Visual Perception PDF
Document Details
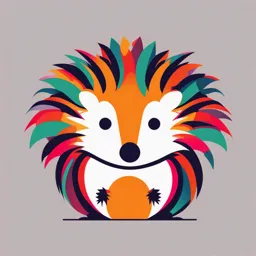
Uploaded by PreeminentSatellite6455
Ifugao State University
Tags
Summary
This document is a textbook chapter on visual perception, going over various theories, approaches to perception, how perception is done and the important factors that shape our perception of the world, and lastly the importance of certain anomalies to understand the process better.
Full Transcript
Visual Perception From Sensation to Perception Some Basic Concepts of Perception...
Visual Perception From Sensation to Perception Some Basic Concepts of Perception 3 Seeing Things That Aren’t There, or Are They? How Does Our Visual System Work? Pathways to Perceive the What and the Where O U T L I N E Approaches to Perception: How Do We Make Sense of What We See? Bottom-Up Theories Direct Perception Template Theories Feature-Matching Theories Recognition-by-Components Theory Top-Down Theories How Do Bottom-Up Theories and Top-Down Theories Go Together? Perception of Objects and Forms Viewer-Centered versus Object-Centered Perception The Perception of Groups—Gestalt Laws Recognizing Patterns and Faces Two Different Pattern Recognition Systems The Neuroscience of Recognizing Faces and Patterns C H A P T E R The Environment Helps You See Perceptual Constancies Depth Perception Depth Cues The Neuroscience of Depth Perception Deficits in Perception Agnosias and Ataxias Difficulties Perceiving the “What” Difficulties in Knowing the “How” Are Perceptual Processes Independent of Each Other? Anomalies in Color Perception Why Does It Matter? Perception in Practice Key Themes Summary Thinking about Thinking: Analytical, Creative, and Practical Questions Key Terms Media Resources CogLab The CogLab icon directs you to concept-related experiments and labs you can explore. Each CogLab reference includes the Lab title and number for easy access. Go to CogLab.Cengage.com. 71 Copyright 2017 Cengage Learning. All Rights Reserved. May not be copied, scanned, or duplicated, in whole or in part. Due to electronic rights, some third party content may be suppressed from the eBook and/or eChapter(s). Editorial review has deemed that any suppressed content does not materially affect the overall learning experience. Cengage Learning reserves the right to remove additional content at any time if subsequent rights restrictions require it. 72 CHAPTER 3 Visual Perception Here are some of the questions we will explore in this chapter: 1. How can we perceive an object, such as a chair, as having a stable form given that the image of the chair on our retina changes as we look at it from different directions? 2. What are two fundamental approaches to explaining perception? 3. What happens when people with normal visual sensations cannot perceive visual stimuli? If You Encountered Tyrannosaurus Rex, Would B E LIE VE I T O R N O T Standing Still Save You? Have you seen the movie Jurassic Park? In this (for comparison, eagles can see only 3.6 times bet- movie, one protagonist tells another while facing a ter than humans). Its excellent vision is due to the Tyrannosaurus rex that they will be safe as long as big binocular range, which is the area that can be they don’t move because the T. rex can detect its seen by both eyes at the same time. In addition, prey only when it is moving. Well, he could not have over time, T. rex’s snout became longer, its cheeks been more wrong. As it now turns out, T. rex had grew thinner so as not to obstruct the view, and its excellent binocular vision (i.e., the vision fields of eyeballs became bigger. These changes all helped both eyes are combined to achieve depth percep- T. rex to have excellent three-dimensional (3-D) tion). Researchers had the heads of several dino- vision (Jaffe, 2006; Stevens, 2006). This chapter will saur species reconstructed and found that T. rex introduce you to the basics of visual perception for probably could see 13 times better than humans humans—and sometimes for other species as well. As we are writing this chapter, we can look out of the window onto the city of Boston. The high-rise buildings that are less than a mile away look about as small as our com- puter screen. Yet we know that they are actually much bigger than our screen. They only appear to be small. Try it out yourself. Look out of your window. Can you see how things that are farther away seem much smaller than you know they are? This is just one example of the complex process of perception. Have you ever been told that you can’t see something that’s right under your nose? How about that you can’t see the forest for the trees? Have you ever listened to your favorite song over and over, trying to decipher the lyrics? In each of these situations, we call on the complex construct of perception. Perception is the set of processes by which we recognize, organize, and make sense of the sensations we receive from environmental stimuli (Goo- dale, 2000a, 2000b; Kosslyn & Osherson, 1995; Marr, 1982; Pomerantz, 2003). When you look at an image in this book, sensory receptors in the retina are being stimulated and your sensation is transmitted to the brain through nerve impulses. Your brain interprets and organizes these sensations and turns them into a perception of a coherent image. Percep- tion encompasses many psychological phenomena. In this chapter, we focus primarily on visual perception. It is the most widely recognized and the most widely studied perceptual modality (i.e., system for a particular sense, such as touch or smell). First, we will get to know a few basic terms and concepts of perception. We will then consider optical illusions that illustrate some of the intricacies of human perception. Next, we examine the biology 72 of the visual system. We will consider some approaches to explain perception and, after- ward, have a closer look at some details of the perceptual process, namely the perception of Copyright 2017 Cengage Learning. All Rights Reserved. May not be copied, scanned, or duplicated, in whole or in part. Due to electronic rights, some third party content may be suppressed from the eBook and/or eChapter(s). Editorial review has deemed that any suppressed content does not materially affect the overall learning experience. Cengage Learning reserves the right to remove additional content at any time if subsequent rights restrictions require it. Visual Perception CHAPTER 3 73 objects and forms, and how the environment provides cues to help you perceive your sur- roundings. We will also explore what happens when people have difficulties in perception. I N V E S T I G AT I N G COGNITIVE PSYCHOLOGY Perception Stand at one end of a room and hold your thumb up to that the door is farther away from you than your thumb your eye so that it is the same size as the door on the is. In your mind, you make the door much larger to com- opposite side of the room. Do you really think that your pensate for the distance away from you. Knowledge is thumb is as large as a door? No. You know that your a key to perception. You know that your thumb and the thumb is close to you, so it just looks as large as the door are not the same size, so you are able to use this door. There are numerous cues in the room to tell you knowledge to correct for what you know is not so. From Sensation to Perception We do not perceive the world exactly as our eyes see it. Instead, our brain actively tries to make sense of the many stimuli that enter our eyes and fall on our retina. Take a look at Figure 3.1. You can see two high-rise buildings in the city of Boston. (We live in one of them!) In the right photo, the right tower seems to be substantially higher than the Courtesy of the author (a) (b) Figure 3.1 Objects Look Different Depending on the Perspective. The pictures show the same two high-rise buildings in Boston from two different perspectives. In (a) they look about the same size, as they in fact are. In (b), their image on the retina makes them seem to be of different heights, and it is only through further processing that we can pinpoint they are the same size. Copyright 2017 Cengage Learning. All Rights Reserved. May not be copied, scanned, or duplicated, in whole or in part. Due to electronic rights, some third party content may be suppressed from the eBook and/or eChapter(s). Editorial review has deemed that any suppressed content does not materially affect the overall learning experience. Cengage Learning reserves the right to remove additional content at any time if subsequent rights restrictions require it. 74 CHAPTER 3 Visual Perception Courtesy of the author Figure 3.2 Reality or Reflection? This picture shows the reflection of a church in a skyscraper. What is easy for us to perceive constitutes a big problem for computers. Where does one building end and the next one start? Which part of the percept belongs to which object? What distinguishes the real person on the street from his or her reflection in the building so that a computer can recognize which one is the reflection? left one. The left picture, however, shows that the towers actually are in fact exactly the same height. Depending on your viewpoint, objects can look different, revealing differ- ent details. Thus, perception does not consist of just seeing what is being projected onto your retina; the process is much more complex. Your brain processes the visual stimuli, giving the stimuli meaning and interpreting them. In recent years, researchers have tried to teach computers to “see.” Although computers still lag behind humans in object recognition, the research showed how difficult it is to interpret visual stimuli, or what we see. Can you recognize what is shown in Figure 3.2 ? The picture shows the reflection of a church in a high-rise building. It might have taken you a few moments to figure out what is in the photo, but for computers, this is an extremely difficult task. It is not immediately clear in this picture what is reflection, what is the building, and what is surrounding. Fur- thermore, the borders of the church are blurred so that it becomes challenging to see where the object ends and what it really is. Although it may not take you a lot of effort to identify the objects in this photo, it takes a lot of processing to perceive them, as the stimuli are ambiguous. This section focuses on the processes of visual perception and the processes we use to make sense of the visual stimuli that are focused on our retina. We start our explora- tion by familiarizing ourselves with some basic concepts. To illustrate the intricacies of perception, we then look at some optical illusions. And finally we learn how the eye receives impressions of stimuli and sends signals to the brain. Copyright 2017 Cengage Learning. All Rights Reserved. May not be copied, scanned, or duplicated, in whole or in part. Due to electronic rights, some third party content may be suppressed from the eBook and/or eChapter(s). Editorial review has deemed that any suppressed content does not materially affect the overall learning experience. Cengage Learning reserves the right to remove additional content at any time if subsequent rights restrictions require it. Visual Perception CHAPTER 3 75 Some Basic Concepts of Perception James Gibson (1966, 1979) provided a useful framework for studying perception in his influential work. He introduced the concepts of distal (external) object, informational medium, proximal stimulation, and perceptual object. Let’s examine each of these. The distal (far) object is the object in the external world (e.g., a falling tree). A tree falling creates a pattern on an informational medium. The informational medium could be sound waves, as in the sound of the falling tree or reflected light, chemical molecules, or tactile information coming from the environment. For example, when the informa- tion from light waves comes into contact with the appropriate sensory receptors of the eyes, proximal (near) stimulation occurs (i.e., the cells in your retina absorb the light waves). Perception occurs when a perceptual object (i.e., what you see) is created in you that reflects the properties of the external world. That is, an image of a falling tree is cre- ated on your retina that reflects the falling tree that is in front of you. Table 3.1 lists the various properties of distal objects, informational media, proxi- mal stimuli, and perceptual objects for five different senses (sight, sound, smell, taste, and touch). The processes of perception vary tremendously across the different senses. So, if a tree falls in the forest and no one is around to hear it, does it make a sound? It makes no perceived sound. But it does make a sound by creating sound waves. So the answer is yes or no, depending on how you look at the question. You would answer yes if Ta b l e 3. 1 From Sensation to Perception Perception occurs when the informational medium carries information about a distal object to a person. When the person’s sense receptors pick up on the information, proximal stimulation occurs and the person perceives an object. Modality Distal Object Informational Medium Proximal Stimulation Perceptual Object Vision—sight Grandma’s face Reflected light from Grand- Photon absorption in the Grandma’s face ma’s face (visible electro- rod and cone cells of magnetic waves) the retina, the receptor surface in the back of the eye Audition—sound A falling tree Sound waves generated by Sound-wave conduction A falling tree the tree’s fall to the basilar membrane, the receptor surface within the cochlea of the inner ear Olfaction—smell Bacon being Molecules released by frying Molecular absorption in Bacon fried bacon the cells of the olfactory epithelium, the recep- tor surface in the nasal cavity Gustation—taste Ice cream Molecules of ice cream both Molecular contact with Ice cream released into the air and dis- taste buds, the receptor solved in water cells on the tongue and soft palate, combined with olfactory stimulation Touch A computer Mechanical pressure and Stimulation of various Computer keys keyboard vibration at the point of con- receptor cells within the tact between the surface of dermis, the innermost the skin and the keyboard layer of skin Copyright 2017 Cengage Learning. All Rights Reserved. May not be copied, scanned, or duplicated, in whole or in part. Due to electronic rights, some third party content may be suppressed from the eBook and/or eChapter(s). Editorial review has deemed that any suppressed content does not materially affect the overall learning experience. Cengage Learning reserves the right to remove additional content at any time if subsequent rights restrictions require it. 76 CHAPTER 3 Visual Perception you believe that the existence of sound waves is all that’s needed to confirm the existence of a sound, but you would answer no if you believe the sound needs to be perceived (for the sound waves to have landed on the receptors in someone’s ears). Where does perception end and cognition begin? Furthermore, where does sen- sation end and perception begin? Answers to both questions are debatable. Instead we should view these processes as part of a continuum. Information flows through the system. Different processes address different questions. Questions of sensation focus on qualities of stimulation. Is that shade of red brighter than the red of a Red Delicious apple? Is the sound of that falling tree louder than the sound of thunder? How well do your impressions of color or sound match your friends’ impressions of the same? Perception questions typically relate to identity and form, pattern, and movement. Is that red thing an apple? Did I just hear a tree falling? Cognition occurs when this information is used to determine further goals. Is that apple edible? Should I get out of this forest? We can never see, hear, taste, smell, or feel the exact same set of stimulus properties we experienced before. Every apple casts a somewhat different image on our retina, no falling tree sounds exactly like another, and even the faces of our relatives and friends look different, depending on whether they are smiling, enraged, or sad. And a person’s voice sounds somewhat different, depending on whether he or she is sick, out of breath, tired, happy, or sad. Therefore, one fundamental question for perception is “How do we achieve perceptual stability when there is so much instability at the level of sensory receptors?” Given the nature of our sensory receptors, variation is necessary for percep- tion! In sensory adaptation, receptor cells adapt to constant stimulation by not firing until there is a change in stimulation. Through sensory adaptation, we may stop detect- ing the presence of a stimulus. To study visual perception, scientists devised a way to create stabilized images. Sta- bilized images do not move across the retina because they follow the eye movements. The use of this technique confirmed the hypothesis that constant stimulation of the cells of the retina gives the impression that the image disappears (Ditchburn, 1980; Martinez- Conde, Macknik, & Hybel, 2004; Riggs et al., 1953). When your eyes are exposed to a uniform field of stimulation (e.g., a red surface area without any shades, a clear blue sky, or dense fog), you will stop perceiving that stimulus after a few minutes and see just a gray field instead. This is because your eyes have adapted to the stimulus. Such a uniform visual field is called Ganzfeld. Ganzfeld is German and means “complete field” (Metzger, 1930). I N V E S T I G AT I N G COGNITIVE PSYCHOLOGY The Ganzfeld Effect Cut a Ping-Pong ball in two halves or use two plastic because your cells have adapted to the constant spoons. Paint them red, making sure there are no stimulus (the red of the ball halves or spoons). This streaks to ensure one uniform field of color. Put the is a Ganzfeld. Some people may perceive hallucina- ball halves or the spoons over your eyes so that your tions and experience altered states of consciousness eyes are completely covered. Then gaze toward a when exposed to a Ganzfeld (Wackermann, Puetz, & light source for a few minutes. At some point, your Allefeld, 2008). perception will change from the color red to gray Copyright 2017 Cengage Learning. All Rights Reserved. May not be copied, scanned, or duplicated, in whole or in part. Due to electronic rights, some third party content may be suppressed from the eBook and/or eChapter(s). Editorial review has deemed that any suppressed content does not materially affect the overall learning experience. Cengage Learning reserves the right to remove additional content at any time if subsequent rights restrictions require it. Visual Perception CHAPTER 3 77 Sensory adaptation ensures that sensory information is changing constantly. Because of the dulling effect of sensory adaptation in the retina (the receptor surface of the eye), our eyes constantly are making tiny rapid movements. These movements create constant changes in the location of the projected image inside the eye. Thus, stimulus variation is an essential attribute for perception. It paradoxically makes the task of explaining percep- tion more difficult. Sensory adaptation also plays a role in other senses. Have you ever been to a hotel room that strongly smelled like smoke when you entered first? After a while, you adapt to the smell and do not notice it anymore, or at least not as much. Seeing Things That Aren’t There, Figure 3.3 Dallenbach’s Cow. What do you learn about your own perception by trying to identify or Are They? the object staring at you from this photo? To expand our knowledge of perception, psychologists often Source: From Dallenbach, K. M. (1951). A puzzle-picture with a new principle of concealment. American Journal of Psychology, study situations that pose problems in making sense of our 54, 431–433. sensations. Consider the image displayed in Figure 3.3. To most people, the figure initially looks like a blur of meaning- less shadings. A recognizable creature is staring them in the face, but they may not see it. When people finally realize what is in the figure, they rightfully feel “cowed.” The figure of the cow is hidden within the continuous gradations of shading that constitute the picture. Before you recognized the figure as a cow, you correctly sensed all aspects of the figure. But you had not yet organized those sensations to form a mental percept—a mental representation of a stimulus that is perceived. Without such a percept of the cow, you could not meaningfully grasp what you pre- viously had sensed. The preceding examples show that sometimes we cannot perceive things that exist. At other times, however, we perceive things that do not exist. For example, notice the blue triangle in the center of the left panel of Figure 3.4. Also note the white triangle in the center of the right panel of Figure 3.4. They jump right out at you. Now look closely at each of the panels. You will see that the triangles are not really all there. The blue that constitutes the center triangle in the left panel looks darker, or more blue, than the surrounding blue. But it is not. Nor is the white central triangle in the right panel any brighter, or whiter, than the surrounding white. Both central triangles are optical illusions. They involve the perception of visual information not physically present in the visual sensory stimulus. So, sometimes we perceive what is not there. Other times, we do not perceive what is there. And at still other times, we perceive what cannot be there. The existence of perceptual illusions suggests that what we sense (in our sensory organs) is not necessar- ily what we perceive (in our minds). Our minds must take the available sensory information and manipulate that information to create mental representations of objects, properties, and spatial relationships within our environments (Peterson, 1999). The way we represent Figure 3.4 Elusive Triangles: Real or Illusions? You easily can see the triangles in this figure—or are the these objects will depend in part on our viewpoint in triangles just an illusion? perceiving the objects (Edelman & Weinshall, 1991; Pog- Source: Adapted from In Search of the Human Mind by Robert J. gio & Edelman, 1990; Tarr, 1995; Tarr & Bülthoff, 1998). Sternberg, by Harcourt Brace & Company. Copyright 2017 Cengage Learning. All Rights Reserved. May not be copied, scanned, or duplicated, in whole or in part. Due to electronic rights, some third party content may be suppressed from the eBook and/or eChapter(s). Editorial review has deemed that any suppressed content does not materially affect the overall learning experience. Cengage Learning reserves the right to remove additional content at any time if subsequent rights restrictions require it. 78 CHAPTER 3 Visual Perception (a) (b) Figure 3.5 The Parthenon. The columns of the Parthenon in Greece actually bulge slightly in the middle (b) to compensate for the visual tendency to perceive that straight parallel lines (a) seem to curve inward. Similarly, the horizontal lines of the beams crossing the top of the columns and the top step of the porch bulge slightly upward to counteract the tendency to perceive that they curve slightly downward. In addition, the columns lean ever so slightly inward at the top to compensate for the tendency to perceive them as spreading out as we gaze upward at them. Architects consider these distortions of visual perception in their designs today. An example in architecture is the use of optical illusions in the construction of the Par- thenon (Figure 3.5 ). Were the Parthenon actually constructed the way it appears to us perceptually (with strictly rectilinear form), its appearance would be bizarre. Architects are not the only ones to recognize some fundamental principles of per- ception. For centuries, artists have known how to lead us to perceive 3-D percepts when viewing two-dimensional (2-D) images. What are some of the principles that guide our perceptions of both real and illusory percepts? We will explore the answer to this ques- tion as we move through the chapter. We begin with examining our visual system. How Does Our Visual System Work? The precondition for vision is the existence of light. Light is electromagnetic radiation that can be described in terms of wavelength. Humans can perceive only a small range of the wavelengths that exist; the visible wavelengths are from 380 to 750 nanometers (Figure 3.6 ; Starr, Evers, & Starr, 2007). Vision begins when light passes through the protective covering of the eye (Figure 3.7 ). This covering, the cornea, is a clear dome that protects the eye. The light then passes through the pupil, the opening in the center of the iris. It continues through the crystalline lens and the vitreous humor. The vitreous humor is a gel-like substance that makes up the majority of the eye. Eventually, the light focuses on the retina where electromagnetic light energy is transduced—that is, converted—into neural electrochemical impulses (Blake, 2000). Vision is most acute in the fovea, which is a small, thin region of the retina, the size of the head of a pin. When you look straight at an object, your eyes rotate so that the image Copyright 2017 Cengage Learning. All Rights Reserved. May not be copied, scanned, or duplicated, in whole or in part. Due to electronic rights, some third party content may be suppressed from the eBook and/or eChapter(s). Editorial review has deemed that any suppressed content does not materially affect the overall learning experience. Cengage Learning reserves the right to remove additional content at any time if subsequent rights restrictions require it. Visual Perception CHAPTER 3 79 Increasing energy Increasing wavelength 350 500 600 700 Violet Green Yellow Red Ultra X- Infrared AC Gamma rays violet Radar FM TV AM ray rays circuits rays 10–3 10–1 101 103 105 107 109 1011 1013 1015 Wavelength (nm) Figure 3.6 The Electromagnetic Spectrum. This image shows the different wavelengths that light comes in, and the small array of wavelengths that is actually visible to humans. Source: Based on Kalat, Biological Psychology, 11e Figure 6.8. falls directly onto the fovea. The retina contains the photoreceptors, which convert light energy into electrochemical energy that is transmitted by neurons to the brain. There are two kinds of photoreceptors—rods and cones. Each eye contains roughly 120 million rods and 8 million cones. Rods and cones differ not only in shape but also in their com- positions, locations, and responses to light. Within the rods and cones are photo pig- ments, chemical substances that react to light and transform physical electromagnetic energy into an electrochemical neural impulse that can be understood by the brain. The rods are long and thin photoreceptors. They are more highly concentrated in the periph- ery of the retina than in the foveal region. The rods are responsible for night vision and Rods and cones Iris (colored area) Fovea Pupil Blind spot Cornea Lens Ciliary muscle (controls the lens) Optic Retina nerve Figure 3.7 The Human Eye. The composition of the human eye. Copyright 2017 Cengage Learning. All Rights Reserved. May not be copied, scanned, or duplicated, in whole or in part. Due to electronic rights, some third party content may be suppressed from the eBook and/or eChapter(s). Editorial review has deemed that any suppressed content does not materially affect the overall learning experience. Cengage Learning reserves the right to remove additional content at any time if subsequent rights restrictions require it. 80 CHAPTER 3 Visual Perception are sensitive to light and dark stimuli. The cones are short and thick photoreceptors and allow for the perception of color. They are more highly concentrated in the foveal region than in the periphery of the retina (Durgin, 2000). The rods and cones are connected to the brain. The neurochemical messages pro- cessed by the rods and cones of the retina travel via the bipolar cells to the ganglion cells (see Goodale, 2000a, 2000b). The axons of the ganglion cells in the eye collectively form the optic nerve for that eye. The optic nerves of the two eyes join at the base of the brain to form the optic chiasma (see Figure 2.8 in Chapter 2). At this point, the ganglion cells from the inward, or nasal, part of the retina—the part closer to your nose—cross through the optic chiasma and extend to the opposite hemisphere of the brain. The gan- glion cells from the outward, or temporal area of the retina closer to your temple go to the hemisphere on the same side of the body. The lens of each eye naturally inverts the image of the world as it projects the image onto the retina. In this way, the message sent to your brain is literally upside-down and backward. After being routed via the optic chiasma, about 90% of the ganglion cells then go to the lateral geniculate nucleus of the thalamus. From the thalamus, neurons carry infor- mation to the primary visual cortex (V1 or striate cortex) in the occipital lobe of the brain. The visual cortex contains several processing areas. Each area handles different kinds of visual information relating to intensity and quality, including color, location, depth, pattern, and form. Pathways to Perceive the What and the Where What are the visual pathways in the brain? A pathway in general is the path the visual information takes from its entering the human perceptual system through the eyes to its being completely processed. Generally, researchers agree that there are two pathways. Work on visual perception has identified separate neural pathways in the cerebral cortex for processing different aspects of the same stimuli (De Haan & Cowey, 2011; De Yoe & Van Essen, 1988; Köhler et al., 1995). Perception deficits, such as ataxia and agnosia, indicate the existence of different pathways. Both of these perception deficits are covered later in this chapter. Why are there two pathways? The information from the primary visual cortex in the occipital lobe is forwarded through two fasciculi (fiber bundles): One ascends toward the parietal lobe (along the dorsal pathway), and one descends to the temporal lobe (along the ventral pathway). The dorsal pathway is also called the where pathway and is responsible for processing location and motion information; the ventral pathway is called the what pathway because it is mainly responsible for processing the color, shape, and identity of visual stimuli (Patel, Lin, & Khaderi, 2014; Ungerleider & Haxby, 1994; Ungerleider & Mishkin, 1982). This is the what–where hypothesis. Most of the research in this area has been carried out with monkeys. In particular, a group of monkeys with lesions in the temporal lobe were able to indicate where things were but seemed unable to recognize what they were. In contrast, monkeys with lesions in the parietal lobe were able to recognize what things were but not where they were. The what–how hypothesis is an alternative interpretation of the visual pathways (Goodale, 2011; Goodale & Milner, 2004; Goodale & Westwood, 2004). This hypothesis suggests the two pathways refer not to what things are and to where they are, but rather to what they are and to how they function. According to the what–how hypothesis, spa- tial information about where something is located in space is always present in visual information processing. What differs between the two pathways is whether the emphasis is on identifying what an object is or, instead, on how we can situate ourselves so as to grasp the object. Copyright 2017 Cengage Learning. All Rights Reserved. May not be copied, scanned, or duplicated, in whole or in part. Due to electronic rights, some third party content may be suppressed from the eBook and/or eChapter(s). Editorial review has deemed that any suppressed content does not materially affect the overall learning experience. Cengage Learning reserves the right to remove additional content at any time if subsequent rights restrictions require it. Visual Perception CHAPTER 3 81 The what pathway can be found in the ventral stream and is responsible for the identification of objects. The how pathway is located in the dorsal stream and controls movements in relation to the objects that have been identified through the what path- way. Ventral and dorsal streams both arise from the same early visual areas (Milner & Goodale, 2008). The what–how hypothesis is best supported by evidence of processing deficits: There are deficits that impair people’s ability to recognize what they see and there are distinct deficits that impair people’s ability to reach for what they see (how). C O N C E PT C H E C K 1. What is the difference between sensation and perception? 2. What is the difference between the distal and the perceptual object? 3. How are rods and cones both similar to and different from each other? 4. What are some of the major parts of the eye and what are their functions? 5. What is the what–where hypothesis? Approaches to Perception: How Do We Make Sense of What We See? Now that we know how a light stimulus that enters our eye is processed and routed to the brain, the question still remains as to how we actually perceive what we see. Do we just perceive whatever is being projected on our retina, or is there more to perception? Does our knowledge, and other rules we have learned throughout our life, perhaps influence our perception of the world? Going back to our view out of the window, the image on our retina suggests that the buildings we see in the distance are small. We do see other buildings, trees, and streets in front of them, however, that suggest that those buildings are in fact large and just appear small because they are far away from our office. In this case, our experience with and knowledge about perception and the world allow us to perceive those buildings as being tall even though they do not look larger than our hand in front of us on our desk. There are different views on how we perceive the world. These views can be sum- marized as bottom-up theories and top-down theories. Bottom-up theories describe approaches in which perception starts with the stimuli whose appearance you take in through your eye. You look out onto the cityscape, and perception happens when the light information is transported to your brain. Therefore, they are data-driven (i.e., stim- ulus-driven) theories. Not all theorists focus on the sensory data of the perceptual stimulus. Many theorists prefer top-down theories, according to which perception is driven by high-level cogni- tive processes, existing knowledge, and the prior expectations that influence perception (Clark, 2003). These theories then work their way down to considering the sensory data, such as the perceptual stimulus. You perceive buildings as big in the background of the city scene because you know these buildings are far away and therefore must be bigger than they appear. From this viewpoint, expectations are important. When people expect to see something, they may see it even if it is not there or is no longer there. For example, suppose people expect to see a certain person in a certain location. They may think they Copyright 2017 Cengage Learning. All Rights Reserved. May not be copied, scanned, or duplicated, in whole or in part. Due to electronic rights, some third party content may be suppressed from the eBook and/or eChapter(s). Editorial review has deemed that any suppressed content does not materially affect the overall learning experience. Cengage Learning reserves the right to remove additional content at any time if subsequent rights restrictions require it. 82 CHAPTER 3 Visual Perception see that person, even if they are actually seeing someone else who looks only vaguely similar (Simons, 1996). Top-down and bottom-up approaches have been applied to virtually every aspect of cognition. Bottom-up and top-down approaches usually are presented as being in opposition to each other. But to some extent, they deal with different aspects of the same phenomenon. Ultimately, a complete theory of perception will need to encompass both bottom-up and top-down processes. Bottom-Up Theories The four main bottom-up theories of form and pattern perception are direct perception, template theories, feature theories, and recognition-by-components theory. Direct Perception How do you know the letter A when you see it? Easy to ask, hard to answer. Of course, it’s an A because it looks like an A. However, what makes it look like an A instead of like an H? Just how difficult it is to answer this question becomes apparent when you look at Figure 3.8. You probably will see the image in Figure 3.8 as the words THE CAT. Yet the H of THE is identical to the A of CAT. What subjectively feels like a simple process of pattern recognition is almost certainly complex. Gibson’s Theory of Direct Perception How do we connect what we perceive to what we have stored in our minds? According to Gibson’s theory of direct perception, the information in our sensory receptors, including the sensory context, is all we need to perceive anything. As the envi- ronment supplies us with all the information we need for perception, this view is some- times also called ecological perception. In other words, we do not need higher cognitive processes or anything else to mediate between our sensory experiences and our percep- tions. Existing beliefs or higher-level inferential thought processes are not necessary for perception. Gibson (1979) believed that, in the real world, sufficient contextual information usually exists to make perceptual judgments. We do not need to appeal to higher-level intelligent processes to explain perception but rather use contextual information directly. In essence, we are biologically tuned to respond to it. According to Gibson, we use tex- ture gradients as cues for depth and distance. Those cues aid us to perceive directly the relative proximity or distance of objects and of parts of objects. In Figure 3.9 , you can see different rock formations at the seacoast. For the rocks that are closest to the photographer, you can see many details, such as notches, holes, and variations in color. The farther away the objects on the picture are, the fewer the details you can see. You are using texture gradients as an indicator of how far away the rocks are. And because some of the rocks cover up parts of other rocks, you infer from that information that the rocks that are partly covered must be farther away than the rocks that cover them. On the basis of our analysis of the stable relationships among Figure 3.8 Can You Read These Words? When you read these words, you proba- bly have no difficulty differentiating the A from the H. Look more closely at each of these two letters. What features differentiate them? Copyright 2017 Cengage Learning. All Rights Reserved. May not be copied, scanned, or duplicated, in whole or in part. Due to electronic rights, some third party content may be suppressed from the eBook and/or eChapter(s). Editorial review has deemed that any suppressed content does not materially affect the overall learning experience. Cengage Learning reserves the right to remove additional content at any time if subsequent rights restrictions require it. Visual Perception CHAPTER 3 83 Courtesy of the author Figure 3.9 Cues Used in Depth Perception. The farther away an object is, the fewer details you can see. You can see small holes and the rough texture of the rock in the foreground, whereas the rocks in the background look much smoother. The rock that is partly obscured is located behind the rock that obscures it. We use these cues to aid us in depth perception. features of objects and settings in the real world, we directly perceive our environment (Gibson, 1950, 1954/1994; Mace, 1986). We do not need the aid of complex thought processes. Such contextual information might not be readily controlled in a laboratory experi- ment. But such information is likely to be available in a real-world setting. Therefore, as noted previously, Gibson’s model sometimes is referred to as an ecological model (Turvey, 2003). This reference is a result of Gibson’s concern with perception as it occurs in the everyday world (the ecological environment) rather than in laboratory situations, where less contextual information is available. Ecological constraints apply not only to initial perceptions but also to the ultimate internal representations (such as concepts) that are formed from those perceptions (de Wit, van der Kamp, & Withagen, 2015; Hubbard, 1995; Shepard, 1984). Continuing to wave the Gibsonian banner was Eleanor Gibson (1991, 1992), James’s wife, and, like her husband, a highly distinguished scholar. She conducted landmark research in infant perception. She observed that infants (who certainly lack much prior knowledge and experience) quickly develop many aspects of perceptual awareness, including depth perception. Direct perception may also play a role in interpersonal situations when we try to make sense of others’ emotions and intentions (Gallagher, 2008). After all, we can almost instantaneously recognize facial expression as a whole emotion; we do not see multiple aspects of facial expressions that we then try, aspect by aspect, to piece together into the perception of an emotion (Wittgenstein, 1980). Copyright 2017 Cengage Learning. All Rights Reserved. May not be copied, scanned, or duplicated, in whole or in part. Due to electronic rights, some third party content may be suppressed from the eBook and/or eChapter(s). Editorial review has deemed that any suppressed content does not materially affect the overall learning experience. Cengage Learning reserves the right to remove additional content at any time if subsequent rights restrictions require it. 84 CHAPTER 3 Visual Perception Neuroscience and Direct Perception Neuroscience also indicates that direct perception may be involved in person perception. About 30 to 100 milliseconds after a visual stimulus, mirror neurons start firing. Mirror neurons are active both when a person acts and when he or she observes that same act performed by somebody else. So before we even have time to form hypotheses about what we are perceiving, we may already be able to understand the expressions, emotions, and movements of the person we observe (Gallagher, 2008). Furthermore, studies indicate that separate neural pathways (what pathways) in the lateral occipital area process form, color, and texture in objects. When asked to judge the length of an object, for example, people cannot ignore the width. Color, form, and texture of an object, however, can be judged independently of the other qualities (Cant & Goodale, 2007; Cant et al., 2008). Template Theories Template theories suggest that our minds store myriad sets of templates. Templates are highly detailed models for patterns we might recognize. We recognize a pattern by comparing it with our set of templates. We then choose the exact template that perfectly matches what we observe (Selfridge & Neisser, 1960). We see examples of template matching in our everyday lives. Fingerprints are matched in this way. Machines rapidly process imprinted numerals on checks by comparing them to templates. Products of all kinds are identified with universal product codes (UPCs or “bar codes”). They can be scanned and identified by computers at the time of purchase. Chess players who have knowledge of many games use a matching strategy in line with template theory to recall previous games (Gobet & Jackson, 2002). Template matching theories belong to the group of chunk-based theories; these theories suggest that expertise is attained by acquiring chunks of knowledge in long-term memory that can later be accessed for fast recognition. Studies with chess players have shown that the temporal lobe is indeed activated when the players access the stored chunks in their long-term memory (Campitelli et al., 2007). In each of these instances, the goal is to find one perfect match and disregard imper- fect matches. You would be alarmed to find that your bank’s numeral-recognition system failed to register a deposit to your account. Such failure might occur because it was pro- grammed to accept an ambiguous character according to what seemed to be a best guess. For template matching, only an exact match will do. This is exactly what you want from a bank computer. Consider, however, your perceptual system at work in everyday situa- tions. It rarely would work if you required exact matches for every stimulus you were to recognize. Imagine, for example, needing mental templates for every possible percept of the face of someone you love. Imagine one for each facial expression, each angle of view- ing, each addition or removal of makeup, each hairdo, and so on. Template-matching theories fail to explain some aspects of the perception of letters. For one thing, such theories cannot easily account for our perception of the letters and words in Figure 3.8 (“THE CAT”). We identify two different letters (A and H) from only one physical form. Hoffding (1891) noted other problems. We can recognize an A as an A despite variations in the size, orientation, and form in which the letter is written. Are we to believe that we have mental templates for each possible size, orientation, and form of a letter? Storing, organizing, and retrieving so many templates in memory would be unwieldy. How could we possibly anticipate and create so many templates for every con- ceivable object of perception (Figure 3.10 )? Neuroscience and Template Theories Letters of the alphabet are simpler than faces and other complex stimuli. But how do we recognize letters? And does it make Copyright 2017 Cengage Learning. All Rights Reserved. May not be copied, scanned, or duplicated, in whole or in part. Due to electronic rights, some third party content may be suppressed from the eBook and/or eChapter(s). Editorial review has deemed that any suppressed content does not materially affect the overall learning experience. Cengage Learning reserves the right to remove additional content at any time if subsequent rights restrictions require it. Visual Perception CHAPTER 3 85 a difference to our brain whether we perceive letters or digits? Experiments suggest that there is indeed a difference between letters and digits. An area on or near the left fusiform gyrus (part of the occipital and temporal lobes) is activated signifi- cantly more when a person is presented with letters than with A digits. It is not clear whether this “letter area” only processes 7 611146 922892 letters or whether it also plays a more minor role in the process- ing of digits (Polk et al., 2002). The notion of the visual cortex specializing in different stimuli is not new; other areas have been found that specialize in faces, for example (see Kanwisher, McDermott, & Chun, 1997; McCarthy et al., 1997). Later in this 7 611146 922892 chapter, we will consider in more detail the structures of the brain that enable us to recognize faces. Why Computers Have Trouble Reading Handwriting Think about how easy it is for you to perceive and understand 7 611146 922892 someone’s handwriting. In handwriting, everybody’s numbers and letters look a bit different. You can still distinguish them without any problems (at least in most cases). This is something computers do not do well. For computers, reading handwrit- ing is incredibly difficult and susceptible to mistakes. When you deposit a check at an automated teller machine, it “reads” your 7 611146 922892 check automatically. In fact, the numbers at the bottom of your check that are written in a strange-looking font are so distinct Figure 3.10 Template Matching in Barcodes and Letters. A particular barcode will always that a machine cannot mistake them for one another. It is much look exactly the same way, making it easy for harder, however, for a machine to decipher handwriting. Simi- computers to read. Letters, to the contrary, can larly, a machine also will have trouble determining that all the look different although they depict the same letter. letters in the right of Figure 3.10 are A’s (unless it has a template Template matching will distinguish between differ- for each one of the As). Therefore, some computers work with ent bar codes but will not recognize that different versions of the letter A written in different scripts algorithms that consider the context in which the word is pre- are indeed both A’s. sented, the angular positions of the written letters (e.g., upright or tilted), and other factors. You are probably familiar with CAPTCHAs (Completely Automated Public Turing Test to Tell Computers and Humans Apart; see Figure 3.11). They are used to tell humans and computers apart so that spamming machines cannot post spam on web- sites or sign up for e-mail accounts and send spam through those accounts. CAPTCHAs capitalize on the fact that most approaches to machine vision use template matching (Hannagan et al., 2012). But as you can imagine, new approaches are continuously being developed to improve reading abilities of machines so, at some point, CAPTCHAs might have to be developed that are more intricate. Feature-Matching Theories Yet another alternative explanation of pattern and form perception may be found in feature-matching theories. According to these theories, we attempt to match features of a pattern to features stored in memory, rather than to match a whole pattern to a tem- plate or a prototype (Stankiewicz, 2003). The Pandemonium Model One such feature-matching model has been called Pan- demonium (“pandemonium” refers to a noisy, chaotic place and hell). In this model, metaphorical “demons” with specific duties receive and analyze the features of a stimulus (Selfridge, 1959). Copyright 2017 Cengage Learning. All Rights Reserved. May not be copied, scanned, or duplicated, in whole or in part. Due to electronic rights, some third party content may be suppressed from the eBook and/or eChapter(s). Editorial review has deemed that any suppressed content does not materially affect the overall learning experience. Cengage Learning reserves the right to remove additional content at any time if subsequent rights restrictions require it. 86 CHAPTER 3 Visual Perception Figure 3.11 CAPTCHAS are used to tell humans and computers apart. epa european pressphoto agency b.v./Alamy In the Pandemonium model, conceived by Oliver Selfridge, there are four kinds of demons: image demons, feature demons, cognitive demons, and decision demons. Figure 3.12 shows this model. The “image demons” receive a retinal image and pass it on to “feature demons.” Each feature demon calls out when matches are made between the stimulus and the given feature. These matches are yelled out at demons at the next level of the hierarchy, the “cognitive (thinking) demons.” The cognitive demons in turn shout out possible patterns stored in memory that conform to one or more of the features noticed by the feature demons. A “decision demon” listens to the pandemonium of the cognitive demons. It decides on what has been seen, based on which cognitive demon is shouting the most frequently (i.e., which has the most matching features). Other feature models have been proposed (Grainger, Rey, & Dufau, 2008), but Selfridge’s Pandemonium model is one of the most widely known. Most other fea- ture models distinguish different features as well as different kinds of features, such as global versus local features. Local features constitute the small-scale or detailed aspects of a given pattern. There is no consensus as to what exactly constitutes a local feature. Nevertheless, we generally can distinguish such features from global features, the features that give a form its overall shape. Consider, for example, the stimuli depicted in Figures 3.13(a) and 3.13(b). These are of the type used in some research on pattern perception (e.g., see Navon, 1977, or Olesen et al., 2007). Globally, the stimuli in panels (a) and (b) form the letter H. In panel (a), the local features (small H’s) correspond to the global ones. In panel (b), including many local letter S’s, they do not. In one study, participants were asked to identify the stimuli at either the global or the local level (Navon, 1977). When the local letters were small and positioned close together, participants could identify stimuli at the global level (the “big” letter) more quickly than at the local level. When participants were required to identify stimuli at the global level, whether the local features (small letters) matched the global one (big letter) did not mat- ter. They responded equally rapidly whether the global H was made up of local H’s or of local S’s. However, when the participants were asked to identify the “small” local letters, they responded more quickly if the global features agreed with the local ones. In other Copyright 2017 Cengage Learning. All Rights Reserved. May not be copied, scanned, or duplicated, in whole or in part. Due to electronic rights, some third party content may be suppressed from the eBook and/or eChapter(s). Editorial review has deemed that any suppressed content does not materially affect the overall learning experience. Cengage Learning reserves the right to remove additional content at any time if subsequent rights restrictions require it. Visual Perception CHAPTER 3 87 Cognitive demons Feature demons (“shout” when they receive (decode specific certain combinations features) of features) Vertical lines 1 A 2 B 3 4 C D Horizontal lines E 1 2 F 3 4 G H Oblique lines Decision demon (“listens” for Image demon (receives sensory input) 1 2 I J loudest shout 3 in pandemonium R 4 K to identify input) Right angles L P? D? R Processing 1 M R of signal 2 3 N 4 O Acute angles P 1 2 Q 3 R 4 Discontinuous S curves T 1 2 3 U 4 V Continuous W curves 1 X 2 3 Y 4 Z Figure 3.12 Selfridge’s Feature-Matching Model. According to Oliver Selfridge’s feature-matching model, we recognize patterns by matching observed features to features already stored in memory. We recognize the patterns for which we have found the greatest number of matches. In the example above, the feature demons indicate that the sensory input (the letter R) has one vertical line, two horizontal lines, one oblique line, two right angles, two acute angles, and one discontinuous curve. These features permit the cognitive demons to conclude that the letter in ques- tion may be either the letter D, P, or R. The decision demon then makes a decision on which letter has been perceived. words, they were slowed down if they had to identify local (small) S’s combining to form a global (big) H instead of identifying local (small) H’s combining to form a global (big) H. This pattern of results is called the global precedence effect (see also Kimchi, 1992). Experiments show global information dominates over local information even in infants (Cassia et al., 2002). In contrast, when letters are more widely spaced, as in Figures 3.14(a) and 3.14(b) , the effect is reversed. Then a local precedence effect appears. That is, the Copyright 2017 Cengage Learning. All Rights Reserved. May not be copied, scanned, or duplicated, in whole or in part. Due to electronic rights, some third party content may be suppressed from the eBook and/or eChapter(s). Editorial review has deemed that any suppressed content does not materially affect the overall learning experience. Cengage Learning reserves the right to remove additional content at any time if subsequent rights restrictions require it. 88 CHAPTER 3 Visual Perception H H S S participants more quickly identify the local features of the individual letters H H S S than the global ones, and the local features interfere with the global recogni- H H S S tion in cases of contradictory stimuli (Martin, 1979). So when the letters are H H S S close together at the local level, people have problems identifying the local HHHHHH S S S S S S stimuli (small letters) if they are not concordant with the global stimulus H H S S S S (big letter). When the letters on the local level are relatively far apart from H H S S each other, it is harder for people to identify the global stimulus (big letter) H H H H S S if it is not concordant with the local stimuli (small letters). Other limitations (e.g., the size of the stimuli) besides special proximity of the local stimuli (a) (b) hold as well, and other kinds of features also influence perception. Figure 3.13 The Global Precedence Effect. Com- Neuroscience and Feature-Matching Theories Some support pare panel (a), a global H made of local H’s, with for feature theories comes from neurological and physiological research. panel (b), a global H made Researchers used single-cell recording techniques with animals (Hubel & of local S’s. All the local let- Wiesel, 1963, 1968, 1979). They carefully measured the responses of indi- ters are tightly spaced. vidual neurons to visual stimuli in the visual cortex. Then they mapped Source: Based on D. Navon, “Forest before Trees: The Prec- those neurons to corresponding visual stimuli for particular locations in edence to Global Features in the visual field (see Chapter 2). Their research showed that the visual cortex Visual Perception,” Cognitive Psychology, July 1977, Vol. 9, contains specific neurons that respond only to a particular kind of stimulus No. 3, pp. 353–382. (e.g., a horizontal line), and only if that stimulus fell onto a specific region of the retina. Each individual cortical neuron, therefore, can be mapped to a specific receptive field on the retina. A disproportionately large amount of the visual cortex is devoted to neurons mapped to receptive fields in the foveal region of the retina, which is the area of the most acute vision. Most of the cells in the cortex do not respond simply to spots of light. Rather, they respond to “specifically oriented line segments” (Hubel & Wiesel, 1979, p. 9). Moreover, these cells seem to show a hierarchical structure in the degree of complex- ity of the stimuli to which they respond, somewhat in line with the ideas behind the Pandemonium Model. That means that the outputs of the cells are combined to cre- ate higher-order detectors that can identify increasingly more complex features. At the lowest level, cells respond to lines; at a higher level, they respond to corners and edges, then to shapes, and so forth. Neurons that can recognize a complex object are called gnostic units or “grandmother cells” because they imply that there is a neuron that is capable of recognizing your grandmother. None of those neurons are so spe-