Cell Cycle Final Review PDF
Document Details
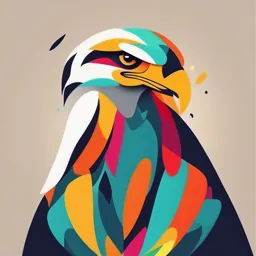
Uploaded by SucceedingLavender
Tags
Related
- DNA Biology & Cell Division PDF
- Molecular Biology Lecture 2: Regulation of Cell Division PDF
- Molecular and Cell Biology - The Cell Cycle (GC11) PDF
- Cell Cycle & DNA Replication Lecture 5 PDF
- Cell and Molecular Biology Study Guide PDF
- Molecular Biology of the Cell Textbook - Chapter 17: The Cell Cycle PDF
Summary
This document provides a comprehensive overview and review of the cell cycle, covering key phases, regulatory mechanisms, and control systems. It details the processes of chromosome duplication, mitosis, and cytokinesis.
Full Transcript
Cell cycle The cell cycle This cycle of duplication and division, known as the cell cycle, is the essential mechanism by which all living things reproduce. At a minimum, the cell must accomplish its most fundamental task: the passing on of its genetic information to the next generation of cells. Chr...
Cell cycle The cell cycle This cycle of duplication and division, known as the cell cycle, is the essential mechanism by which all living things reproduce. At a minimum, the cell must accomplish its most fundamental task: the passing on of its genetic information to the next generation of cells. Chromosome duplication occurs during S phase (S for DNA synthesis), which requires 10–12 hours and occupies about half of the cell-cycle time in a typical mammalian cell. After S phase, chromosome segregation and cell division occur in M phase (M for mitosis), which requires much less time (less than an hour in a mammalian cell). M phase comprises two major events: nuclear division, or mitosis, during which the copied chromosomes are distributed into a pair of daughter nuclei; and cytoplasmic division, or cytokinesis, when the cell itself divides in two Overview of the cell cycle The eukaryotic cell cycle usually consists of four phases Partly to allow time for growth, most cell cycles have gap phases—a G1 phase between M phase and S phase and a G2 phase between S phase and mitosis. Thus, the eukaryotic cell cycle is traditionally divided into four sequential phases: G1, S, G2, and M. G1, S, and G2 together are called interphase. In a typical human cell proliferating in culture, interphase might occupy 23 hours of a 24-hour cycle, with 1 hour for M phase. Cell growth occurs throughout the cell cycle, except during mitosis. The two gap phases are more than simple time delays to allow cell growth. They also provide time for the cell to monitor the internal and external environment to ensure that conditions are suitable and preparations are complete before the cell commits itself to the major upheavals of S phase and mitosis. If extracellular conditions are unfavorable, for example, cells delay progress through G1 and may even enter a specialized resting state known as G0 (G zero), in which they can remain for days, weeks, or even years before resuming proliferation. Indeed, many cells remain permanently in G0 until they or the organism dies. If extracellular conditions are favorable and signals to grow and divide are present, cells in early G1 or G0 progress through a commitment point near the end of G1 known as Start (in yeasts) or the restriction point (in mammalian cells). We will use the term Start for both yeast and animal cells. After passing this point, cells are committed to DNA replication, even if the extracellular signals that stimulate cell growth and division are removed The control of the cell cycle In most eukaryotic cells, the cell-cycle control system governs cell-cycle progression at three major regulatory transitions: 1) The first is Start (or the restriction point) in late G1, where the cell commits to cell-cycle entry and chromosome duplication. 2) The second is the G2/M transition, where the control system triggers the early mitotic events that lead to chromosome alignment on the mitotic spindle in metaphase. 3) The third is the metaphase-toanaphase transition, where the control system stimulates sister-chromatid separation, leading to the completion of mitosis and cytokinesis The Cell-Cycle Control System Depends on Cyclically Activated Cyclin-Dependent Protein Kinases (Cdks) Central components of the cell-cycle control system are members of a family of protein kinases known as cyclin-dependent kinases (Cdks). The activities of these kinases rise and fall as the cell progresses through the cycle Cyclical changes in Cdk activity are controlled by a complex array of enzymes and other proteins. The most important of these Cdk regulators are proteins known as cyclins. Cdks, as their name implies, are dependent on cyclins for their activity: unless they are bound tightly to a cyclin, they have no protein kinase activity Cyclins were originally named because they undergo a cycle of synthesis and degradation in each cell cycle. The levels of the Cdk proteins, by contrast, are constant. Cyclical changes in cyclin protein levels result in the cyclic assembly and activation of cyclin– Cdk complexes at specific stages of the cell cycle. Cyclin–Cdk complexes of the cellcycle control system. The concentrations of the three major cyclin types oscillate during the cell cycle, while the concentrations of Cdks (not shown) do not change and exceed cyclin amounts. There are four classes of cyclins, each defined by the stage of the cell cycle at which they bind Cdks and function 1) G1/S-cyclins activate Cdks in late G1 and thereby help trigger progression through Start, resulting in a commitment to cellcycle entry. Their levels fall in S phase. 2) S-cyclins bind Cdks soon after progression through Start and help stimulate chromosome duplication. S-cyclin levels remain elevated until mitosis, and these cyclins also contribute to the control of some early mitotic events. 3) M-cyclins activate Cdks that stimulate entry into mitosis at the G2/M transition. M-cyclin levels fall in mid-mitosis. 4) In most cells, a fourth class of cyclins, the G1-cyclins, helps govern the activities of the G1/S-cyclins, which control progression through start in late G1. How do different cyclin–Cdk complexes trigger different cell-cycle events? The answer, at least in part, seems to be that the cyclin protein does not simply activate its Cdk partner but also directs it to specific target proteins. As a result, each cyclin–Cdk complex phosphorylates a different set of substrate proteins. The structural basis of Cdk activation. A) Studies of the three-dimensional structures of Cdk and cyclin proteins have revealed that, in the absence of cyclin, the active site in the Cdk protein is partly obscured by a protein loop, like a stone blocking the entrance to a cave B) Cyclin binding causes the loop to move away from the active site, resulting in partial activation of the Cdk enzyme. C) Full activation of the cyclin–Cdk complex then occurs when a separate kinase, the Cdkactivating kinase (CAK), phosphorylates an amino acid near the entrance of the Cdk active site. This causes a small conformational change that further increases the activity of the Cdk, allowing the kinase to phosphorylate its target proteins effectively and thereby induce specific cell-cycle events Regulation of CDK activity by phosphorylation Phosphorylation at a pair of amino acids in the roof of the kinase active site inhibits the activity of a cyclin–Cdk complex. Phosphorylation of these sites by a protein kinase known as Wee1 inhibits Cdk activity, while dephosphorylation of these sites by a phosphatase known as Cdc25 increases Cdk activity. We will see later that this regulatory mechanism is particularly important in the control of M-Cdk activity at the onset of mitosis. Binding of Cdk inhibitor proteins (CKIs) inactivates cyclin–Cdk complexes. The threedimensional structure of a cyclin–Cdk–CKI complex reveals that CKI binding stimulates a large rearrangement in the structure of the Cdk active site, rendering it inactive. Cells use CKIs primarily to help govern the activities of G1/Sand S-Cdks early in the cell cycle. Metaphase-to-anaphase transition The key regulator of the metaphaseto-anaphase transition is the anaphase promoting complex, or cyclosome (APC/C), a member of the ubiquitin ligase family of enzymes. Following its activation in midmitosis, the APC/C remains active in G1 to provide a stable period of Cdk inactivity. When G1/S-Cdk is activated in late G1, the APC/C is turned off, thereby allowing cyclin accumulation in the next cell cycle. APC/C catalyzes the ubiquitylation and destruction of two major types of proteins. 1) securin, which protects the protein linkages that hold sister-chromatid pairs together in early mitosis. 1) Destruction of securin in metaphase activates a protease that separates the sisters and unleashes anaphase, as described later. 2) The S- and M-cyclins are the second major targets of the APC/C. 1) As a result, the many proteins phosphorylated by Cdks from S phase to early mitosis are dephosphorylated by various phosphatases in the anaphase cell. This dephosphorylation of Cdk targets is required for the completion of M phase, including the final steps in mitosis and then cytokinesis. Control of proteolysis by SCF The cell-cycle control system also uses another ubiquitin ligase called SCF Function: to ubiquitylate certain CKI proteins in late G1, thereby helping to control the activation of S-Cdks and DNA replication. SCF is also responsible for the destruction of G1/S-cyclins in early S phase. Ubiquitylation by SCF is controlled instead by changes in the phosphorylation state of its target proteins, as F-box subunits recognize only specifically phosphorylated proteins Short summary Central components of the control system are the cyclindependent protein kinases (Cdks), which depend on cyclin subunits for their activity. Oscillations in the activities of different cyclin–Cdk complexes control various cell-cycle events. activation of S-phase cyclin–Cdk complexes (S-Cdk) initiates S phase, whereas activation of M-phase cyclin–Cdk complexes (M-Cdk) triggers mitosis The mechanisms that control the activities of cyclin–Cdk complexes include phosphorylation of the Cdk subunit, binding of Cdk inhibitor proteins (CKIs), proteolysis of cyclins, and changes in the transcription of genes encoding Cdk regulators The cell-cycle control system also depends crucially on two additional enzyme complexes, the APC/C and SCF ubiquitin ligases, which catalyze the ubiquitylation and consequent destruction of specific regulatory proteins that control critical events in the cycle (slide 13-14). S-Cdk Initiates DNA Replication Once Per Cycle To ensure that chromosome duplication occurs only once per cell cycle, the initiation phase of DNA replication is divided into two distinct steps that occur at different times in the cell cycle: 1) The first step occurs in late mitosis and early G1, when a pair of inactive DNA helicases is loaded onto the replication origin, forming a large complex called the prereplicative complex or preRC. This step is sometimes called licensing of replication origins because initiation of DNA synthesis is permitted only at origins containing a preRC. 2) The second step occurs in S phase, when the DNA helicases are activated, resulting in DNA unwinding and the initiation of DNA synthesis. Once a replication origin has been fired in this way, the two helicases move out from the origin with the replication forks, and that origin cannot be reused until a new preRC is assembled there at the end of mitosis. As a result, origins can be activated only once per cell cycle. Control of the initiation of DNA replication. At the onset of S phase, S-Cdk triggers origin activation by phosphorylating specific initiator proteins, which then nucleate the assembly of a large protein complex that activates the DNA helicase and recruits the DNA synthesis machinery. Summary for S phase Duplication of the chromosomes in S phase involves the accurate replication of the entire DNA molecule in each chromosome, as well as the duplication of the chromatin proteins that associate with the DNA and govern various aspects of chromosome function. Chromosome duplication is triggered by the activation of S-Cdk, which activates proteins that unwind the DNA and initiate its replication at replication origins. Once a replication origin is activated, S-Cdk also inhibits proteins that are required to allow that origin to initiate DNA replication again. Thus, each origin is fired once and only once in each S phase and cannot be reused until the next cell cycle. Mitosis Following the completion of S phase and transition through G2, the cell undergoes the dramatic upheaval of M phase. This begins with mitosis, during which the sister chromatids are separated and distributed (segregated) to a pair of identical daughter nuclei, each with its own copy of the genome. Mitosis is traditionally divided into five stages—prophase, prometaphase, metaphase, anaphase, and telophase—defined primarily on the basis of chromosome behavior as seen in a microscope. As mitosis is completed, the second major event of M phase—cytokinesis—divides the cell into two halves, each with an identical nucleus. Panel 17–1 summarizes the major events of M phase Regulations Mitosis From a regulatory point of view, mitosis can be divided into two major parts, each governed by distinct components of the cell-cycle control system: 1) an abrupt increase in M-Cdk activity at the G2/M transition triggers the events of early mitosis (prophase, prometaphase, and metaphase). During this period, M-Cdk and several other mitotic protein kinases phosphorylate a variety of proteins, leading to the assembly of the mitotic spindle and its attachment to the sister-chromatid pairs. 2) The second major part of mitosis begins at the metaphaseto-anaphase transition, when the APC/C triggers the destruction of securin, liberating a protease that cleaves cohesin and thereby initiates separation of the sister chromatids. The APC/C also promotes the destruction of cyclins, which leads to Cdk inactivation and the dephosphorylation of Cdk targets, which is required for all events of late M phase, including the completion of anaphase, the disassembly of the mitotic spindle, and the division of the cell by cytokinesis. M-CdK drives entry into Mitosis M-Cdk activation begins with the accumulation of M-cyclin The increase in M-cyclin protein leads to a corresponding accumulation of M-Cdk the Cdk in these complexes is phosphorylated at an activating site by the Cdk-activating kinase (CAK) the protein kinase Wee1 holds it in an inactive state by inhibitory phosphorylation at two neighboring sites. Thus, by the time the cell reaches the end of G2, it contains an abundant stockpile of M-Cdk that is primed and ready to act but is suppressed by phosphates that block the active site of the kinase. What, then, triggers the activation of the M-Cdk stockpile? The crucial event is the activation of the protein phosphatase Cdc25, which removes the inhibitory phosphates that restrain M-Cdk At the same time, the inhibitory activity of the kinase Wee1 is suppressed, further ensuring that MCdk activity increases. Interestingly, Cdc25 can also be activated, at least in part, by its target, M-Cdk. M-Cdk may also inhibit the inhibitory kinase Wee1. The ability of M-Cdk to activate its own activator (Cdc25) and inhibit its own inhibitor (Wee1) suggests that M-Cdk activation in mitosis involves positive feedback loops. Such a mechanism would quickly promote the activation of all M-Cdk complexes in the cell. The Mitotic Spindle Is a MicrotubuleBased Machine https://www.khanacademy.org/testprep/mcat/cells/cytoskeleton/v/microtubules2 central event of mitosis—chromosome segregation—depends in all eukaryotes on a complex and beautiful machine called the mitotic spindle M-Cdk triggers the assembly of the spindle early in mitosis, in parallel with the chromosome restructuring just described. The core of the mitotic spindle is a bipolar array of microtubules, the minus ends of which are focused at the two spindle poles, and the plus ends of which radiate outward from the poles The metaphase mitotic spindle in an animal cell. The plus ends of some microtubules— called the interpolar microtubules—overlap with the plus ends of microtubules from the other pole, resulting in an antiparallel array in the spindle midzone. The plus ends of other microtubules—the kinetochore microtubules—are attached to sister-chromatid pairs at large protein structures called kinetochores, which are located at the centromere of each sister chromatid. Finally, many spindles also contain astral microtubules that radiate outward from the poles and contact the cell cortex, helping to position the spindle in the cell Microtubule-Dependent Motor Proteins Govern Spindle Assembly and Function These proteins belong to two families—the kinesin-related proteins, which usually move toward the plus end of microtubules, and dyneins, which move toward the minus end. In the mitotic spindle, these motor proteins generally operate at or near the ends of the microtubules. Four major types of motor proteins— kinesin-5, kinesin-14, kinesins-4/10, and dynein—are particularly important in spindle assembly and function Major motor proteins of the spindle Kinesin-5 proteins contain two motor domains that interact with the plus ends of antiparallel microtubules in the spindle midzone. Because the two motor domains move toward the plus ends of the microtubules, they slide the two antiparallel microtubules past each other toward the spindle poles, pushing the poles apart. Kinesin-14 proteins, by contrast, are minus-end directed motors with a single motor domain and other domains that can interact with a neighboring microtubule. They can cross-link antiparallel interpolar microtubules at the spindle midzone and tend to pull the poles together. Kinesin-4 and kinesin-10 proteins, also called chromokinesins, are plus-end directed motors that associate with chromosome arms and push the attached chromosome away from the pole (or the pole away from the chromosome) Finally, dyneins are minus-end directed motors that, together with associated proteins, organize microtubules at various locations in the cell. They link the plus ends of astral microtubules to components of the actin cytoskeleton at the cell cortex, for example; by moving toward the minus end of the microtubules, the dynein motors pull the spindle poles toward the cell cortex and away from each other. Centrosome Duplication Occurs Early in the Cell Cycle There are interesting parallels between centrosome duplication and chromosome duplication. Both use a semiconservative mechanism of duplication, in which the two halves separate and serve as templates for construction of a new half. Centrosomes, like chromosomes, must replicate once and only once per cell cycle, to ensure that the cell enters mitosis with only two copies: an incorrect number of centrosomes could lead to defects in spindle assembly and thus errors in chromosome segregation. Bi-orientation Is Achieved by Trial and Error The success of mitosis demands that sister chromatids in a pair attach to opposite poles of the mitotic spindle, so that they move to opposite ends of the cell when they separate in anaphase. Part of the answer is that sister kinetochores are constructed in a back-toback orientation that reduces the likelihood that both kinetochores can face the same spindle pole. Nevertheless, incorrect attachments do occur, and elegant regulatory mechanisms have evolved to correct them. How is this mode of attachment, called bi-orientation, achieved? What prevents the attachment of both kinetochores to the same spindle pole or the attachment of one kinetochore to both spindle poles? Trial and error system Incorrect attachments are corrected by a system of trial and error that is based on a simple principle: incorrect attachments are highly unstable and do not last, whereas correct attachments become locked in place. How does the kinetochore sense a correct attachment? The answer appears to be tension: When a sister-chromatid pair is properly bi-oriented on the spindle, the two kinetochores are pulled in opposite directions by strong poleward forces. Sisterchromatid cohesion resists these poleward forces, creating high levels of tension within the kinetochores. When chromosomes are incorrectly attached—when both sister chromatids are attached to the same spindle pole, for example—tension is low How tension might increase microtubule attachment to the kinetochore. The tension-sensing mechanism depends on the protein kinase Aurora-B, which is associated with the kinetochore and is thought to generate the inhibitory signal that reduces the strength of microtubule attachment in the absence of tension. Aurora-B phosphorylates several components of the microtubule attachment site, including the Ndc80 complex, decreasing the site’s affinity for a microtubule plus end. When bi-orientation occurs, the resulting tension somehow reduces phosphorylation by Aurora-B, thereby increasing the affinity of the attachment site Following their attachment to the two spindle poles, the chromosomes are tugged back and forth, eventually assuming a position equidistant between the two poles, a position called the metaphase plate. The initiation of sisterchromatid separation by the APC/C. After M-Cdk has triggered the complex processes leading up to metaphase, the cell cycle reaches its climax with the separation of the sister chromatids at the metaphase-to-anaphase transition the anaphase-promoting complex (APC/C) discussed earlier throws the switch that initiates sisterchromatid separation by ubiquitylating several mitotic regulatory proteins and thereby triggering their destruction. The APC/C Triggers Sister-Chromatid Separation and the Completion of Mitosis The APC/C initiates the process by targeting the inhibitory protein securin for destruction. Before anaphase, securing binds to and inhibits the activity of a protease called separase. The destruction of securin at the end of metaphase releases separase, which is then free to cleave one of the subunits of cohesin. The cohesins fall away, and the sister chromatids separate In addition to securin, the APC/C also targets the S- and Mcyclins for destruction, leading to the loss of most Cdk activity in anaphase. Cdk inactivation allows phosphatases to dephosphorylate the many Cdk target substrates in the cell, as required for the completion of mitosis and cytokinesis What activates the APC/C? APC/C activation requires binding to the protein Cdc20 phosphorylation of the APC/C helps Cdc20 bind to the APC/C, thereby helping to create an active complex. The ability of M-Cdk to promote Cdc20–APC/C activity creates a negative feedback loop: M-Cdk sets in motion a regulatory process that leads to cyclin destruction and thus its own inactivation. Chromosomes Segregate in Anaphase A and B The chromosomes move by two independent and overlapping processes: 1) The first, anaphase A, is the initial poleward movement of the chromosomes, which is accompanied by shortening of the kinetochore microtubules. 2) The second, anaphase B, is the separation of the spindle poles themselves, which begins after the sister chromatids have separated and the daughter chromosomes have moved some distance apart Summary M-Cdk triggers the events of early mitosis, including chromosome condensation, assembly of the mitotic spindle, and bipolar attachment of the sister-chromatid pairs to microtubules of the spindle. Spindle formation in animal cells depends largely on the ability of mitotic chromosomes to stimulate local microtubule nucleation and stability, as well as on the ability of motor proteins to organize microtubules into a bipolar array. Many cells also use centrosomes to facilitate spindle assembly. Anaphase is triggered by the APC/C, which stimulates the destruction of the proteins that hold the sister chromatids together APC/C also promotes cyclin destruction and thus the inactivation of M-Cdk. The resulting dephosphorylation of Cdk targets is required for the events that complete mitosis, including the disassembly of the spindle and the reformation of the nuclear envelope Cytokinesis: Final step in the cell cycle Cytokinesis: division of the cytoplasm in 2. he first visible change of cytokinesis in an animal cell is the sudden appearance of a pucker, or cleavage furrow, on the cell surface. The furrow rapidly deepens and spreads around the cell until it completely divides the cell in two. The structure underlying this process is the contractile ring—a dynamic assembly composed of actin filaments, myosin II filaments, and many structural and regulatory proteins. During anaphase, the ring assembles just beneath the plasma membrane. Local Activation of RhoA Triggers Assembly and Contraction of the Contractile Ring RhoA, a small GTPase of the Ras superfamily controls the assembly and function of the contractile ring at the site of cleavage RhoA is thought to be activated by a guanine nucleotide exchange factor (RhoGEF), which is found at the cell cortex at the future division site and stimulates the release of GDP and binding of GTP to RhoA Study Guidelines