Chapter 26 Digestion, Absorption & Nutritional Principles PDF
Document Details
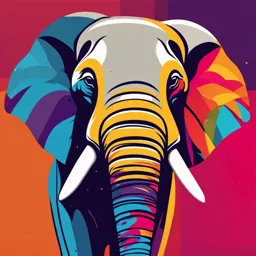
Uploaded by RadiantHyena
null
Tags
Summary
This document provides a detailed overview of digestion and absorption principles, covering carbohydrates, proteins, lipids, and nucleic acids. It discusses the role of enzymes, the importance of pH in digestion, and the various absorption mechanisms in the gastrointestinal tract. Different macronutrients and their corresponding digestive processes are explained.
Full Transcript
Chapter 26 Digestion, Absorption& Nutritional Principles 1 The gastrointestinal system is the portal through which nutritive substances, vitamins, minerals, and fluids enter the body. Proteins, fats, and complex carbohydrates are broken down into absorbable units (digested), principally, although no...
Chapter 26 Digestion, Absorption& Nutritional Principles 1 The gastrointestinal system is the portal through which nutritive substances, vitamins, minerals, and fluids enter the body. Proteins, fats, and complex carbohydrates are broken down into absorbable units (digested), principally, although not exclusively, in the small intestine. The products of digestion and the vitamins, minerals, and water cross the mucosa and enter the lymph or the blood (absorption). Enzymes from the salivary glands attack carbohydrates (and fats in some species); enzymes from the stomach attack proteins and fats; and enzymes from the exocrine portion of the pancreas attack carbohydrates, proteins, lipids, DNA, and RNA. Other enzymes that complete the digestive process are found in the luminal membranes and the cytoplasm of the cells that line the small intestine. The action of the enzymes is aided by the hydrochloric acid secreted by the stomach and the bile secreted by the liver. 2 DIGESTION & ABSORPTION: CARBOHYDRATES DIGESTION The principal dietary carbohydrates are polysaccharides, disaccharides, and monosaccharides. Starches (glucose polymers) and their derivatives are the only polysaccharides that are digested to any degree in the human gastrointestinal tract by human enzymes. Amylopectin, which typically constitutes around 75% of dietary starch, is a branched molecule, whereas amylose is a straight chain with only 1:4α linkages. The disaccharides lactose (milk sugar) and sucrose (table sugar) are also ingested, along with the monosaccharides fructose and glucose. 3 In the mouth, starch is attacked by salivary α-amylase. The optimal pH for this enzyme is 6.7 However, it remains partially active even once it moves into the stomach, despite the acidic gastric juice, because the active site is protected in the presence of substrate to some degree. In the small intestine, both the salivary and the pancreatic α-amylase also act on the ingested polysaccharides. The end products of α-amylase digestion are 1. Oligosaccharides (3 and 10 single sugar) 2. the disaccharide maltose 3. the trisaccharide maltotriose 4. α- dextrins 5. polymers of glucose containing an average of about eight glucose molecules. 4 The oligosaccharidases responsible for the further digestion of the starch derivatives are located in the brush border of small intestinal epithelial cells. Some of these enzymes have more than one substrate. Isomaltase is mainly responsible for hydrolysis of 1:6α linkages. Along with maltase and sucrase, it also breaks down maltotriose and maltose. Sucrase and isomaltase are initially synthesized as a single glycoprotein chain that is inserted into the brush border membrane. It is then hydrolyzed by pancreatic proteases into sucrase and isomaltase subunits. Sucrase hydrolyzes sucrose into (glucose +fructose). In addition, lactase hydrolyzes lactose to glucose + galactose. 5 6 The diarrhea is due to the increased number of osmotically active oligosaccharide molecules that remain in the intestinal lumen, causing the volume of the intestinal contents to increase. In the colon, bacteria break down some of the oligosaccharides, further increasing the number of osmotically active particles. The bloating and flatulence are due to the production of gas (CO2 and H2) from disaccharide residues in the lower small intestine and colon. 7 ABSORPTION Hexoses are rapidly absorbed across the wall of the small intestine. Essentially all the hexoses are removed before the remains of a meal reach the terminal part of the ileum. The sugar molecules pass from the mucosal cells to the blood in the capillaries draining into the portal vein. The transport of glucose and galactose depends on Na+ in the intestinal lumen; a high concentration of Na+ on the mucosal surface of the cells facilitates sugar influx into the epithelial cells while a low concentration inhibits sugar influx into the epithelial cells. This is because these sugars and Na+ share the same cotransporter, or symport, the sodium-dependent glucose transporter (SGLT, Na+ glucose cotransporter) 8 The members of this family of transporters, SGLT-1 and SGLT-2, resemble the glucose transporters (GLUTs) responsible for facilitated diffusion in that they cross the cell membrane 12 times and have their –COOH and –NH2 terminals on the cytoplasmic side of the membrane. However, there is no homology to the GLUT series of transporters. SGLT-1 is responsible for uptake of dietary glucose from the gut. SGLT-2, is responsible for glucose transport out of the renal tubules. 9 10 Because the intracellular Na+ concentration is low in intestinal cells (as it is in other cells), Na+ moves into the cell along its concentration gradient. Glucose moves with the Na+ and is released in the cell (Figure 26–2). The Na+ is transported into the lateral intercellular spaces, and the glucose is transported by GLUT2 into the interstitial and thence to the capillaries. Thus, glucose transport is an example of secondary active transport; the energy for glucose transport is provided indirectly, by the active transport of Na+ out of the cell. 11 This maintains the concentration gradient across the luminal border of the cell, so that more Na+ and consequently more glucose enter. When the Na+/glucose cotransporter is congenitally defective, the resulting glucose/galactose malabsorption causes severe diarrhea that is often fatal if glucose and galactose are not promptly removed from the diet. Glucose and its polymers can also be used to retain Na+ in diarrheal disease. 12 As indicated, SGLT-1 also transports galactose, but fructose utilizes a different mechanism. fructose absorption is independent of Na+ or the transport of glucose and galactose; it is transported instead by facilitated diffusion from the intestinal lumen into the enterocytes by GLUT5 and out of the enterocytes into the interstitial by GLUT2. Some fructose is converted to glucose in the mucosal cells. Insulin has little effect on intestinal transport of sugars. In this respect, intestinal absorption resembles glucose reabsorption in the proximal convoluted tubules of the kidneys ; neither process requires phosphorylation, and both are essentially normal in diabetes but are depressed by the drug phlorizin. The maximal rate of glucose absorption from the intestine is about 120 g/h. 13 PROTEINS & NUCLEIC ACIDS PROTEIN DIGESTION Protein digestion begins in the stomach, where pepsins cleave some of the peptide linkages. Like many of the other enzymes concerned with protein digestion, pepsins are secreted in the form of inactive precursors (proenzymes) and activated in the gastrointestinal tract. The pepsin precursors are called pepsinogens and are activated by gastric acid. Human gastric mucosa contains a number of related pepsinogens, which can be divided into two immunohistochemically distinct groups, pepsinogen I and pepsinogen II. Pepsinogen I is found only in acid-secreting regions, pepsinogen II is also found in the pyloric region. Maximal acid secretion correlates with pepsinogen I levels. 14 Pepsins hydrolyze the bonds between aromatic amino acids such as phenylalanine or tyrosine and a second amino acid, so the products of peptic digestion are polypeptides of very diverse sizes. Because pepsins have a pH optimum of 1.6–3.2, their action is terminated when the gastric contents are mixed with the alkaline pancreatic juice in the duodenum and jejunum. The pH of the intestinal contents in the duodenal bulb is 3.0–4.0, but rapidly rises; in the rest of the duodenum it is about 6.5. 15 In the small intestine, the polypeptides formed by digestion in the stomach are further digested by the powerful proteolytic enzymes of the pancreas and intestinal mucosa. Trypsin, the chymotrypsins, and elastase act at interior peptide bonds in the peptide molecules and are called endopeptidases. The formation of the active endopeptidases from their inactive precursors occurs only when they have reached their site of action, secondary to the action of the brush border hydrolase, enterokinase 16 17 The powerful protein-splitting enzymes of the pancreatic juice are secreted as inactive proenzymes. Trypsinogen is converted to the active enzyme trypsin by enterokinase when the pancreatic juice enters the duodenum. Trypsin converts chymotrypsinogens into chymotrypsins and other proenzymes into active enzymes (Figure 26–3). Trypsin can also activate trypsinogen; therefore, once some trypsin is formed, there is an auto-catalytic chain reaction. Enterokinase deficiency occurs as a congenital abnormality and leads to protein malnutrition. The carboxypeptidases of the pancreas are exopeptidases that hydrolyze the amino acids at the carboxyl ends of the polypeptides 18 19 Some free amino acids are liberated in the intestinal lumen, but others are liberated at the cell surface by the aminopeptidases, carboxypeptidases, endopeptidases, and dipeptidases in the brush border of the mucosal cells. Some dipeptides and tripeptides are actively transported into the intestinal cells and hydrolyzed by intracellular peptidases, with the amino acids entering the bloodstream. Thus, the final digestion to amino acids occurs in three locations: 1. the intestinal lumen 2. the brush border 3. the cytoplasm of the mucosal cells. 20 ABSORPTION At least seven different transport systems transport amino acids into enterocytes. Five of these require Na+ and cotransport amino acids and Na+ in a fashion similar to the cotransport of Na+ and glucose (Figure 26–3). Two of these five also require Cl–. In two systems, transport is independent of Na+. The dipeptides and tripeptides are transported into enterocytes by a system known as PepT1 (or peptide transporter 1) that requires H+ instead of Na+ 21 22 There is a very little absorption of larger peptides. In the enterocytes, amino acids released from the peptides by intracellular hydrolysis plus the amino acids absorbed from the intestinal lumen and brush border are transported out of the enterocytes along their basolateral borders by at least five transport systems. From there, they enter the hepatic portal blood. 23 Absorption of amino acids is rapid in the duodenum and jejunum. There is a little absorption in the ileum in health, because the majority of the free amino acids have already been assimilated at that point. Approximately 50% of the digested protein comes from ingested food, 25% from proteins in digestive juices, and 25% from desquamated mucosal cells. Only 2–5% of the protein in the small intestine escapes digestion and absorption. 24 Some of this is eventually digested by bacterial action in the colon. Almost all of the protein in the stools is not of dietary origin but comes from bacteria and cellular debris. Evidence suggests that the peptidase activities of the brush border and the mucosal cell cytoplasm are increased by resection of part of the ileum and that they are independently altered in starvation. Thus, these enzymes appear to be subject to homeostatic regulation. 25 In infants, moderate amounts of undigested proteins are also absorbed. The protein antibodies in maternal colostrum are largely secretory immunoglobulins (IgAs), the production of which is increased in the breast in late pregnancy. They cross the mammary epithelium by transcytosis and enter the circulation of the infant from the intestine, providing passive immunity against infections. Absorption is by endocytosis and subsequent exocytosis. 26 Absorption of intact proteins declines sharply after weaning, but adults still absorb small quantities. Foreign proteins that enter the circulation provoke the formation of antibodies, and the antigen–antibody reaction occurring on subsequent entry of more of the same protein may cause allergic symptoms. Thus, absorption of proteins from the intestine may explain the occurrence of allergic symptoms after eating certain foods. The incidence of food allergy in children is said to be as high as 8%. Certain foods are more allergenic than others. Crustaceans, mollusks, and fish are common offenders, and allergic responses to legumes, cows’ milk, and egg white are also relatively frequent. However, in most individual food allergies do not occur, and there is an evidence for a genetic component in susceptibility. 27 Absorption of protein antigens, particularly bacterial and viral proteins, takes place in large microfold cells or M cells, specialized intestinal epithelial cells that overlie aggregates of lymphoid tissue (Peyer patches). These cells pass the antigens to the lymphoid cells, and lymphocytes are activated. The activated lymphoblasts enter the circulation, but they later return to the intestinal mucosa and other epithelia, where they secrete IgA in response to subsequent exposures to the same antigen. 28 NUCLEIC ACIDS Nucleic acids are split into nucleotides in the intestine by the pancreatic nucleases, the nucleotides are split into the nucleosides and phosphoric acid by enzymes that appear to be located on the luminal surfaces of the mucosal cells. The nucleosides are then split into their constituent sugars and purine and pyrimidine bases. The bases are absorbed by active transport. Families of equilibrative (ie, passive) and concentrative (ie, secondary active) nucleoside transporters have recently been identified and are expressed on the apical membrane of enterocytes. 29 LIPIDS FAT DIGESTION A lingual lipase is secreted by Ebner glands on the dorsal surface of the tongue in some species, and the stomach also secretes a lipase. They are of little quantitative significance for lipid digestion other than in the setting of pancreatic insufficiency, but they may generate free fatty acids that signal to most distal parts of the gastrointestinal tract (eg, causing the release of CCK). 30 Most fat digestion therefore begins in the duodenum, pancreatic lipase being one of the most important enzymes involved. This enzyme hydrolyzes the 1- and 3-bonds of the triglycerides (triacylglycerols) with relative ease but acts on the 2-bonds at a very low rate, so the principal products of its action are free fatty acids and 2monoglycerides (2-monoacylglycerols). It acts on fats that have been emulsified (see below). 31 32 Colipase, a protein secreted in the pancreatic juice, and when this molecule binds to the –COOH- terminal domain of the pancreatic lipase, opening of the lid is facilitated. Colipase is secreted in an inactive preform and is activated in the intestinal lumen by trypsin. Colipase is also critical for the action of lipase because it allows lipase to remain associated with droplets of dietary lipid even in the presence of bile acids. 33 34 Another pancreatic lipase that is activated by bile acids has been characterized. This 100,000-kDa cholesterol esterase represents about 4% of the total protein in pancreatic juice. In adults, pancreatic lipase is 10–60 times more active as compared to cholesterol esterase cholesterol esterase catalyzes the hydrolysis of cholesterol esters, esters of fat-soluble vitamins, and phospholipids, as well as triglycerides. A very similar enzyme is found in human milk. 35 Fats are relatively insoluble, which limits their ability to cross the unstirred layer and reach the surface of the mucosal cells. However, they are finely emulsified in the small intestine by the detergent action of bile acids, phosphatidylcholine, and monoglycerides. When the concentration of bile acids in the intestine is high, as it is after contraction of the gallbladder, lipids and bile acids interact spontaneously to form micelles 36 37 These cylindrical aggregates (micellar) take up lipids, and although their lipid concentration varies, they generally contain fatty acids, monoglycerides, and cholesterol in their hydrophobic centers. Micellar formation further solubilizes the lipids and provides a mechanism for their transport to the enterocytes. Thus, the micelles move down their concentration gradient through the unstirred layer to the brush border of the mucosal cells. The lipids diffuse out of the micelles, and a saturated aqueous solution of the lipids is maintained in contact with the brush border of the mucosal cells (Figure 26–6). 38 Lipids collect in the micelles, with cholesterol in the hydrophobic center and amphipathic phospholipids and monoglycerides lined up with their hydrophilic heads on the outside and their hydrophobic tails in the center. The micelles play an important role in keeping lipids in solution and transporting them to the brush border of the intestinal epithelial cells, where they are absorbed. 39 FAT ABSORPTION Traditionally, lipids were thought to enter the enterocytes by passive diffusion, but some evidence now suggests that carriers are involved. Inside the cells, the lipids are rapidly esterified, maintaining a favorable concentration gradient from the lumen into the cell 40 41 There are also carriers that export certain lipids back into the lumen, thereby limiting their oral availability. This is the case for plant sterols as well as cholesterol. The fate of the fatty acids in enterocytes depends on their size. Fatty acids containing less than 10–12 carbon atoms are watersoluble enough that they pass through the enterocyte unmodified and are actively transported into the portal blood. They circulate as free (unesterified) fatty acids. 42 The fatty acids containing more than 10–12 carbon atoms are too insoluble for this. They are re-esterified to triglycerides in the enterocytes. In addition, some of the absorbed cholesterol is esterified. The triglycerides and cholesterol esters are then coated with a layer of protein, cholesterol, and phospholipid to form chylomicrons. These leave the cell and enter the lymphatics, because they are too large to pass through the junctions between capillary endothelial cells 43 In mucosal cells, most of the triglyceride is formed by the acylation of the absorbed 2-monoglycerides, primarily in the smooth endoplasmic reticulum. However, some of the triglyceride is formed from glycerophosphate, which in turn is a product of glucose catabolism. Glycerophosphate is also converted into glycerophospholipids that participate in chylomicron formation. The acylation of glycerophosphate and the formation of lipoproteins occur in the rough endoplasmic reticulum. Carbohydrate moieties are added to the proteins in the Golgi apparatus, and the finished chylomicrons are extruded by exocytosis from the basolateral aspect of the cell. 44 Absorption of long-chain fatty acids is greatest in the upper parts of the small intestine, but appreciable amounts are also absorbed in the ileum. On a moderate fat intake, 95% or more of the ingested fat is absorbed. The processes involved in fat absorption are not fully mature at birth, and infants fail to absorb 10–15% of ingested fat. Thus, they are more susceptible to the ill effects of disease processes that reduce fat absorption. 45 ABSORPTION of IRON In adults, the amount of iron lost from the body is relatively small. The losses are generally unregulated, and total body stores of iron are regulated by changes in the rate at which it is absorbed from the intestine. Men lose about 0.6 mg/d, largely in the stools. Premenopausal women have a variable, larger loss averaging about twice this value because of the additional iron lost during menstruation. 46 The average daily iron intake in the United States and Europe is about 20 mg, but the amount absorbed is equal only to the losses. Thus, the amount of iron absorbed is normally about 3–6% of the amount ingested. Various dietary factors affect the availability of iron for absorption; for example, the phytic acid found in cereals reacts with iron to form insoluble compounds in the intestine, as do phosphates and oxalates Most of the iron in the diet is in the ferric (Fe3+ ) form, whereas it is the ferrous (Fe2+ ) form that is absorbed. Fe 3+ reductase activity is associated with the iron transporter in the brush borders of the enterocytes 47 48 Gastric secretions dissolve the iron and permit it to form soluble complexes with ascorbic acid and other substances that aid its reduction to the Fe2+ form. The importance of this function in humans is indicated by the fact that iron deficiency anemia is a troublesome and relatively frequent complication of partial gastrectomy. Almost all iron absorption occurs in the duodenum. Transport of Fe2+ into the enterocytes occurs via divalent metal transporter 1 (DMT1) (Figure 26–8). Some is stored in ferritin, and the remainder is transported out of the enterocytes by a basolateral transporter named ferroportin 1. 49 A protein called hephaestin (Hp) is associated with ferroportin 1. It is not a transporter itself, but it facilitates basolateral transport. In the plasma, Fe 2+ is converted to Fe 3+ and bound to the iron transport protein transferrin. This protein has two iron-binding sites. Normally, transferrin is about 35% saturated with iron, and the normal plasma iron level is about 130 μg/dL (23 μmol/L) in men and 110 μg/dL (19 μmol/L) in women 50 Heme binds to an apical transport protein in enterocytes and is carried into the cytoplasm. In the cytoplasm, HO-2, a subtype of heme oxygenase, removes Fe2+ from the porphyrin and adds it to the intracellular Fe2+ pool. 70% of the iron in the body is in hemoglobin, 3% in myoglobin, and the rest in ferritin, which is present not only in enterocytes, but also in many other cells. 51 Apoferritin is a globular protein made up of 24 subunits. Ferritin is readily visible under the electron microscope and has been used as a tracer in studies of phagocytosis and related phenomena. Ferritin molecules in lysosomal membranes may aggregate in deposits that contain as much as 50% iron. These deposits are called hemosiderin. Intestinal absorption of iron is regulated by three factors: 1. recent dietary intake of iron 2. the state of the iron stores in the body 3. the state of erythropoiesis in the bone marrow. The normal operation of the factors that maintain iron balance is essential for health 52 53