discusses electromagnetic energy and remote sensing, including properties of electromagnetic radiation such as wavelength and frequency. This document also looks at how electromagnetic radiations are impacted by the atmosphere and reflected or emitted from various surfaces like the Earth's surface.
Full Transcript
Chapter-Two Electromagnetic energy and remote sensing Electromagnetic Radiation The first requirement for remote sensing is to have an energy source to illuminate the target (unless the sensed energy is being emitted by the target). This energy is in the form of electrom...
Chapter-Two Electromagnetic energy and remote sensing Electromagnetic Radiation The first requirement for remote sensing is to have an energy source to illuminate the target (unless the sensed energy is being emitted by the target). This energy is in the form of electromagnetic radiation. All electromagnetic radiation has fundamental properties and behaves in predictable ways according to the basics of wave theory. ER consists of an electrical field (E) which varies in magnitude in a direction perpendicular to the direction in which the radiation is traveling, and a magnetic field (M) oriented at right angles to the electrical field. Both these fields travel at the speed of light (c). Two characteristics of electromagnetic radiation are particularly important for understanding remote sensing. ❖ These are: 1. Wavelength 2. Frequency Wave Model of EMR o Therefore, the two are inversely related to each other. The shorter the wavelength, the higher the frequency. o The longer the wavelength, the lower the frequency. o Understanding the characteristics of electromagnetic radiation in terms of their wavelength and frequency is crucial to understanding the information to be extracted from remote sensing data. o Wavelength and frequency are related by the following formula: c=λv where Wave Model of EMR Electromagnetic energy All matter with a certain temperature radiates electromagnetic waves of various wavelengths. The total range of wavelengths is commonly referred to as the electromagnetic spectrum. Remote sensing operates in several regions of the electromagnetic spectrum. The electromagnetic spectrum ranges from the shorter wavelengths (including gamma and x-rays) to the longer wavelengths (including microwaves and broadcast radio waves). There are several regions of the electromagnetic spectrum which are useful for remote sensing. Ultraviolet (UV) The ultraviolet (UV) portion of the spectrum has the shortest wavelengths that are of practical use for remote sensing. This radiation is beyond the violet portion of the visible wavelengths. Some of the Earth’s surface materials, primary rocks and minerals, emit or fluoresce visible light when illuminated with UV radiation. Visible The light which our eyes - our "remote sensors" - can detect is part of the visible spectrum. It is important to recognize how small the visible portion is relative to the rest of the spectrum. There is a lot of radiation around us which is "invisible" to our eyes, but can be detected by other remote sensing instruments and used to our advantage. The visible wavelengths cover a range from approximately 0.4 to 0.7 μm. The longest visible wavelength is red and the shortest is violet. Common wavelengths of what we perceive as particular colours from the visible portion of the spectrum are listed below. It is important to note that this is the only portion of the spectrum we can associate with the concept of colours. Violet: 0.4 - 0.446 μm Blue: 0.446 - 0.500 μm Green: 0.500 - 0.578 μm Yellow: 0.578 - 0.592 μm Orange: 0.592 - 0.620 μm Red: 0.620 - 0.7 μm ✓ Blue, green, and red are the primary colours or wavelengths of the visible spectrum. Infrared (IR) The next portion of the spectrum of interest is the infrared (IR) region which covers the wavelength range from approximately 0.7 μm to 100 μm - more than 100 times as wide as the visible portion! The infrared region can be divided into two categories based on their radiation properties - the reflected IR, and the emitted or thermal IR. Radiation in the reflected IR region is used for remote sensing purposes in ways very similar to radiation in the visible portion. The reflected IR covers wavelengths from approximately 0.7 μm to 3.0 μm. The thermal IR region is quite different than the visible and reflected IR portions, as this energy is essentially the radiation that is emitted from the Earth’s surface in the form of heat. The thermal IR covers wavelengths from approximately 3.0 μm to 100 μm. Microwave region The portion of the spectrum of more recent interest to remote sensing is the microwave region from about 1 mm to 1 m. This covers the longest wavelengths used for remote sensing. The shorter wavelengths have properties similar to the thermal infrared region while the longer wavelengths approach the wavelengths used for radio broadcasts. Principal division of EM spectrum Energy interaction in the atmosphere Before radiation used for remote sensing reaches the Earth's surface it has to travel through some distance of the Earth's atmosphere. Particles and gases in the atmosphere can affect the incoming light and radiation. These effects are caused by the mechanisms of scattering and absorption. Scattering occurs when particles or large gas molecules present in the atmosphere interact with and cause the electromagnetic radiation to be redirected from its original path. How much scattering takes place depends on several factors including the wavelength of the radiation, the abundance of particles or gases, and the distance the radiation travels through the atmosphere. There are three (3) types of scattering which take place. 1. Rayleigh scattering occurs when particles are very small compared to the wavelength of the radiation. These could be particles such as small specks of dust or nitrogen and oxygen molecules. Rayleigh scattering causes shorter wavelengths of energy to be scattered much more than longer wavelengths. Rayleigh scattering is the dominant scattering mechanism in the upper atmosphere. The fact that the sky appears "blue" during the day is because of this phenomenon. As sunlight passes through the atmosphere, the shorter wavelengths (i.e. blue) of the visible spectrum are scattered more than the other (longer) visible wavelengths. At sunrise and sunset the light has to travel farther through the atmosphere than at midday and the scattering of the shorter wavelengths is more complete; this leaves a greater proportion of the longer wavelengths to penetrate the atmosphere. 2. Mie scattering occurs when the particles are just about the same size as the wavelength of the radiation. Dust, pollen, smoke and water vapour are common causes of Mie scattering which tends to affect longer wavelengths than those affected by Rayleigh scattering. Mie scattering occurs mostly in the lower portions of the atmosphere where larger particles are more abundant, and dominates when cloud conditions are overcast. 3. Nonselective scattering occurs when the particles are much larger than the wavelength of the radiation. Water droplets and large dust particles can cause this type of scattering. Nonselective scattering gets its name from the fact that all wavelengths are scattered about equally. This type of scattering causes fog and clouds to appear white to our eyes because blue, green, and red light are all scattered in approximately equal quantities (blue + green + red light = white light). Absorption is the other main mechanism at work electromagnetic radiation interacts with the atmosphere. ❑ In contrast to scattering, this phenomenon causes molecules in the atmosphere to absorb energy at various wavelengths. ❑ Ozone, carbon dioxide, and water vapour are the three main atmospheric constituents which absorb radiation. ❑ Ozone serves to absorb the harmful (to most living things) ultraviolet radiation from the sun. ❑ Without this protective layer in the atmosphere our skin would burn when exposed to sunlight. ❑ Carbon dioxide referred to as a greenhouse gas. ❑ This is because it tends to absorb radiation strongly in the far infrared portion of the spectrum - that area associated with thermal heating - which serves to trap this heat inside the atmosphere. ❑ Water vapour in the atmosphere absorbs much of the incoming longwave infrared and shortwave microwave radiation (between 22μm and 1m). ❑ Those areas of the spectrum which are not severely influenced by atmospheric absorption and thus, are useful to remote sensors, are called atmospheric windows. Energy interaction with the earth’s surface Radiation that is not absorbed or scattered in the atmosphere can reach and interact with the Earth's surface. There are three (3) forms of interaction that can take place when energy strikes, or is incident (I) upon the surface. These are: absorption (A); transmission (T); and reflection (R). The total incident energy will interact with the surface in one or more of these three ways. The proportions of each will depend on the wavelength of the energy and the material and condition of the feature. E(I) = E(A) + E(R) + E(T) Absorption (A) occurs when radiation (energy) is absorbed into the target while transmission (T) occurs when radiation passes through a target. Reflection (R) occurs when radiation "bounces" off the target and is redirected. In remote sensing, we are most interested in measuring the radiation reflected from targets. We refer to two types of reflection, which represent the two extreme ends of the way in which energy is reflected from a target: specular reflection and diffuse reflection. When a surface is smooth we get specular or mirror-like reflection where all (or almost all) of the energy is directed away from the surface in a single direction. Diffuse reflection occurs when the surface is rough and the energy is reflected almost uniformly in all directions. a) specular reflection b) diffuse reflection. Most earth surface features lie somewhere between perfectly specular or perfectly diffuse reflectors. Leaves: A chemical compound in leaves called chlorophyll strongly absorbs radiation in the red and blue wavelengths but reflects green wavelengths. Leaves appear "greenest“ to us in the summer, when chlorophyll content is at its maximum. In autumn, there is less chlorophyll in the leaves, so there is less absorption and proportionately more reflection of the red wavelengths, making the leaves appear red or yellow (yellow is a combination of red and green wavelengths). The internal structure of healthy leaves act as excellent diffuse reflectors of near-infrared wavelengths. If our eyes were sensitive to near- infrared, trees would appear extremely bright to us at these wavelengths. In fact, measuring and monitoring the near-IR reflectance is one way that scientists can determine how healthy (or unhealthy) vegetation may be. Water: Longer wavelength visible and near infrared radiation is absorbed more by water than shorter visible wavelengths. Thus water typically looks blue or blue-green due to stronger reflectance at these shorter wavelengths, and darker if viewed at red or near infrared wavelengths. If there is suspended sediment present in the upper layers of the water body, then this will allow better reflectivity and a brighter appearance of the water. The apparent colour of the water will show a slight shift to longer wavelengths. Suspended sediment (S) can be easily confused with shallow (but clear) water, since these two phenomena appear very similar. Chlorophyll in algae absorbs more of the blue wavelengths and reflects the green, making the water appear more green in colour when algae is present. Spectral reflectance curves Consider a surface composed of a certain material. The energy reaching this surface is called irradiance. The energy reflected by the surface is called radiance. Irradiance and radiance are expressed in W m-2. For each material, a specific reflectance curve can be established. Such curves show the fraction of the incident radiation that is reflected as a function of wavelength. From such curve you can find the degree of reflection for each wavelength. Most remote sensing sensors are sensitive to larger wavelength bands, for example from 400–480 nm, and the curve can be used to estimate the overall reflectance in such bands. Reflectance curves are made for the optical part of the electromagnetic spectrum (up to 2.5 µm). Today, large efforts are made to store collections of typical curves in spectral libraries. Reflectance measurements can be carried out in a laboratory or in the field using a field spectrometer. In the following subsections the reflectance characteristics of some common land cover types are discussed. Vegetation The reflectance characteristics of vegetation depend on the properties of the leaves including the orientation and the structure of the leaf canopy. The proportion of the radiation reflected in the different parts of the spectrum depends on leaf pigmentation, leaf thickness and composition (cell structure) and on the amount of water in the leaf tissue. Figure below shows an ideal reflectance curve from healthy vegetation. In the visible portion of the spectrum, the reflection from the blue and red light is comparatively low since these portions are absorbed by the plant (mainly by chlorophyll) for photosynthesis and the vegetation reflects relatively more green light. The reflectance in the near infrared is highest but the amount depends on the leaf development and the cell structure of the leaves. In the middle infrared, the reflectance is mainly determined by the free water in the leaf tissue; more free water results in less reflectance. They are therefore called water absorption bands. When the leaves dry out, for an example during the harvest time of the crops, the plant may change colour (for example, to yellow). At this stage there is no photosynthesis, causing reflectance in the red portion of the spectrum to be higher. Also, the leaves will dry out resulting in higher reflectance in the middle infrared whereas the reflectance in the near infrared may decrease. As a result, optical remote sensing data provide information about the type of plant and also about its health condition. Vegetation reflectance Bare soil Surface reflectance from bare soil is dependent on so many factors that it is difficult to give one typical soil reflectance curve. However, the main factors influencing the soil reflectance are soil colour, moisture content, the presence of carbonates, and iron oxide content. Figure below gives some reflectance curves for the five main types of soil occurring in the USA. Note the typical shapes of most of the curves, which show a convex shape between 500–1300 nm with dips at 1450 and 1950 nm. These dips are so- called water absorption bands and are caused by the presence of soil moisture. The iron-dominated soil has a quite different reflectance curve that can be explained by the iron absorption dominating at longer wavelengths. Reflectance Spectra surface samples of five mineral soils a. Organic dominated b. Minimally altered c. Iron altered d. Organic affected e. Iron dominated Soil reflectance Water Compared to vegetation and soils, water has the low reflectance. Vegetation may reflect up to 50%, soils, up to 30–40% while water reflects at the most 10% of the incoming radiation. Water reflects EM energy in the visible up to the near-infrared. Beyond 1200 nm all energy is absorbed. Some curves of different types of water are given in Figure below. The highest reflectance is given by turbid (silt loaded) water and water containing plants with a chlorophyll reflection peak at the green wavelength. Typical effects of chlorophyll and sediments on water reflectance a. ocean water b.Turbid water c. Water with chlorophyll water reflectance For each material, a specific reflectance curve can be established. Such a curve shows the fraction of the incident radiation that is reflected as a function of a wavelength. Spectral reflectance curves