Enzymes: Mechanism of Action PDF
Document Details
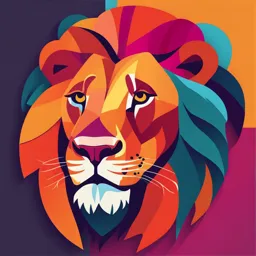
Uploaded by FieryComprehension3097
Palawan State University
Peter J. Kennelly & Victor W. Rodwell
Tags
Summary
This chapter discusses enzymes, their mechanisms of action, and their roles in biological processes. It covers the structural relationships between vitamins and coenzymes, and the mechanistic strategies employed by enzymes for catalyzing reactions. The chapter also explores enzyme-linked immunoassays, the role of restriction enzymes, and site-directed mutagenesis.
Full Transcript
C H A P T E R Enzymes: Mechanism of Action Peter J. Kennelly, PhD, & Victor W. Rodwell, PhD...
C H A P T E R Enzymes: Mechanism of Action Peter J. Kennelly, PhD, & Victor W. Rodwell, PhD 7 OBJ E C TI VE S Describe the structural relationships between specific B vitamins and certain coenzymes. After studying this chapter, Outline the four principal mechanistic strategies employed by enzymes to you should be able to: catalyze chemical reactions. Explain the concepts of the “lock and key” and “induced fit” models for enzyme– substrate interaction and how the latter accounts for the dynamic nature of enzyme catalysis. Outline the underlying principles of enzyme-linked immunoassays. Describe how detecting and measuring the activity of many enzymes can be facilitated by coupling with an appropriate dehydrogenase. Identify proteins whose plasma levels are used as biomarkers for diagnosis and prognosis. Describe the application of restriction endonucleases and of restriction fragment length polymorphisms in the detection of genetic diseases. Illustrate the utility of site-directed mutagenesis for the identification of aminoacyl residues that are involved in the recognition of substrates or allosteric effectors, or in the mechanism of catalysis. Describe how “affinity tags” can facilitate purification of a protein expressed from its cloned gene. Discuss the events that led to the discovery that RNAs can act as enzymes, and briefly describe the evolutionary concept of an “RNA world.” BIOMEDICAL IMPORTANCE of key enzymes that resut from genetic efects, nutritiona eficits, tissue amage, toxins, or infection by vira or bacteria Nobe aureate (1946) James Sumner efine an enzyme as pathogens (eg, Vibrio cholerae). Thus, the abiity to etect an “a catayst of high moecuar weight an bioogica origin.” to quantify the activity of specific enzymes in boo, other tissue Enzymes catayze the chemica reactions that make ife on fuis, or ce extracts provies information that enhances the the earth possibe, such as the breakown of nutrients to sup- physician’s abiity to iagnose many iseases. py energy an biomoecuar buiing bocks; the assemby In aition to serving as the cataysts for a metaboic pro- of those buiing bocks into proteins, DNA, membranes, cesses, the impressive cataytic activity an substrate specificity ces, an tissues; an the harnessing of energy to power ce of enzymes enabes them to fufi unique roes in human heath motiity, neura function, an musce contraction. Amost a an we-being. The protease rennin, for exampe, is utiize in enzymes are proteins. Notabe exceptions incue ribosoma the prouction of cheeses, whie actase is empoye to remove RNAs an a hanfu of RNA moecues imbue with eno- actose from mik to benefit actose-intoerant iniviuas. nucease or nuceotie igase activity known coectivey as Proteases an amyases augment the capacity of etergents to ribozymes. Many pathoogic conitions are the irect conse- remove irt an stains, whie other enzymes can participate in quence of changes in the quantity or in the cataytic activity the stereospecific synthesis of compex rugs or antibiotics. 59 60 SECTION II Enzymes: Kinetics, Mechanism, Regulation, & Role of Transition Metals 4 inicating the particuar substrate on which the enzyme acts (xanthine oxiase), its source (pancreatic ribonucease), its moe of reguation (hormone-sensitive ipase), or a charac- 1 1 3 teristic feature of its mechanism of action (cysteine protease). Where neee, aphanumeric esignators can be ae to 3 ientify mutipe forms, or isozymes, of an enzyme (eg, RNA 2 poymerase III; protein kinase Cβ). 2 As more enzymes were iscovere, these eary naming Enzyme site Substrate conventions increasingy resute in the inavertent esigna- tion of some enzymes by mutipe names or the assignment of FIGURE 7–1 Planar representation of the “three-point upicate names to enzymes exhibiting simiar cataytic capa- attachment” of a substrate to the active site of an enzyme. biities. To aress these probems, the Internationa Union of Although atoms 1 and 4 are identical, once atoms 2 and 3 are bound to their complementary sites on the enzyme, only atom 1 can bind. Biochemistry (IUB) eveope a system of enzyme nomen- Once bound to an enzyme, apparently identical atoms thus may be cature in which each enzyme has a unique name an coe distinguishable, permitting a stereospecific chemical change. number that ientify the type of reaction catayze an the substrates invove. Enzymes are groupe into the foowing six casses: ENZYMES ARE EFFICIENT & 1. Oxidoreductases—enzymes that catayze oxiations an HIGHLY SPECIFIC CATALYSTS reuctions The enzymes that catayze the conversion of one or more com- 2. Transferases—enzymes that catayze transfer of moieties pouns (substrates) into one or more ifferent compouns such as gycosy, methy, or phosphory groups (products) generay enhance the rates of the corresponing noncatayze reaction by factors of 106 to 109 or even more. 3. Hydrolases—enzymes that catayze hydrolytic ceavage of Athough some enzymes become moifie uring cataysis, C—C, C—O, C—N, an other covaent bons these transient aterations are resove in the course of the 4. Lyases—enzymes that catayze ceavage of C—C, C—O, reaction being catayze. In aition to being highy efficient, C—N, an other covaent bons by atom elimination, gen- enzymes are aso extremey selective. Typicay, the cataysts erating oube bons use in synthetic chemistry are specific for the type of reac- tion catayze an wi act on any compoun containing the 5. Isomerases—enzymes that catayze geometric or structura reevant functiona group. Enzymes, however, are generay changes within a moecue specific for a singe substrate or even a singe stereoisomer 6. Ligases—enzymes that catayze the joining together (igation) thereof—for exampe, d- but not l-sugars, l- but not d-amino of two moecues in reactions coupe to the hyroysis of ATP acis—or a sma set of cosey reate substrates. Since they bin substrates through at east “three points of attachment” The IUB name of hexokinase is ATP:D-hexose 6- (Figure 7–1), enzymes aso can prouce chira proucts from phosphotransferase E.C. 2.7.1.1. This name ientifies hexoki- nonchira substrates. For exampe, reuction of the nonchira nase as a member of cass 2 (transferases), subcass 7 (transfer substrate pyruvate by actate ehyrogenase prouces excu- of a phosphory group), sub-subcass 1 (acoho is the phos- sivey l-actate, not a racemic mixture of d- an l-actate. The phory acceptor), an “hexose-6” inicates that the acoho exquisite specificity of enzyme cataysts imbues iving ces phosphoryate is on carbon six of a hexose. Whie EC num- with the abiity to simutaneousy conuct an inepenenty bers have proven particuary usefu to ifferentiate enzymes contro a broa spectrum of biochemica processes. with simiar functions or simiar cataytic activities, IUB names ten to be engthy an cumbersome. Consequenty, hexokinase an many other enzymes commony are referre ENZYMES ARE CLASSIFIED BY to using their traitiona, abeit sometimes ambiguous names. REACTION TYPE Some of the names for enzymes first escribe in the eari- PROSTHETIC GROUPS, est ays of biochemistry persist in use to this ay. Exampes COFACTORS, & COENZYMES PLAY incue pepsin, trypsin, an amyase. Eary biochemists gener- ay name newy iscovere enzymes by aing the suffix –ase IMPORTANT ROLES IN CATALYSIS to a escriptor for the type of reaction catayze. For exampe, Many enzymes contain sma moecues or meta ions that par- enzymes that remove the eements of hyrogen, H2 or H− ticipate irecty in substrate bining or in cataysis. Terme pus H+, generay are referre to as ehyrogenases, enzymes prosthetic groups, cofactors, an coenzymes, they exten the that hyroyze proteins as proteases, an enzymes that cata- repertoire of mechanistic capabiities beyon those affore yze rearrangements in configuration as isomerases. In many by the functiona groups present on the aminoacy sie chains cases, these genera escriptors were suppemente with terms of pepties. CHAPTER 7 Enzymes: Mechanism of Action 61 Prosthetic Groups O Prosthetic groups are tighty an staby incorporate into a NH2 protein’s structure by covaent bons or noncovaent forces. + N Exampes incue pyrioxa phosphate, favin mononuceo- tie (FMN), favin aenine inuceotie (FAD), thiamin pyro- O CH2 phosphate, ipoic aci, biotin, an transition metas such as Fe, O Co, Cu, Mg, Mn, an Zn. Meta ions that participate in reox reactions generay are boun as organometaic compexes H H such as the prosthetic groups heme or iron–sufur custers (see HO OH O P O– Chapter 10). Metas may faciitate the bining an orientation of substrates, the formation of covaent bons with reaction NH2 intermeiates (Co2+ in coenzyme B12, see Chapter 44), or by N acting as Lewis acis or bases to rener substrates more elec- N trophilic (eectron-poor) or nucleophilic (eectron-rich), an O N N hence more reactive (see Chapter 10). O P O CH2 Cofactors Associate Reversibly With O – O Enzymes or Substrates H H Cofactors serve functions simiar to those of prosthetic groups HO OR an overap with them. The major ifference between the two is operationa, not chemica. Cofactors bin weaky an tran- FIGURE 7–2 Structure of NAD+ and NADP+. For NAD+, OR = sienty to their cognate enzymes or substrates, forming is- —OH. For NADP+, —OR = —OPO32–. sociabe compexes. Therefore, unike associate prosthetic groups, cofactors must be present in the surrouning environ- permeate ces. Secon, they increase the number of points of ment to promote compex formation in orer for cataysis to contact between substrate an enzyme, which increases the occur. Meta ions form the most numerous cass of cofactors. affinity an specificity with which sma chemica groups such Enzymes that require a meta ion cofactor are terme metal- as acetate (coenzyme A), gucose (UDP), or hyrie (NAD+) activated enzymes to istinguish them from the metalloen- are boun by their target enzymes. Other chemica moieties zymes for which boun meta ions serve as prosthetic groups. transporte by coenzymes incue methy groups (foates) an It is estimate that one-thir of a enzymes fa into one of oigosaccharies (oicho). these two groups. Many Coenzymes, Cofactors, CATALYSIS OCCURS AT & Prosthetic Groups Are Derivatives THE ACTIVE SITE of B Vitamins An important eary 20th-century insight into enzymic catay- sis sprang from the observation that the presence of substrates The water-soube B vitamins suppy important components reners enzymes more resistant to the enaturing effects of of numerous coenzymes. Nicotinamide is a component of the eevate temperatures. This observation e Emi Fischer to reox coenzymes NAD an NADP (Figure 7–2); riboflavin propose that enzymes (E) an their substrates (S) form an is a component of the reox coenzymes FMN an FAD; pan- enzyme–substrate (ES) compex whose therma stabiity is tothenic acid is a component of the acy group carrier coen- greater than that of the enzyme itsef. This insight profouny zyme A. As its pyrophosphate thiamin participates in the shape our unerstaning of both the chemica nature an ecarboxyation of α-keto acis whie foic aci an cobamie kinetic behavior of enzymic cataysis. coenzymes function in one-carbon metaboism. In aition, Fischer reasone that the exquisitey high specificity with severa coenzymes contain the aenine, ribose, an phosphory which enzymes iscriminate their substrates when forming an moieties of AMP or ADP (see Figure 7–2). ES compex was anaogous to the manner in which a mechani- ca ock istinguishes the proper key. The anaogy to enzymes is Coenzymes Serve as Substrate Shuttles that the “ock” is forme by a surface-accessibe ceft or pocket Coenzymes serve as recycabe shuttes that transport many in the enzyme cae the active site (see Figures 5–6 an 5–8). substrates from one point within the ce to another. The func- As impie by the ajective “active,” the active site is much tion of these shuttes is twofo. First, they stabiize species more than simpy a recognition site for bining substrates; it such as hyrogen atoms (FADH2) or hyrie ions (NADH) provies the environment wherein chemica transformation that are too reactive to persist for any significant time in the takes pace. Within the active site, substrates are brought into presence of the water, oxygen, or the organic moecues that cose proximity with one another in optima aignment with 62 SECTION II Enzymes: Kinetics, Mechanism, Regulation, & Role of Transition Metals Arg 145 Acid–Base Catalysis NH In aition to contributing to the abiity of the active site to OH + NH2 C NH2 bin substrates, the ionizabe functiona groups of aminoacy sie chains an, where present, of prosthetic groups, can con- O tribute to cataysis by acting as acis or bases. We istinguish H H C O O two types of aci–base cataysis. Specific acid or base cataly- N H C C sis refers to reactions for which the ony participating acis N H Tyr 248 H or bases are protons or hyroxie ions. The rate of reaction N C His 196 CH2 thus is sensitive to changes in the concentration of protons O Zn2+ or hyroxie ions, but is independent of the concentrations of NH2 O O other acis (proton onors) or bases (proton acceptors) pres- His 69 C N ent in the soution or at the active site. Reactions whose rates Glu 72 are responsive to all the acis or bases present are sai to be N subject to general acid catalysis or general base catalysis. H FIGURE 7–3 Two-dimensional representation of a dipeptide Catalysis by Strain substrate, glycyl-tyrosine, bound within the active site of For cataysis of ytic reactions, which invove breaking a covaent carboxypeptidase A. bon, enzymes typicay bin their substrates in a conforma- tion that weakens the bon targete for ceavage through physica the cofactors, prosthetic groups, an aminoacy sie chains istortion an eectronic poarization. This straine conforma- that participate in catayzing the transformation of substrates tion mimics that of the transition state intermediate, a transient into proucts (Figure 7–3). Cataysis is further enhance by species that represents the miway point in the transformation the capacity of the active site to shie substrates from water of substrates to proucts. Nobe Laureate Linus Pauing was the an generate an environment whose poarity, hyrophobicity, first to suggest a roe for transition state stabilization as a aciity, or akainity can iffer markey from that of the sur- genera mechanism by which enzymes acceerate the rates of rouning cytopasm. chemica reactions. Knowege of the transition state of an enzyme-catayze reaction is frequenty expoite by chem- ists to esign an synthesize more effective enzyme inhibitors, ENZYMES EMPLOY MULTIPLE cae transition state analogs, as potentia pharmacophores. MECHANISTIC STRATEGIES TO FACILITATE CATALYSIS Covalent Catalysis The process of covalent catalysis invoves the formation of a Enzymes use combinations of four genera mechanistic strate- covaent bon between the enzyme an one or more substrates. gies to achieve ramatic enhancements of the rates of chemica The modified enzyme thus becomes a reactant. Covaent reactions. cataysis provies a new reaction pathway whose activation energy is ower—an rate of reaction therefore faster—than Catalysis by Proximity the pathways avaiabe in homogeneous soution. The chemi- In orer to chemicay interact, substrate moecues must come cay moifie state of the enzyme is, however, transient. within bon-forming istance of one another. The higher Competion of the reaction returns the enzyme to its origi- their concentration, the more frequenty they wi encounter na, unmoifie state. Its roe thus remains cataytic. Covaent one another, an the greater wi be the rate at which reaction cataysis is particuary common among enzymes that catayze proucts appear. When an enzyme bins substrate moecues group transfer reactions. Resiues on the enzyme that par- at its active site, it creates a region of high oca substrate con- ticipate in covaent cataysis generay are cysteine or serine, centration, one in which they are oriente in an iea position an occasionay histiine. Covaent cataysis often foows a to chemicay interact. In an of itsef, this proximity resuts “ping-pong” mechanism—one in which the first substrate is in rate enhancements of at east a 1000-fo over that observe in boun an its prouct reease prior to the bining of the the absence of an enzyme. secon substrate (Figure 7–4). Pyr Glu Ala CHO CH2NH2 KG CH2NH2 CHO E CHO E E E CH2NH2 E E E CHO Ala Pyr KG Glu FIGURE 7–4 “Ping-pong” mechanism for transamination. E—CHO and E—CH2NH2 represent the enzyme-pyridoxal phosphate and enzyme-pyridoxamine complexes, respectively. (Ala, alanine; Glu, glutamate; KG, α-ketoglutarate; Pyr, pyruvate.) CHAPTER 7 Enzymes: Mechanism of Action 63 O A B N.. C R H H.... O 1 H O O H O O C C A B CH2 CH2 Asp Y Asp X O N.. C R A B 2 H OH H H O O O O C C FIGURE 7–5 Two-dimensional representation of Koshland’s induced fit model of the active site of a lyase. Binding of the sub- CH2 CH2 strate A—B induces conformational changes in the enzyme that align Asp Y Asp X catalytic residues which participate in catalysis and strain the bond O between A and B, facilitating its cleavage. N H C R H HO SUBSTRATES INDUCE CONFORMATIONAL CHANGES 3 O O H O O IN ENZYMES C C Whie Fischer’s “ock an key moe” accounte for the exqui- CH2 CH2 site specificity of enzyme–substrate interactions, the impie Asp Y Asp X rigiity of the enzyme’s active site faie to account for the ynamic changes that accompany cataytic transformations. FIGURE 7–6 Mechanism for catalysis by an aspartic protease This rawback was aresse by Danie Koshan’s induced such as HIV protease. Curved arrows indicate directions of electron fit model, which states that as substrates bin to an enzyme, movement. ➀ Aspartate X acts as a base to activate a water molecule by abstracting a proton. ➁ The activated water molecule attacks they inuce a conformationa change that is anaogous to pac- the peptide bond, forming a transient tetrahedral intermediate. ing a han (substrate) into a gove (enzyme) (Figure 7–5). The ➂ Aspartate Y acts as an acid to facilitate breakdown of the tetra- enzyme in turn inuces reciproca changes in its substrates, hedral intermediate and release of the split products by donating a harnessing the energy of bining to faciitate the transfor- proton to the newly formed amino group. Subsequent shuttling of the mation of substrates into proucts. The inuce fit moe proton on Asp X to Asp Y restores the protease to its initial state. has been ampy confirme by biophysica stuies of enzyme motion uring substrate bining. then faciitates the ecomposition of this tetrahera inter- meiate by onating a proton to the amino group prouce by rupture of the peptie bon. The two active site aspartates HIV PROTEASE ILLUSTRATES can act simutaneousy as a genera base or as a genera aci ACID–BASE CATALYSIS because their immeiate environment favors ionization of one, but not the other. Enzymes of the aspartic protease family, which incues the igestive enzyme pepsin, the ysosoma cathepsins, an the protease prouce by the human immunoeficiency virus CHYMOTRYPSIN & FRUCTOSE-2, (HIV), share a common mechanism that empoys two con- serve asparty resiues as aci–base cataysts. In the first 6-BISPHOSPHATASE ILLUSTRATE stage of the reaction, one aspartate functions as a genera base COVALENT CATALYSIS (Asp X, Figure 7–6) that extracts a proton from a water mo- ecue to make it more nuceophiic. The resuting nuceophie Chymotrypsin then attacks the eectrophiic carbony carbon of the peptie Whie cataysis by aspartic proteases invoves the irect hyro- bon targete for hyroysis, forming a tetrahedral transition ytic attack of water on a peptie bon, cataysis by the ser- state intermediate. A secon aspartate (Asp Y, Figure 7–6) ine protease chymotrypsin invoves formation of a covaent 64 SECTION II Enzymes: Kinetics, Mechanism, Regulation, & Role of Transition Metals acy-enzyme intermeiate. A conserve sery resiue, serine H O 195 in the bovine form, is activate via interactions with his- R1 N C R2 tiine 57 an aspartate 102. Whie these three resiues are far apart in primary structure, in the active site of the mature, 1 O O H N N H O foe protein they resie within bon-forming istance of C Ser 195 one another. Aigne in the orer Asp 102-His 57-Ser 195, Asp 102 His 57 this trio forms a inke charge-relay network that acts as a “proton shuttle.” H O Bining of substrate initiates proton shifts that in R1 N C R2 effect transfer the hyroxy proton of Ser 195 to Asp 102 (Figure 7–7). The enhance nuceophiicity of the sery oxy- 2 O O H N N H O gen faciitates its attack on the carbony carbon of the peptie Ser 195 bon of the substrate, forming a covaent acyl-enzyme inter- Asp 102 His 57 mediate. The proton on Asp 102 then shuttes via His 57 to O the amino group iberate when the peptie bon is ceave. R1 NH2 C R2 The portion of the origina peptie with a free amino group then eaves the active site an is repace by a water moecue. 3 O O H N N O The charge-reay network now activates the water moecue by Ser 195 withrawing a proton through His 57 to Asp 102. The resut- Asp 102 His 57 ing hyroxie ion attacks the acy-enzyme intermeiate, an a O reverse proton shutte returns a proton to Ser 195, restoring its H C R2 origina state. Whie moifie uring the process of cataysis, O O chymotrypsin emerges unchange on competion of the reac- 4 O O H N N H Ser 195 tion. The proteases trypsin an eastase empoy a simiar cata- ytic mechanism, but the numbering of the resiues in their Asp 102 His 57 Ser-His-Asp proton shuttes iffer. O H Fructose-2,6-Bisphosphatase O C R2 Fructose-2,6-bisphosphatase, a reguatory enzyme of guco- 5 O O H N N H O neogenesis (see Chapter 19), catayzes the hyroytic reease Ser 195 of the phosphate on carbon 2 of fructose-2,6-bisphosphate. Asp 102 His 57 Figure 7–8 iustrates the roes of seven active site resiues. HOOC R2 Cataysis invoves a “cataytic tria” consisting of one Gu an two His resiues, of which one His forms a covaent phospho- 6 O O H N N H O histiy intermeiate. Ser 195 Asp 102 His 57 CATALYTIC RESIDUES ARE HIGHLY FIGURE 7–7 Catalysis by chymotrypsin. ➀ The charge-relay system removes a proton from Ser 195, making it a stronger nucleo- CONSERVED phile. ➁ Activated Ser 195 attacks the peptide bond, forming a Members of an enzyme famiy such as the aspartic or serine pro- transient tetrahedral intermediate. ➂ Release of the amino terminal teases empoy a simiar mechanism to catayze a common reac- peptide is facilitated by donation of a proton to the newly formed amino group by His 57 of the charge-relay system, yielding an acyl- tion type, but act on ifferent substrates. Most enzyme famiies Ser 195 intermediate. ➃ His 57 and Asp 102 collaborate to activate a appear to have arisen through gene upication events that cre- water molecule, which attacks the acyl-Ser 195, forming a second tet- ate a secon copy of the gene that encoes a particuar enzyme. rahedral intermediate. ➄ The charge-relay system donates a proton The two genes, an consequenty their encoe proteins, can to Ser 195, facilitating breakdown of the tetrahedral intermediate to then evove inepenenty, forming ivergent homologs that release the carboxyl terminal peptide ➅. recognize ifferent substrates. The resut is iustrate by chy- motrypsin, which ceaves peptie bons on the carboxy termi- ISOZYMES ARE DISTINCT ENZYME na sie of arge hyrophobic amino acis, an trypsin, which FORMS THAT CATALYZE THE ceaves peptie bons on the carboxy termina sie of basic amino acis. Proteins that iverge from a common ancestor are SAME REACTION sai to be homologous to one another. The common ancestry Higher organisms often eaborate severa physicay istinct of enzymes can be inferre from the presence of specific amino versions of a given enzyme, each of which catayzes the same acis in the same reative position in each famiy member. These reaction. These protein cataysts or isozymes can arise through resiues are sai to be evolutionarily conserved. gene upication or, in higher eukaryotes, aternative mRNA CHAPTER 7 Enzymes: Mechanism of Action 65 Assays of the cataytic activity of enzymes are frequenty use Lys 356 Lys 356 Arg Arg both in research an cinica aboratories. + 352 + 352 P + P + 6– 6– Single-Molecule Enzymology 2– 2– – O Arg 307 – O H+ Arg 307 The imite sensitivity of traitiona enzyme assays necessi- + Glu 327 + H P Glu 327 P tates the use of a arge group, or ensembe, of enzyme mo- + + His His ecues in orer to prouce measurabe quantities of prouct. 392 392 Arg 257 1 Arg 257 2 The ata obtaine thus refect the average activity of iniviua His 258 His 258 enzymes across mutipe cyces of cataysis. Recent avances in E Fru-2,6-P2 E-P Fru-6-P nanotechnology an imaging have mae it possibe to observe cataytic events invoving iscrete enzyme an substrate mo- Lys 356 Lys 356 ecues. Consequenty, scientists can now measure the rate of + Arg 352 + Arg 352 iniviua cataytic events, an sometimes a specific step in H + + cataysis, by a process cae single-molecule enzymology, an H O exampe of which is iustrate in Figure 7–9. Arg 307 Arg 307 – – + Pi + Glu 327 + H P + Glu 327 + + Drug Discovery Requires Enzyme His 392 His 392 Assays Suitable for High-Throughput 3 4 Arg 257 His 258 Arg 257 His 258 Screening E-P H2O E Pi Enzymes are frequent targets for the eveopment of rugs an other therapeutic agents. These generay take the form FIGURE 7–8 Catalysis by fructose-2,6-bisphosphatase. of enzyme inhibitors (see Chapter 8). The iscovery of new (1) Lys 356 and Arg 257, 307, and 352 stabilize the quadruple nega- tive charge of the substrate by charge–charge interactions. Glu 327 rugs is greaty faciitate when a arge number of potentia stabilizes the positive charge on His 392. (2) The nucleophile His 392 pharmacophores can be simutaneousy assaye in a rapi, attacks the C-2 phosphoryl group and transfers it to His 258, form- automate fashion—a process referre to as high-throughput ing a phosphoryl-enzyme intermediate. Fructose-6-phosphate now screening (HTS). HTS empoys robotics, optics, ata process- leaves the enzyme. (3) Nucleophilic attack by a water molecule, pos- ing, an microfuiics to simutaneousy conuct an monitor sibly assisted by Glu 327 acting as a base, forms inorganic phosphate. (4) Inorganic orthophosphate is released from Arg 257 and Arg 307. thousans of parae enzyme assays. It thus serves as a per- (Reproduced with permission from Pilkis SJ, Claus TH, Kurland IJ, et al: fect compement to combinatorial chemistry, a metho for 6-Phosphofructo-2-kinase/fructose-2,6-bisphosphatase: a metabolic signaling enzyme, Annu Rev Biochem. 1995;64:799-835.) spicing (see Chapter 36). Whie the homoogous proteases escribe act on ifferent substrates, isozymes aso may iffer in auxiiary features such as sensitivity to particuar reguatory factors (see Chapter 9) or subceuar ocation that aapt them to specific tissues or circumstances rather than istinct sub- strates. Isozymes that catayze the ientica reaction may aso enhance surviva by proviing a “backup” copy of an essentia enzyme. THE CATALYTIC ACTIVITY OF ENZYMES FACILITATES THEIR 1 2 3 4 DETECTION FIGURE 7–9 Direct observation of single DNA cleavage events catalyzed by a restriction endonuclease. DNA molecules The reativey sma quantities of enzymes typicay containe immobilized to beads (blue) are placed in a flowing stream of in ces hamper etermination of their presence an abun- buffer (black arrows), which causes them to assume an extended ance. However, the abiity to rapiy transform thousans of conformation. Cleavage at one of the restriction sites (orange) by an moecues of a specific substrate into proucts imbues each endonuclease leads to a shortening of the DNA molecule, which can enzyme with the abiity to ampify its presence. Uner appro- be observed directly in a microscope since the nucleotide bases in DNA are fluorescent. Although the endonuclease (red) does not fluo- priate conitions (see Chapter 8), the rate of the cataytic resce, and hence is invisible, the progressive manner in which the DNA reaction being monitore is proportionate to the amount of molecule is shortened (1→4) reveals that the endonuclease binds to enzyme present, which aows its concentration to be inferre. the free end of the DNA molecule and moves along it from site to site. 66 SECTION II Enzymes: Kinetics, Mechanism, Regulation, & Role of Transition Metals generating arge ibraries of chemica compouns spanning a prouce. Conversey, when a ehyrogenase catayzes the possibe combinations of a given set of chemica precursors. oxiation of NAD(P)H, a ecrease in absorbance at 340 nm wi be observe. In each case, the rate of change in absorbance Enzyme-Linked Immunoassays at 340 nm wi be proportionate to the quantity of the enzyme present. The sensitivity of enzyme assays can be expoite to etect The assay of enzymes whose reactions are not accompa- proteins that ack cataytic activity. Enzyme-linked immuno- nie by a change in absorbance or fuorescence is generay sorbent assays (ELISAs) use antiboies covaenty inke to more ifficut. In some instances, either the prouct or remain- a “reporter enzyme”, such as akaine phosphatase or horse- ing substrate can be transforme into a more reaiy etecte raish peroxiase, that can be use to prouce easiy-etecte compoun, athough the reaction prouct may have to be chromogenic or fuorescent proucts in vitro. Serum or other separate from unreacte substrate prior to measurement. bioogic sampes to be teste are first pace in mutiwe An aternative strategy is to evise a synthetic substrate whose microtiter pates. Most proteins ahere to the pastic surface prouct absorbs ight or fuoresces. For exampe, hyroysis of an thus are immobiize. Any expose pastic that remains is the phosphoester bon in p-nitropheny phosphate (pNPP), subsequenty “bocke” by aing a nonantigenic protein such an artificia substrate moecue, is catayze at a measurabe as bovine serum abumin. A soution of antiboy covaenty rate by numerous phosphatases, phosphoiesterases, an ser- inke to a reporter enzyme is then ae an the antiboies ine proteases. Whie pNPP oes not absorb visibe ight, the aowe to ahere to any immobiize antigen moecues. Excess anionic form of the p-nitropheno (pKa 6.7) generate on its free antiboy moecues are then remove by washing. The hyroysis strongy absorbs ight at 419 nm, an thus can be presence an quantity of boun antiboy is then etermine quantifie. by assaying the activity of the reporter enzyme, a quantity that shou be proportiona to the number of antiboy moecues present an, presumaby, the antigen moecues to which they Many Enzymes May Be Assayed by are boun. Coupling to a Dehydrogenase Another quite genera approach is to empoy a “coupe” assay NAD(P)+-Dependent Dehydrogenases (Figure 7–11). Typicay, a ehyrogenase whose substrate Are Assayed Spectrophotometrically is the prouct of the enzyme of interest is ae in cataytic excess. The rate of appearance or isappearance of NAD(P)H The physicochemica properties of the reactants in an then epens on the rate of the enzyme reaction to which the enzyme-catayze reaction ictate the options for the assay of ehyrogenase has been coupe. enzyme activity. Spectrophotometric assays expoit the abiity of a substrate or prouct to absorb ight. The reuce coen- zymes NADH an NADPH, written as NAD(P)H, absorb ight at a waveength of 340 nm, whereas their oxiize forms THE ANALYSIS OF CERTAIN NAD(P)+ o not (Figure 7–10). When NAD(P)+ is reuce, ENZYMES AIDS DIAGNOSIS the absorbance at 340 nm therefore increases in proportion The anaysis of enzymes in boo pasma pays a centra roe to—an at a rate etermine by—the quantity of NAD(P)H in the iagnosis of severa isease processes. Many enzymes are functiona constituents of boo. Exampes incue pseu- ochoinesterase, ipoprotein ipase, an components of the 1.0 Glucose 0.8 ATP, Mg2+ Hexokinase Optical density 0.6 ADP, Mg2+ Glucose-6-phosphate 0.4 NADP+ NADH Glucose-6-phosphate dehydrogenase 0.2 NADPH + H + 6-Phosphogluconolactone NAD+ 0 FIGURE 7–11 Coupled enzyme assay for hexokinase activity. 200 250 300 350 400 The production of glucose-6-phosphate by hexokinase is coupled to Wavelength (nm) the oxidation of this product by glucose-6-phosphate dehydrogenase in the presence of added enzyme and NADP+. When an excess of FIGURE 7–10 Absorption spectra of NAD+ and NADH. Densi- glucose-6-phosphate dehydrogenase is present, the rate of formation ties are for a 44-mg/L solution in a cell with a 1-cm light path. NADP+ of NADPH, which can be measured at 340 nm, is governed by the rate and NADPH have spectra analogous to NAD+ and NADH, respectively. of formation of glucose-6-phosphate by hexokinase. CHAPTER 7 Enzymes: Mechanism of Action 67 cascaes that trigger boo cotting, cot issoution, an opso- TABLE 7–1 Principal Serum Enzymes Used in Clinical nization of invaing microbes. Severa enzymes are reease Diagnosis into pasma foowing ce eath or injury. Whie these atter Serum Enzyme Major Diagnostic Use enzymes perform no physioogic function in pasma, they can serve as biomarkers, moecues whose appearance or eves Alanine aminotransferase (ALT) Viral hepatitis can assist in the iagnosis an prognosis of iseases an inju- Amylase Acute pancreatitis ries affecting specific tissues. The pasma concentration of an Ceruloplasmin Hepatolenticular degeneration enzyme or other protein reease consequent to injury may (Wilson disease) rise eary or ate, an may ecine rapiy or sowy. Cytopas- Creatine kinase Muscle disorders mic proteins ten to appear more rapiy than those from sub- ceuar organees. γ-Glutamyl transferase Various liver diseases Quantitative anaysis of the activity of reease enzymes or Lactate dehydrogenase Liver diseases other proteins, typicay in pasma or serum but aso in urine isozyme 5 or various ces, provies information concerning iagnosis, Lipase Acute pancreatitis prognosis, an response to treatment. Assays of enzyme activ- ity typicay empoy stanar kinetic assays of initia reaction β-Glucocerebrosidase Gaucher disease rates. Table 7–1 ists severa enzymes of vaue in cinica iag- Phosphatase, acid Prostate disease, including nosis. Note that these enzymes are not absoutey specific for cancer the inicate isease. For exampe, eevate boo eves of Phosphatase, alkaline Various bone disorders, prostatic aci phosphatase are associate typicay with pros- (isozymes) obstructive liver diseases tate cancer, but aso may occur with certain other cancers an ALT, alanine aminotransferase. noncancerous conitions. Interpretation of enzyme assay ata Note: Many of the above enzymes are not specific to the disease listed. must make ue aowance for the sensitivity an the iagnos- tic specificity of the enzyme test, together with other factors eicite through a comprehensive cinica examination that or ess of a preiminary iagnosis to permit initiation of appro- incues patient’s age, sex, prior history, an possibe rug use. priate therapy. The first enzymes use to iagnose MI were aspartate aminotransferase (AST), aanine aminotransferase (ALT), an actate ehyrogenase (LDH). Diagnosis using Analysis of Serum Enzymes Following LDH expoits the tissue-specific variations in its quaternary Tissue Injury structure (Figure 7–12). However, it is reease reativey An enzyme usefu for iagnostic enzymoogy shou be rea- sowy foowing injury. Creatine kinase (CK) has three tissue- tivey specific for the tissue or organ uner stuy, an shou specific isozymes: CK-MM (skeeta musce), CK-BB (brain), appear in the pasma or other fui at a time usefu for iag- an CK-MB (heart an skeeta musce), aong with a more nosis (the “iagnostic winow”). In the case of a myocaria optima iagnostic winow. As with LDH, iniviua CK infarction (MI), etection must be possibe within a few hours isozymes are separabe by eectrophoresis. Toay, assays of + – Lactate (Lactate) SH2 S (Pyruvate) dehydrogenase Heart A NAD+ NADH + H+ Normal B Reduced PMS Oxidized PMS Liver C Oxidized NBT Reduced NBT (colorless) (blue formazan) 5 4 3 2 1 FIGURE 7–12 Normal and pathologic patterns of lactate dehydrogenase (LDH) isozymes in human serum. Samples of serum were separated by electrophoresis. LDH isozymes were then visualized using a dye-coupled reaction-specific for LDH. Pattern A is serum from a patient with a myocardial infarct; B is normal serum; and C is serum from a patient with liver disease. Arabic numerals identify LDH isozymes 1 through 5. Electrophoresis and a specific detection technique thus can be used to visualize isozymes of enzymes other than LDH. 68 SECTION II Enzymes: Kinetics, Mechanism, Regulation, & Role of Transition Metals pasma CK eves are primariy use to assess skeeta musce bioogica “fingerprints” to hep trace the source of a sampe isorers such as Duchene muscuar ystrophy. of DNA to a specific iniviua. RFLP suffers from severa important imitations, the most critica of which was the Plasma Troponin Constitutes the requirement for reativey high quantities of DNA to empoy as substrates for the restriction enzymes. Toay, a ramaticay Currently Preferred Diagnostic Marker more sensitive set of moecuar iagnostic toos base on the for an MI polymerase chain reaction (PCR) have argey suppante Troponin is a compex of three proteins present in the contrac- RFLP anaysis. tie apparatus of skeletal an cardiac muscle but not in smooth muscle (see Chapter 51). Troponin eves in pasma typicay Medical Applications of the Polymerase rise for 2 to 6 hours after an MI, an remain eevate for 4 to 10 ays. Immunoogica measurements of pasma eves of Chain Reaction cariac troponins I an T thus provie sensitive an specific As escribe in Chapter 39, the poymerase chain reaction inicators of amage to heart musce. Since other sources of (PCR) empoys a thermostabe DNA poymerase an appro- heart musce amage aso eevate serum troponin eves, car- priate oigonuceotie primers to prouce thousans of copies iac troponins provie a genera marker of cariac injury. of a efine segment of DNA from a minute quantity of start- ing materia. PCR enabes meica, bioogica, an forensic scientists to etect an characterize DNA present initiay at Additional Clinical Uses of Enzymes eves too ow for irect etection. In aition to screening for Enzymes are empoye in the cinica aboratory to etermine genetic mutations, PCR can be use to etect pathogens an the presence an the concentration of critica metaboites. For parasites such as Trypanosoma cruzi, the causative agent of exampe, gucose oxiase frequenty is utiize to measure Chagas isease, an Neisseria meningitides, the causative agent pasma gucose concentration. Enzymes aso are empoye of bacteria meningitis, through the seective ampification of with increasing frequency for the treatment of injury an their DNA. isease. Exampes incue tissue pasminogen activator (tPA) or streptokinase for treatment of acute MI, an trypsin for treat- ment of cystic fibrosis. Intravenous infusion of recombinanty RECOMBINANT DNA PROVIDES prouce gycosyases can be use to treat ysosoma storage synromes such as Gaucher isease (β-gucosiase), Pompe AN IMPORTANT TOOL FOR isease (α-gucosiase), Fabry isease (α-gaactosiase A), Sy STUDYING ENZYMES isease (β-gucuroniase), an mucopoysacchariosis types I, Highy purifie sampes of enzymes are essentia for the stuy II, an VI—aso known as Hurer synrome (α-L-iuroniase), of their structure an function. The isoation of an inivi- Hunter synrome (iuronate 2-sufatase), an Maroteaux-Lamy ua enzyme, particuary one present in ow concentration, synrome (arysufatase B), respectivey. from among the thousans of proteins present in a ce can be extremey ifficut. By coning the gene for the enzyme of interest, it generay is possibe to prouce arge quantities of ENZYMES FACILITATE DIAGNOSIS its encoe protein in Escherichia coli or yeast. However, not OF GENETIC & INFECTIOUS a anima proteins can be expresse in their appropriatey foe, functionay competent form in microbia ces as these DISEASES organisms cannot perform certain posttransationa process- Many iagnostic techniques take avantage of the specific- ing tasks specific to higher organisms. In these instances, ity an efficiency of the enzymes that act on oigonuceoties options incue expression of recombinant genes in cuture such as DNA. Eary on restriction endonucleases emerge anima ce systems or by empoying the bacuovirus expres- as an important too for both cinica an forensic anayses. sion vector of cuture insect ces. For more etais concern- Restriction enonuceases, often referre to as restriction ing recombinant DNA techniques, see Chapter 39. enzymes, ceave oube-strane DNA at sites specifie by a sequence of four, six, or more base pairs cae restriction sites (see Chapter 39). If an iniviua bears a mutation or polymor- Recombinant Fusion Proteins Are phism that either eiminates or generates the restriction site Purified by Affinity Chromatography for one of the enonuceases being use, the number an size Recombinant DNA technoogy can aso be use to generate of the DNA fragments prouce on ceavage wi iffer from proteins specificay moifie to rener them reaiy purifie that of another iniviua. Such restriction fragment length by affinity chromatography. The gene of interest is inke to polymorphisms (RFLPs) can be use for prenata etection an aitiona oigonuceotie sequence that encoes a car- of eeterious mutations characteristic of hereitary isorers boxy or amino termina extension to the protein of interest. such as sicke ce trait, β-thaassemia, infant phenyketonuria, The resuting fusion protein contains a new omain taiore an Huntington isease. They aso can be use as moecuar to interact with an appropriatey moifie affinity support. CHAPTER 7 Enzymes: Mechanism of Action 69 GST T Enzyme an cataysis. For exampe, the inference that a particuar ami- noacy resiue functions as a genera aci can be teste by Plasmid encoding GST Cloned DNA repacing it with an aminoacy resiue incapabe of onating with thrombin site (T) encoding enzyme a proton. Ligate together RIBOZYMES: ARTIFACTS FROM THE RNA WORLD GST T Enzyme Cech Discovered the First Catalytic Transfect cells, add RNA Molecule inducing agent, then For many years after their iscovery it was assume that a break cells enzymes were proteins. However, whie examining the pro- Apply to glutathione (GSH) cessing of ribosoma RNA (rRNA) moecues in the ciiate affinity column protozoan Tetrahymena in the eary 1980s, Thomas Cech an his coworkers observe that processing of the 26S rRNA pro- Sepharose GSH GST bead T Enzyme ceee smoothy in vitro even in the tota absence of protein. They subsequenty trace the source of this spicing activity Elute with GSH, to a 413 bp cataytic segment of the RNA, which they terme treat with thrombin a ribozyme (see Chapter 36)—a iscovery that earne Cech a Nobe Prize. GSH GST T Enzyme Severa other ribozymes have since been iscovere. The vast majority catayze nuceophiic ispacement reactions FIGURE 7–13 Use of glutathione S-transferase (GST) fusion that target the phosphoiester bons of the RNA backbone. proteins to purify recombinant proteins. (GSH, glutathione.) In sma sef-ceaving RNAs, such as hammerhea or hepatitis eta virus RNA, the attacking nuceophie is water an the One popuar approach is to attach an oigonuceotie that resut is hyroysis. For the arge group I intron ribozymes, encoes six consecutive histiine resiues. The expresse “His the attacking nuceophie is the 3′-hyroxy of the termina tag” protein bins to chromatographic supports that contain ribose of another segment of RNA an the resut is a spicing an immobiize ivaent meta ion such as Ni2+ or C2+. This reaction. approach expoits the abiity of these ivaent cations to bin His resiues. Once boun, contaminating proteins are washe The Ribosome—The Ultimate off an the His-tagge enzyme is eute with buffers contain- Ribozyme ing high concentrations of free histiine or imiazoe, which compete with the poyhistiine tais for bining to the immo- The ribosome was the first exampe of a “moecuar machine” biize meta ions. Aternativey, the substrate-bining omain to be recognize. A massive compex comprise of scores of of gutathione S-transferase (GST) can serve as a “GST tag.” protein subunits an severa arge ribosoma RNA moecues, Figure 7–13 iustrates the purification of a GST-fusion pro- the ribosome performs the vitay important an highy com- tein using an affinity support containing boun gutathione. pex process of synthesizing ong poypeptie chains foowing The aition of an N-termina fusion omain may aso the instructions encoe in messenger RNA (mRNA) mo- hep inuce proper foing of the remainer of the recombi- ecues (see Chapter 37). For many years, it was assume that nant poypeptie. Most fusion omains aso possess a ceav- rRNAs paye a passive, structura roe, or perhaps assiste age site for a highy specific protease such as thrombin in the in the recognition of cognate mRNAs through a base pair- region that inks the two portions of the protein to permit its ing mechanism. It was thus somewhat surprising when it was eventua remova. iscovere that rRNAs were both necessary an sufficient for catayzing peptie synthesis. Site-Directed Mutagenesis Provides The RNA World Hypothesis Mechanistic Insights The iscovery of ribozymes has ha a profoun infuence on Once the abiity to express a protein from its cone gene has evoutionary theory. For many years, scientists ha hypothe- been estabishe, it is possibe to empoy site-directed muta- size that the first bioogic cataysts were forme when amino genesis to change specific aminoacy resiues by atering their acis containe in the primoria soup coaesce to form sim- coons. Use in combination with kinetic anayses an x-ray pe proteins. With the reaization that RNA cou both carry crystaography, this approach faciitates ientification of the information an catayze chemica reactions, a new “RNA specific roes of given aminoacy resiues in substrate bining Wor” hypothesis emerge in which RNA constitute the 70 SECTION II Enzymes: Kinetics, Mechanism, Regulation, & Role of Transition Metals first bioogic macromoecue. Eventuay, a more chemicay Many enzymes can be assaye spectrophotometricay by stabe oigonuceotie, DNA, supersee RNA for ong-term couping them to an NAD(P)H-epenent ehyrogenase. information storage, whie proteins, by virtue of their greater Assay of pasma proteins, incuing severa enzymes, ais chemica functiona group an conformationa iversity cinica iagnosis an prognosis. ominate cataysis. If one assumes that some sort of RNA- Restriction enonuceases faciitate iagnosis of genetic iseases protein hybri was forme as an intermeiate in the transi- by reveaing restriction fragment ength poymorphisms. tion from ribonuceotie to poypeptie cataysts, one nees The PCR ampifies DNA initiay present in quantities too to ook no further than the ribosome to fin the presume sma for anaysis. missing ink. Attachment of a poyhistiy, GST, or other “tag” to the N- or Why i not proteins take over a cataytic functions? C-terminus of a recombinant protein faciitates its purification Presumaby, in the case of the ribosome the process was both by affinity chromatography. too compex an too essentia to permit much opportunity for Not a enzymes are proteins. Severa ribozymes are known that possibe competitors to gain a footho. In the case of the sma can cut an respice the phosphoiester bons of RNA whie sef-ceaving RNAs an sef-spicing introns, they may repre- it is the RNA components of the ribosome that are primariy sent one of the few cases in which RNA autocataysis is more responsibe for catayzing poypeptie synthesis. efficient than eveopment of a new protein catayst. REFERENCES SUMMARY Appe FS, Sanova Y, Jaffe AS, et a: Cariac troponin assays: Guie Enzymes are efficient cataysts whose stringent specificity to unerstaning anaytica characteristics an their impact on extens to the kin of reaction catayze, an typicay, to the cinica care. Cin Chem 2017;63:73. stereochemistry of their substrates. Baumer ZT, Whitehea TA: The inner workings of an enzyme: A high-throughput mutation screen issects the mechanistic basis In many enzymes, the suite of chemica toos avaiabe to of enzyme activity. Science 2021;373:391. faciitate cataysis has been expane using non-amino aci Bishop ML, Foy EP, Schoeff, LE: Clinical Chemistry. Principles, moecues. When tighty boun, these inorganic an organic Techniques, and Correlations, 8th e. Jones & Bartett Learning, 2018. moecues are referre to as prosthetic groups. If oosey boun Frey PA, Hegeman AD: Enzyme Reaction Mechanisms. Oxfor (issociabe), they are referre to as cofactors. University Press, 2006. Coenzymes, many of which are erivatives of B vitamins, Heckman CM, Paraisi F: Looking back: A short history of the serve as “shuttes” for commony use groups such as amines, iscovery of enzymes an how they became powerfu chemica eectrons, an acety groups. too. Chem Cat Chem 2020;12:6082. During cataysis, enzymes reirect the conformationa changes Hestrom L: Serine protease mechanism an specificity. Chem Rev inuce by substrate bining to effect compementary changes 2002;102:4501. that faciitate transformation into prouct. Knight AE: Singe enzyme stuies: A historica perspective. Meth Mechanistic strategies empoye by enzymes to faciitate Mo Bio 2011;778:1. cataysis incue the introuction of strain, approximation of Rho JH, Lampe PD: High–throughput anaysis of pasma hybri reactants, aci–base cataysis, an covaent cataysis. markers for eary etection of cancers. Proteomes 2014;2:1. Sanabria H, Ronin D, Hemmen K, et a: Resoving ynamics an Aminoacy resiues that participate in cataysis are highy function of transient states in singe enzyme moecues. Nature conserve through the evoution of enzymes. Commun 2020;11:1231. The abiity to change resiues suspecte of being important Spies M, Chema Y (es): Single-Molecule Enzymology: Fluorescence- in cataysis or substrate bining by site-irecte mutagenesis based and High-throughput Methods. Methos Enzymo, vo. 581, provies important insights into mechanisms of enzyme action. Acaemic Press, 2016 (Entire voume). The cataytic activity of enzymes reveas their presence, Weinberg CA, Weinberg Z, Hammann C: Nove ribozymes: faciitates their etection, an provies the basis for enzyme- Discovery, cataytic mechanisms, an the quest to unerstan inke immunoassays. bioogica function. Nuc Acis Res 2019;47:9480.