Chapter 3: Carbon Footprints Across Product Lifecycles: Tackling Climate Change - PDF
Document Details
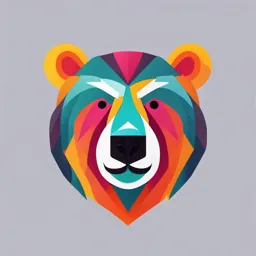
Uploaded by SelfDeterminationBeauty
Tags
Related
- Climate Heroes Guide: The 4 Keys to Combatting Climate Change at Home (PDF)
- Finance Durable Course Notes PDF 2023-2024
- Urban Farming Carbon Footprint 6x Higher Than Conventional Produce (PDF)
- Certified Carbon Auditing Professional (CAP) Exam - Association of Energy Engineers
- Guía de Rodaje Sostenible PDF
- Chapter 19: Biochar to Reduce Carbon Footprint PDF
Summary
This study explores the critical role of lifecycle carbon footprint analysis in addressing climate change. It examines methodologies for assessing carbon footprints, analyzes emission hotspots, and evaluates strategies for reducing emissions.
Full Transcript
# Chapter 3: Carbon Footprints Across Product Lifecycles: Tackling Climate Change from Production to Disposal ## Abstract This comprehensive study explores the critical role of lifecycle carbon footprint analysis in addressing climate change. As global efforts to mitigate greenhouse gas emissions...
# Chapter 3: Carbon Footprints Across Product Lifecycles: Tackling Climate Change from Production to Disposal ## Abstract This comprehensive study explores the critical role of lifecycle carbon footprint analysis in addressing climate change. As global efforts to mitigate greenhouse gas emissions intensify, understanding and managing the carbon footprint across entire product lifecycles has become increasingly important. This paper examines the methodologies for assessing carbon footprints, analyzes emission hotspots at various lifecycle stages, and evaluates strategies for reducing emissions from production to disposal. Through an extensive review of literature, case studies, and empirical data, this research demonstrates how lifecycle thinking can inform more effective climate change mitigation strategies. The study reveals that while certain lifecycle stages often dominate a product's carbon footprint, significant opportunities for emission reductions exist across the entire value chain. Moreover, the paper highlights the interconnectedness of decisions made at different lifecycle stages and their cumulative impact on overall emissions. The article proposes a holistic framework for integrating carbon footprint considerations into product design, manufacturing processes, usage patterns, and end-of-life management. It also explores the synergies between carbon footprint reduction strategies and other sustainability initiatives, such as circular economy approaches and renewable energy adoption. The paper concludes by outlining policy recommendations and future research directions to accelerate the transition towards low-carbon product lifecycles. ## Keywords Carbon Footprint, Lifecycle Assessment, Climate Change Mitigation, Greenhouse Gas Emissions, Sustainable Production, Circular Economy, Eco-design, Supply Chain Management, Waste Management, Environmental Policy # 1. Introduction The urgent need to address climate change has brought increased attention to the carbon footprints of products and services. As countries and organizations strive to meet ambitious emission reduction targets, there is a growing recognition that a lifecycle approach is crucial for identifying and implementing effective mitigation strategies. This article aims to provide a comprehensive exploration of carbon footprints across product lifecycles and their role in tackling climate change. ## 1.1 Background and Context The concept of carbon footprint, derived from the broader ecological footprint idea, has gained significant traction in recent years. It is typically defined as the total amount of greenhouse gases (GHGs) emitted throughout the lifecycle of a product or service, expressed in carbon dioxide equivalents (CO2e). The increasing focus on carbon footprints is driven by several factors: 1. **Climate Change Urgency:** The Intergovernmental Panel on Climate Change (IPCC) has emphasized the need for rapid and far-reaching transitions to limit global warming to 1.5°C above pre-industrial levels. 2. **Policy Developments:** Many countries have introduced carbon pricing mechanisms, emission reporting requirements, and product carbon footprint labeling schemes. 3. **Consumer Awareness:** There is growing consumer demand for low-carbon products and transparency in environmental impacts. 4. **Corporate Sustainability:** Companies are increasingly setting science-based targets for emission reductions, and integrating climate considerations into their strategies. 5. **Technological Advancements:** Improvements in data collection, life cycle assessment (LCA) methodologies, and carbon accounting tools have made lifecycle carbon footprint analysis more accessible and accurate. ## 1.2 Research Objectives This study addresses several key research questions: 1. What are the current methodologies for assessing carbon footprints across product lifecycles, and how can they be improved? 2. How do carbon emissions vary across different stages of a product's lifecycle, and what are the key hotspots? 3. What strategies can be employed to reduce carbon footprints at each lifecycle stage, from raw material extraction to end-of-life management? 4. How can lifecycle thinking inform more effective climate change mitigation policies and corporate strategies? 5. What are the challenges and opportunities in implementing lifecycle carbon footprint reduction strategies? 6. How do carbon footprint reduction efforts interact with other sustainability initiatives, such as circular economy approaches? 7. What role can emerging technologies play in measuring and reducing lifecycle carbon footprints? 8. How can the trade-offs between carbon footprint reduction and other environmental or social impacts be managed? 9. What are the implications of lifecycle carbon footprint analysis for global supply chains and international climate policy? 10. What should be the priorities for future research in this field? ## 1.3 Methodology To answer these questions, this article employs a mixed-methods approach, drawing on a wide range of sources: 1. **Literature Review:** An extensive review of peer-reviewed academic literature, industry reports, policy documents, and international standards on carbon footprint analysis and lifecycle assessment. 2. **Case Studies:** Analysis of carbon footprint reduction initiatives across various industries and product categories. 3. **Quantitative Analysis:** Examination of lifecycle carbon footprint data for different product types and sectors. 4. **Expert Interviews:** Insights from interviews with academics, policymakers, and practitioners in the fields of LCA, carbon management, and sustainable production. 5. **Policy Analysis:** Review of carbon-related policies and regulations at national and international levels. 6. **Scenario Modeling:** Use of lifecycle assessment models to project potential impacts of different carbon reduction strategies. This comprehensive approach allows for a holistic examination of carbon footprints across product lifecycles and their role in addressing climate change. # 2. Theoretical Foundations and Methodologies ## 2.1 Carbon Footprint Concept The carbon footprint concept has its roots in ecological footprint analysis but focuses specifically on greenhouse gas emissions. While there is no universally accepted definition, a widely used interpretation is: "A measure of the exclusive total amount of carbon dioxide emissions that is directly and indirectly caused by an activity or is accumulated over the life stages of a product". Key aspects of this definition include: 1. **Scope:** Encompasses both direct (Scope 1) and indirect (Scope 2 and 3) emissions. 2. **Life Cycle Perspective:** Considers emissions across all stages of a product's life. 3. **Carbon Dioxide Equivalents:** Usually expressed in terms of CO2e, which includes other greenhouse gases converted based on their global warming potential. ## 2.2 Life Cycle Assessment ( LCA ) Framework Life Cycle Assessment provides the methodological foundation for comprehensive carbon footprint analysis. The ISO 14040 and 14044 standards outline the principles and framework for LCA, which typically involves four phases: 1. **Goal and Scope Definition:** Defining the purpose of the study, the product system, functional unit, and system boundaries. 2. **Inventory Analysis:** Collecting data on inputs (resources, energy) and outputs (emissions, waste) for all processes within the defined system boundaries. 3. **Impact Assessment:** Converting inventory data into potential environmental impacts, focusing on global warming potential for carbon footprint studies. 4. **Interpretation:** Analyzing results, identifying significant issues, and drawing conclusions to inform decision-making. ## 2.3 Carbon Footprint Calculation Methodologies Several methodologies have been developed for calculating product carbon footprints: ### 2.3.1 PAS 2050 Developed by the British Standards Institution, PAS 2050 provides specifications for assessing the lifecycle GHG emissions of goods and services. Key features include: - Cradle-to-grave approach - Exclusion of capital goods - Specific guidance on land use change, carbon storage, and delayed emissions ### 2.3.2 GHG Protocol Product Standard The Greenhouse Gas Protocol's Product Life Cycle Accounting and Reporting Standard offers a comprehensive framework for quantifying and reporting product lifecycle GHG emissions. It emphasizes: - Flexibility in boundary setting - Guidance on allocation procedures - Requirements for uncertainty assessment ### 2.3.3 ISO 14067 The International Organization for Standardization's standard on carbon footprint of products provides principles, requirements, and guidelines for quantification and communication. It focuses on: - Alignment with ISO LCA standards - Specific requirements for publicly available studies - Guidance on carbon footprint communication ## 2.4 Emerging Approaches and Technologies Recent developments are enhancing the accuracy and applicability of carbon footprint assessments: ### 2.4.1 Environmentally Extended Input-Output (EEIO) Analysis EEIO combines economic input-output tables with environmental accounts to estimate emissions associated with economic activities. This approach: - Enables assessment of complex supply chains - Addresses some of the limitations of process-based LCA - Facilitates analysis at organizational and national levels ### 2.4.2 Hybrid LCA Hybrid approaches combine process-based LCA with input-output analysis to leverage the strengths of both methods. Benefits include: - Improved system boundary completeness - Reduced truncation errors - Better handling of capital goods ### 2.4.3 Artificial Intelligence and Big Data Emerging technologies are enhancing carbon footprint assessments: - Machine learning for data gap filling and scenario modeling - Internet of Things (IoT) for real-time data collection across supply chains - Blockchain for enhancing traceability and data reliability # 3. Carbon Footprints Across Product Lifecycles ## 3.1 Lifecycle Stages and Emission Hotspots Carbon emissions vary significantly across different stages of a product's lifecycle. Understanding these variations is crucial for identifying effective mitigation strategies. ### 3.1.1 Raw Material Extraction and Processing This stage often accounts for a significant portion of a product's lifecycle emissions, particularly for material-intensive goods: - Mining and Quarrying: Energy-intensive processes with significant land use impacts. - Agricultural Production: Emissions from soil management, livestock, and land use change. - Chemical Processing: High energy use and process emissions in industries like steel and cement. **Key hotspots:** - Energy use in extraction and processing - Land use change emissions - Process emissions (e.g., calcination in cement production) ### 3.1.2 Manufacturing and Assembly The carbon intensity of manufacturing varies widely depending on the product and processes involved: - Energy Use: Often the primary source of emissions, varying with the energy mix and efficiency of operations. - Process Emissions: Significant in some industries, such as semiconductor manufacturing. - Packaging: Can contribute substantially to the overall footprint, especially for consumer goods. **Key hotspots:** - Energy consumption in production processes - Fugitive emissions from industrial processes - Material waste and inefficiencies ### 3.1.3 Distribution and Retail Transportation and storage of products can contribute significantly to lifecycle emissions: - Freight Transport: Varies with mode (air, sea, road, rail) and distance. - Cold Chain: Refrigeration in transport and storage is energy-intensive. - Retail Operations: Energy use in stores and warehouses. **Key hotspots:** - Long-distance transportation, especially air freight - Refrigeration in cold chains - Energy use in retail spaces ### 3.1.4 Use Phase For many products, especially those that consume energy during use, this stage can dominate the lifecycle footprint: - Energy-Using Products: Appliances, vehicles, and electronic devices often have the highest emissions during use. - Consumables: Products like detergents or fuels have indirect use phase emissions. - Maintenance and Repair: Can extend product life but also contribute to emissions. **Key hotspots:** - Energy consumption during product use - Consumables and auxiliary materials - User behavior and usage patterns ### 3.1.5 End-of-Life Management The final stage of a product's life can have significant implications for its overall carbon footprint: - Waste Treatment: Emissions vary greatly between landfilling, incineration, and recycling. - Recycling: While often reducing overall emissions, recycling processes themselves have carbon footprints. - Reuse and Refurbishment: Can extend product life and avoid production of new goods. **Key hotspots:** - Methane emissions from landfills - Energy recovery efficiency in incineration - Transportation and processing in recycling systems ## 3.2 Variation Across Product Categories The distribution of emissions across lifecycle stages varies significantly between product types: ### 3.2.1 Fast-Moving Consumer Goods For products like food, beverages, and personal care items: - Agriculture and packaging often dominate for food products. - Use phase can be significant for products requiring refrigeration or heating. ### 3.2.2 Durable Consumer Goods For appliances, electronics, and furniture: - Manufacturing emissions are often significant due to complex materials and processes. - Use phase dominates for energy-using products like refrigerators or televisions. ### 3.2.3 Vehicles and Transportation For cars, airplanes, and other modes of transport: - Use phase typically dominates due to fuel consumption. - Manufacturing can be significant, especially for electric vehicles due to battery production. ### 3.2.4 Buildings and Infrastructure For construction projects and built environment: - Material production (e.g., cement, steel) often dominates initial emissions. - Use phase energy consumption is significant over long lifespans. ### 3.2.5 Services For intangible products like financial services or telecommunications: - Infrastructure and equipment often account for a large share of emissions. - Energy use in offices and data centers can be significant. Understanding these variations is crucial for prioritizing mitigation efforts and developing targeted strategies for different product categories. # 4. Strategies for Reducing Lifecycle Carbon Footprints Addressing carbon footprints across product lifecycles requires a multi-faceted approach, with strategies tailored to each stage of the lifecycle and specific product characteristics. ## 4.1 Design and Development Phase Decisions made during product design can have far-reaching impacts on lifecycle emissions: ### 4.1.1 Eco-design and Life Cycle Thinking Integrating environmental considerations from the earliest stages of product development: - Material Selection: Choosing low-carbon and renewable materials. - Design for Longevity: Increasing product lifespan to reduce replacement frequency. - Modular Design: Facilitating repair, upgrade, and end-of-life disassembly. ### 4.1.2 Dematerialization Reducing the material intensity of products and services: - Lightweight Design: Particularly important in transportation sectors. - Digitalization: Replacing physical products with digital alternatives where possible. - Functionality Focus: Designing products to deliver the required function with minimal resources. ### 4.1.3 Design for Energy Efficiency Minimizing energy consumption during the use phase: - Efficient Components: Using high-efficiency motors, heat exchangers, etc. - Smart Controls: Incorporating sensors and controls to optimize energy use. - Passive Design: Utilizing passive features to reduce active energy needs, especially in buildings. ## 4.2 Raw Material Sourcing and Processing Strategies to reduce emissions from the earliest stages of the supply chain: ### 4.2.1 Sustainable Sourcing Selecting raw materials with lower carbon footprints: - Recycled Materials: Increasing the use of recycled inputs. - Bio-based Materials: Utilizing renewable materials with potential for carbon sequestration. - Local Sourcing: Reducing transportation emissions. ### 4.2.2 Process Efficiency Improving the efficiency of raw material extraction and processing: - Energy Efficiency: Implementing best available technologies in energy-intensive processes. - Process Integration: Utilizing waste heat and byproducts to reduce overall energy consumption. - Electrification: Shifting from fossil fuels to electricity, coupled with renewable energy sources. ### 4.2.3 Carbon Capture and Utilization Mitigating emissions from unavoidable processes: - Process Emissions Capture: Implementing carbon capture in industries with high process emissions. - Carbon Utilization: Developing markets and applications for captured carbon. ## 4.3 Manufacturing and Assembly Strategies to reduce emissions during the production phase: ### 4.3.1 Energy Efficiency and Renewable Energy Reducing the carbon intensity of manufacturing operations: - Energy Management Systems: Implementing ISO 50001 or similar systems to continually improve energy performance. - On-site Renewable Energy: Installing solar PV, wind turbines, or other renewable energy systems. - Green Manufacturing: Adopting lean and clean production techniques to minimize waste and energy use. ### 4.3.2 Process Optimization Improving manufacturing processes to reduce material and energy waste: - Advanced Process Control: Utilizing AI and machine learning for process optimization. - Additive Manufacturing: Employing 3D printing and other additive techniques to reduce material waste. - Closed-loop Manufacturing: Recirculating materials and water within manufacturing processes ### 4.3.3 Low-carbon Packaging Reducing the carbon footprint of product packaging: - Material Reduction: Minimizing packaging material through innovative design. - Recycled Content: Increasing the use of recycled materials in packaging. - Biodegradable Packaging: Developing compostable packaging materials for appropriate applications. ## 4.4 Distribution and Retail Strategies to reduce emissions in the logistics and retail stages: ### 4.4.1 Sustainable Logistics Optimizing transportation and warehousing: - Mode Shifting: Moving from high-carbon transport modes (e.g., air freight) to lower-carbon alternatives (e.g., rail) where possible. - Route Optimization: Using advanced algorithms to minimize transportation distances. - Green Vehicles: Transitioning to electric or alternative fuel vehicles for logistics. ### 4.4.2 Cold Chain Optimization Improving the efficiency of refrigerated transport and storage: - Advanced Insulation: Using high-performance insulation materials to reduce cooling needs. - Natural Refrigerants: Replacing high-GWP refrigerants with natural alternatives. - Smart Temperature Management: Implementing IoT-based monitoring and control systems. ### 4.4.3 Retail Energy Efficiency Reducing emissions from retail operations: - Building Efficiency: Implementing energy-efficient lighting, HVAC, and refrigeration systems in stores. - Behavioral Changes: Training staff on energy-saving practices. - Demand Response: Participating in grid flexibility programs to support renewable energy integration. ## 4.5 Use Phase Strategies to reduce emissions during product use: ### 4.5.1 Energy Efficiency Improvements Enhancing the energy performance of products: - Technological Advancements: Continually improving the energy efficiency of appliances and devices. - Smart Functionality: Incorporating smart features that optimize energy use based on user behavior. - Energy Labeling: Providing clear information to consumers about energy consumption. ### 4.5.2 Longevity and Repairability Extending product lifespans to reduce replacement frequency: - Durable Design: Using high-quality materials and construction techniques to increase product durability - Right to Repair: Designing products for easy repair and providing access to spare parts and repair information. - Upgradability: Allowing for component upgrades to extend functional lifespan. ### 4.5.3 User Behavior Influencing user behavior to reduce use-phase emissions: - Eco-feedback: Providing real-time information on energy consumption to users. - Default Settings: Setting energy-efficient modes as the default option. - Gamification: Using game-like elements to encourage energy-saving behaviors. ## 4.6 End-of-Life Management Strategies to minimize emissions and maximize resource recovery at product end-of-life: ### 4.6.1 Design for Circularity Facilitating product reuse, remanufacturing, and recycling: - Easy Disassembly: Designing products for efficient dismantling and material separation. - Material Labeling: Clearly marking materials to facilitate sorting and recycling. - Mono-material Design: Using single materials or compatible material combinations where possible. ### 4.6.2 Effective Collection and Sorting Improving end-of-life product management systems: - Reverse Logistics: Developing efficient systems for product take-back and collection. - Advanced Sorting Technologies: Implementing AI and robotics in waste sorting facilities. - Incentive Schemes: Introducing deposit-refund systems or other incentives for product return. ### 4.6.3 High-value Recycling Maximizing the value and carbon benefits of recycling: - Chemical Recycling: Developing advanced recycling technologies for plastics and other materials. - Upcycling: Finding higher-value applications for recycled materials. - Closed-loop Recycling: Implementing systems to recycle materials back into the same product category. # 5. Challenges and Opportunities in Implementation While the strategies outlined above offer significant potential for reducing lifecycle carbon footprints, their implementation faces several challenges: ## 5.1 Technical Challenges ### 5.1.1 Data Availability and Quality Accurate carbon footprint assessment requires comprehensive data across the entire value chain: - Supply Chain Complexity: Gathering data from multiple tiers of suppliers can be challenging. - Data Inconsistency: Variations in data collection and reporting methods can lead to inconsistencies. - Dynamic Nature: Rapid changes in technologies and processes can quickly outdateexisting data. ### 5.1.2 Methodological Limitations Current carbon footprint assessment methodologies have several limitations: - System Boundary Definition: Decisions on what to include or exclude can significantly impact results. - Allocation Procedures: Different methods for allocating emissions between co-products can lead to varying results. - Future Scenario Modeling: Predicting future energy mixes and technologies introduces uncertainties. ### 5.1.3 Trade-offs with Other Environmental Impacts Focusing solely on carbon footprint may lead to unintended consequences in other impact categories: - Burden Shifting: Reducing carbon emissions might increase other impacts like water use or toxicity. - Complexity of Multi-criteria Decision Making: Balancing carbon reduction with other environmental and social objectives can be challenging. ## 5.2 Economic Challenges ### 5.2.1 Investment Costs Many carbon reduction strategies require significant upfront investments: - Technology Upgrades: Implementing energy-efficient or low-carbon technologies often involves high capital costs. - Research and Development: Developing innovative low-carbon solutions requires substantial R&D investment. - Infrastructure Changes: Transitioning to new energy systems or circular economy models may require large-scale infrastructure investments. ### 5.2.2 Market Barriers Existing market structures can hinder the adoption of low-carbon alternatives: - Price Signals: Low prices for virgin materials and fossil fuels can make circular and low-carbon alternatives less competitive. - Split Incentives: In some cases, those investing in carbon reduction measures may not directly benefit from the savings. - Market Inertia: Established industries and technologies benefit from economies of scale and learning effects, making it difficult for new solutions to compete. ## 5.3 Regulatory and Policy Challenges ### 5.3.1 Policy Fragmentation The lack of consistent policies across regions can create challenges for global-supply chains: - Varying Standards: Different countries may have different carbon accounting and reporting requirements. - Regulatory Uncertainty: Frequent changes in climate policies can create investment uncertainties. - International Coordination: Addressing global supply chain emissions requires coordinated action across countries. ### 5.3.2 Inadequate Pricing of Externalities The failure to fully internalize the costs of carbon emissions in market prices: - Carbon Pricing: Many jurisdictions lack carbon pricing mechanisms, or prices are too low to drive significant change. - Subsidies: Continued subsidies for fossil fuels and carbon-intensive industries distort markets. # 5.4 Social and Behavioral Challenges ## 5.4.1 Consumer Acceptance Shifting consumer preferences towards low-carbon products and lifestyles: - Awareness: Many consumers lack understanding of product carbon footprints and their impact - Willingness to Pay: Consumers may be reluctant to pay premiums for low-carbon alternatives. - Behavioral Inertia: Changing established consumption patterns and habits can be difficult. ## 5.4.2 Organizational Culture Embedding carbon consciousness into organizational decision-making: - Short-term Focus: Pressure for short-term financial results can hinder long-term investments in carbon reduction. - Siloed Thinking: Traditional organizational structures may not facilitate the cross-functional collaboration needed for lifecycle carbon management. # 5.5 Opportunities for Overcoming Challenges Despite these challenges, several developments present opportunities for accelerating the transition to low-carbon product lifecycles: ## 5.5.1 Technological Advancements Emerging technologies are enhancing our ability to measure, track, and reduce carbon footprints: - Digital Twins: Creating digital representations of products and processes to optimize performance and reduce emissions. - Blockchain: Enhancing supply chain transparency and traceability of carbon emissions. - Artificial Intelligence: Optimizing processes and enabling more accurate predictive modeling of carbon impacts. ## 5.5.2 Business Model Innovation New business models are aligning profitability with carbon reduction: - Product-as-a-Service: Shifting from selling products to providing services, incentivizing resource efficiency. - Sharing Economy: Increasing asset utilization through shared use models. - Industrial Symbiosis: Creating closed-loop systems where waste from one process becomes input for another. ## 5.5.3 Policy Innovations Innovative policy approaches are emerging to drive carbon reductions: - Carbon Border Adjustments: Addressing carbon leakage and creating a level playing field for low-carbon products. - Green Public Procurement: Using government purchasing power to create markets for low-carbon products. - Regulatory Sandboxes: Creating controlled environments for testing innovative low-carbon solutions. ## 5.5.4 Collaborative Initiatives Cross-sector collaborations are driving systemic changes: - Science-Based Targets: Companies setting emission reduction targets aligned with climate science. - Sector Roadmaps: Industries developing collaborative plans for decarbonization. - Pre-competitive Collaboration: Companies working together on shared challenges in carbon reduction. # 6. Future Directions and Research Needs As the field of lifecycle carbon footprint analysis and management continues to evolve, several key areas emerge as priorities for future research and development: ## 6.1 Methodological Advancements ### 6.1.1 Dynamic LCA Developing methods to better account for temporal aspects in carbon footprint assessments: - Time-dependent Characterization: Incorporating time-sensitive impact assessment methods. - Prospective LCA: Enhancing techniques for modeling future scenarios and technologies. - Real-time LCA: Developing capabilities for continuous, real-time carbon footprint assessment. ### 6.1.2 Consequential Approaches Improving understanding of the systemic consequences of product lifecycle decisions: - Market-mediated Effects: Better modeling of how changes in product systems affect wider market dynamics. - Rebound Effects: Developing methods to account for potential increases in consumption due to efficiency improvements. - Macro-level Consequences: Linking product-level decisions to economy-wide and global-level carbon impacts. ### 6.1.3 Integration with Other Assessment Methods Combining carbon footprint analysis with other sustainability assessment tools: - Multi-criteria Assessment: Developing frameworks that balance carbon reduction with other environmental and social objectives. - Economic Assessment: Integrating carbon footprint analysis with life cycle costing and broader economic assessments. - Risk Assessment: Incorporating climate-related risk analysis into product carbon footprint assessments. ## 6.2 Data and Digitalization ### 6.2.1 Big Data Analytics Leveraging large datasets to enhance carbon footprint analysis: - Supply Chain Mapping: Using big data techniques to map complex global supply chains and their associated emissions. - Consumer Behavior Analysis: Analyzing large-scale data on product use patterns to inform design and policy decisions. - Predictive Modeling: Developing advanced predictive models for carbon footprint scenarios using machine learning techniques. ### 6.2.2 Internet of Things (IoT) and Sensors Utilizing IoT technologies for real-time carbon footprint monitoring: - Smart Products: Embedding sensors in products to track actual use-phase emissions. - Connected Supply Chains: Implementing IoT solutions for real-time tracking of emissions across supply chains. - Smart Grids: Leveraging IoT in energy systems to optimize low-carbon energy use in product lifecycles. ### 6.2.3 Blockchain and Distributed Ledger Technologies Exploring the potential of blockchain for enhancing transparency and traceability in carbon footprint data: - Carbon Credit Tracking: Using blockchain to create more transparent and efficient carbon credit systems. - Product Passports: Developing blockchain-based digital passports to track the carbon footprint of products throughout their lifecycle. - Smart Contracts: Implementing automated, blockchain-based contracts to incentivize and verify carbon reductions in supply chains. ## 6.3 Policy and Governance ### 6.3.1 Carbon Pricing Mechanisms Researching effective carbon pricing strategies to internalize the cost of carbon emissions: - Global Carbon Markets: Exploring the potential for linked international carbon markets. - Sector-specific Approaches: Developing tailored carbon pricing mechanisms for different industries. - Carbon Border Adjustments: Analyzing the impacts and implementation challenges of carbon border adjustment mechanisms. ### 6.3.2 Circular Economy Policies Investigating policy frameworks that support circular economy principles for carbon reduction: - Extended Producer Responsibility: Evaluating and improving EPR schemes to drive lifecycle carbon reductions. - Right to Repair: Analyzing the carbon impact of policies that enhance product repairability and longevity. - Material Efficiency Standards: Developing policies to promote material efficiency and recycled content in products. ### 6.3.3 International Coordination Researching mechanisms for improved international cooperation on lifecycle carbon management: - Trade Policies: Analyzing the role of trade agreements in promoting low-carbon product lifecycles. - Technology Transfer: Investigating effective mechanisms for low-carbon technology transfer between countries. - Global Sectoral Approaches: Exploring the potential for internationally coordinated approaches to decarbonization in specific sectors. ## 6.4 Social and Behavioral Aspects ### 6.4.1 Consumer Behavior Deepening understanding of consumer behavior related to low-carbon products: - Choice Architecture: Researching how product presentation and information can nudge consumers towards low-carbon choices. - Cultural Differences: Investigating how cultural factors influence acceptance of low-carbon products and lifestyles. - Social Influence: Exploring the role of social networks and peer influence in promoting low-carbon consumption patterns. ### 6.4.2 Business Decision-Making Investigating how businesses integrate carbon footprint considerations into decision-making: - Carbon Risk Assessment: Developing frameworks for businesses to assess and manage carbon-related risks - Internal Carbon Pricing: Evaluating the effectiveness of internal carbon pricing mechanisms in driving corporate decarbonization. - Organizational Learning: Studying how organizations develop capabilities for managing lifecycle carbon footprints. ### 6.4.3 Just Transition Researching strategies to ensure the transition to low-carbon product lifecycles is socially equitable: - Employment Impacts: Analyzing the effects of lifecycle carbon management strategies on employment and skills requirements. - Distributional Effects: Investigating the distributional impacts of carbon pricing and other policies across different socioeconomic groups. - Community Engagement: Exploring effective methods for engaging communities in the transition to low-carbon production and consumption systems. # 7. Conclusion ## 7.1 Key Findings This comprehensive review of carbon footprints across product lifecycles has revealed several key insights: 1. **Lifecycle Perspective:** Adopting a lifecycle perspective is crucial for effectively tackling climate change through product-related emissions. Focusing on individual lifecycle stages in isolation can lead to suboptimal outcomes and potential burden-shifting. 2. **Variability Across Products:** The distribution of carbon emissions across lifecycle stages varies significantly between product categories. This underscores the need for tailored strategies for different types of products and services. 3. **Hotspot Identification:** Lifecycle carbon footprint analysis enables the identification of emissions hotspots, allowing for targeted interventions that can yield the most significant reductions. 4. **Multifaceted Strategies:** Effective carbon footprint reduction requires a combination of strategies, including eco-design, process efficiency, renewable energy adoption, circular economy approaches, and behavior change. 5. **Systemic Challenges:** While numerous strategies for reducing lifecycle carbon footprints exist, their implementation faces systemic challenges related to data availability, economic structures, regulatory frameworks, and established behaviors. 6. **Technological Opportunities:** Emerging technologies, particularly in the realms of digitalization and advanced materials, offer significant potential for enhancing our ability to measure, track, and reduce carbon footprints. 7. **Policy Role:** Policy interventions, especially in the areas of carbon pricing, circular economy promotion, and international coordination, are crucial for creating an enabling environment for low-carbon product lifecycles. 8. **Social Dimensions:** The transition to low-carbon product lifecycles has important social implications, including changes in employment, consumption patterns, and distributional effects, which need to be carefully managed. ## 7.2 Implications The findings of this study have significant implications for various stakeholders: 1. Businesses need to adopt a lifecycle perspective in their product development and operational strategies, integrating carbon considerations from design to end-of-life management, balancing regulatory measures with market-based instruments and supportive policies. 2. Consumers have a crucial role to play in driving demand for low-carbon products and adopting more sustainable consumption patterns. 3. Researchers need to continue advancing methodologies for lifecycle carbon footprint assessment and developing innovative solutions for carbon reduction across product lifecycles. 4. International organizations have an important part in facilitating global cooperation and knowledge sharing on lifecycle carbon management strategies. ## 7.3 Future Outlook As we move towards a low-carbon future, the management of product lifecycle carbon footprints will become increasingly critical. Several trends are likely to shape this field in the coming years: 1. **Increased Integration:** Greater integration of carbon footprint considerations into broader sustainability assessments and business decision-making processes. 2. **Digitalization:** Continued advancements in digital technologies enabling more accurate, real-time carbon footprint tracking and management. 3. **Circular Economy:** Growing emphasis on circular economy principles as a key strategy for reducing lifecycle carbon footprints. 4. **Policy Evolution:** Development of more sophisticated and internationally coordinated policy frameworks for addressing product lifecycle emissions. 5. **Consumer Engagement:** Increasing consumer awareness and engagement with product carbon footprints, driven by improved labeling, digital tools, and education. 6. **Innovation Acceleration:** Rapid innovation in low-carbon materials, technologies, and business models, spurred by increasing climate urgency and supportive policies. In conclusion, addressing carbon footprints across product lifecycles presents both a significant challenge and a crucial opportunity in tackling climate change. It requires a systems-level transformation of how we design, produce, use, and manage the end-of-life of products. While the path ahead is complex, the potential for innovation, value creation, and environmental improvement is immense. By embracing lifecycle thinking and fostering collaboration across sectors and value chains, we can accelerate the transition to a low-carbon, climate-resilient future. # References Ahman, M., Nilsson, L. J., & Johansson, B. (2017). Global climate policy and deep decarbonization of energy-intensive industries. *Climate Policy*, 17(5), 634-649. Ahluwalia, M. B. (2017). The business of pricing carbon: How companies are pricing carbon to mitigate risks and prepare for a low-carbon future. *Center for Climate and Energy Solutions*. Allwood, J. M., Ashby, M. F., Gutowski, T. G., & Worrell, E. (2011). Material efficiency: A white paper. *Resources, Conservation and Recycling*, 55(3), 362-381. Axon, S., Morrissey, J., & Aiesha, R. (2018). The Role of Social Networks in Low-Carbon Living. *In Low Carbon Energy Transitions* (pp. 143-165). Palgrave Macmillan, Cham.