CASA B1-11f Aeroplane Systems (Instruments/Avionics) PDF
Document Details
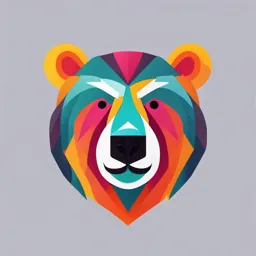
Uploaded by RapturousWendigo
2023
CASA
Tags
Summary
This document details CASA B1-11f training materials on aeroplane systems, specifically focusing on instrument systems, including pitot static and gyroscopic components. It explains fundamental concepts and components, like pressure sensing devices, altimeters, and airspeed indicators.
Full Transcript
MODULE 11A Category B1 Licence CASA B1-11f Aeroplane Systems (Instruments / Avionics) Copyright © 2020 Aviation Australia All rights reserved. No part of this document may be reproduced, transferred, sold o...
MODULE 11A Category B1 Licence CASA B1-11f Aeroplane Systems (Instruments / Avionics) Copyright © 2020 Aviation Australia All rights reserved. No part of this document may be reproduced, transferred, sold or otherwise disposed of, without the written permission of Aviation Australia. CONTROLLED DOCUMENT 2023-01-18 B1-11f Turbine Aeroplane Aerodynamics, Structures and Systems Page 2 of 356 CASA Part Part 66 - Training Materials Only Knowledge Levels Category A, B1, B2 and C Aircraft Maintenance Licence Basic knowledge for categories A, B1 and B2 are indicated by the allocation of knowledge levels indicators (1, 2 or 3) against each applicable subject. Category C applicants must meet either the category B1 or the category B2 basic knowledge levels. The knowledge level indicators are defined as follows: LEVEL 1 Objectives: The applicant should be familiar with the basic elements of the subject. The applicant should be able to give a simple description of the whole subject, using common words and examples. The applicant should be able to use typical terms. LEVEL 2 A general knowledge of the theoretical and practical aspects of the subject. An ability to apply that knowledge. Objectives: The applicant should be able to understand the theoretical fundamentals of the subject. The applicant should be able to give a general description of the subject using, as appropriate, typical examples. The applicant should be able to use mathematical formulae in conjunction with physical laws describing the subject. The applicant should be able to read and understand sketches, drawings and schematics describing the subject. The applicant should be able to apply his knowledge in a practical manner using detailed procedures. LEVEL 3 A detailed knowledge of the theoretical and practical aspects of the subject. A capacity to combine and apply the separate elements of knowledge in a logical and comprehensive manner. Objectives: The applicant should know the theory of the subject and interrelationships with other subjects. The applicant should be able to give a detailed description of the subject using theoretical fundamentals and specific examples. The applicant should understand and be able to use mathematical formulae related to the subject. The applicant should be able to read, understand and prepare sketches, simple drawings and schematics describing the subject. The applicant should be able to apply his knowledge in a practical manner using manufacturer's instructions. The applicant should be able to interpret results from various sources and measurements and apply corrective action where appropriate. 2023-01-18 B1-11f Turbine Aeroplane Aerodynamics, Structures and Systems Page 3 of 356 CASA Part Part 66 - Training Materials Only Table of Contents Instrument Systems Pitot Static (11.5.1.1) 14 Learning Objectives 14 The Atmosphere and Atmospheric Standards 15 Atmosphere 15 Atmospheric Pressure 15 Regions of the Atmosphere 16 Troposphere 17 The Standard Atmosphere 18 Pressure Sensing Devices 21 Aneroid Capsules 21 Bellows 23 Types of Bellows 25 Temperature Compensation 25 Bimetallic Strips 25 Temperature Compensating U-Shaped Bracket 26 Pitot Static Systems 29 Introduction to Pitot Static Systems 29 Pitot Probe 30 Static Ports 31 Altimeters 34 Elements of an Altimeter 34 Non-Sensitive Altimeter 35 Sensitive Altimeter 36 Barometric Adjustment Knob 38 Altimeter Q Code Settings 39 Airspeed Indicator 41 Introduction to Airspeed Indicator 41 Elements of an Airspeed Indicator 42 Airspeed Indicator Construction and Operation 43 Airspeed Indicator Markings 45 Maximum Allowable Airspeed Indicator 46 Airspeed Indicators for High-Speed Flight 47 Critical Mach Number 48 Maximum Operating Mach Number 48 2023-01-18 B1-11f Turbine Aeroplane Aerodynamics, Structures and Systems Page 4 of 356 CASA Part Part 66 - Training Materials Only Machmeter 49 Mach Airspeed Indicator 50 Vertical Speed Indicators 52 Introduction to Vertical Speed Indicators 52 Vertical Speed Indicator Construction 52 Vertical Speed Indicator Operation 53 Instantaneous Vertical Speed Indicator 55 Effects of System Blockages 57 Static Line Blockage 57 Pitot Line Blockage 58 Effects of Pitot Static System Leaks 59 Pitot Static System Precautions 60 System Precautions 60 Pitot Static System Precautions Example 61 Pitot Probe Warning 63 Static Ports 64 Pitot Static Plumbing 65 Drain Holes and Water Traps 66 Instrument Systems Gyroscopic (11.5.1.2) 68 Learning Objectives 68 Summary 68 Gyroscope 69 Introduction to Gyroscope 69 Gyroscopic Principles 70 Rigidity or Inertia 71 Precession 72 Gimbal Lock 73 Toppling 73 Terminology 75 Free or Space Gyro 75 Earth Rate 75 Transport Wander/Rate 75 Drift 76 Earth Gyro 76 Tied Gyro 76 Displacement Gyros 77 Rate Gyro 77 2023-01-18 B1-11f Turbine Aeroplane Aerodynamics, Structures and Systems Page 5 of 356 CASA Part Part 66 - Training Materials Only Gyroscope Applications in Aircraft 78 Introduction to Gyroscope Applications 78 Correction of Drift 79 Gyro Erection Systems 81 Caging 81 Aircraft Vacuum Gyro System 83 Introduction to the Vacuum Gyro System 83 Air Flow and Erection in Gyro 84 Disadvantages of Air-Driven Gyro Systems 86 Electrically-Driven Gyro 87 Introduction to Electrically-Driven Gyro 87 Electrical Gyro Construction 87 DC Gyro Systems Advantages and Disadvantages 88 AC Gyro Systems Advantages and Disadvantages 88 Gyro Limitations 89 Artificial Horizon 90 Introduction to Artificial Horizon 90 Artificial Horizon Stabilised Sky Plate 91 Attitude Director 93 Introduction to Attitude Director 93 Flight Director Components 94 Navigation Mode Select Panel 95 Flight Director Computer 96 Standby Attitude Indicator 98 Direction Indication 100 Introduction to Direction Indication 100 Heading Indicator 101 Directional Gyro and Horizontal Indicator Comparisons 101 Remote Reading Compass System 102 Horizontal Situation Indicator 104 Introduction to Horizontal Situation Indicator 104 Electronic Horizontal Situation Indicator 105 Turn-and-Slip Indicator 106 Introduction to Turn-and-Slip Indicator 106 Rate Gyro 106 Turn-and-Slip Indicator Principle of Operation 107 2023-01-18 B1-11f Turbine Aeroplane Aerodynamics, Structures and Systems Page 6 of 356 CASA Part Part 66 - Training Materials Only Air-Driven Turn-and-Slip Indicator 109 Electrically-Powered Turn-and-Slip Indicator 109 Interpretation of Rate-of-Turn Indication 110 Inclinometer or Slip-and-Skid Indicator 111 Turn Coordinator 114 Introduction to Turn Coordinator 114 Turn Coordinator Display 114 Gyro Handling 116 Introduction to Gyro Handling 116 Instrument Systems Compasses (11.5.1.3) 117 Learning Objectives 117 Magnetic Principles 118 Introduction to Magnetic Principles 118 Terrestrial Magnetism 118 The Earth’s Magnetic Field and the Magnetic Poles 118 Magnetic Variation or Declination 119 Magnetic Inclination or Dip 121 Direct-Reading Compass 123 Introduction to Direct-Reading Compass 123 Direct-Reading Compass Construction 124 Magnet Systems 124 Compass Fluid 127 Liquid Volume Compensation 127 Compass Illumination 128 Deviation 128 Direct Reading Compass Compensation 128 In-Flight Errors 130 Turning Error 131 Acceleration Error 132 Remote-Reading Compass 134 Introduction to Remote-Reading Compass 134 Flux Valve 134 Flux Valve Location 137 Slaved Gyro 138 Indicating Elements 139 Compass Controller 140 Aircraft Magnetism and Its Effect on Compasses 142 2023-01-18 B1-11f Turbine Aeroplane Aerodynamics, Structures and Systems Page 7 of 356 CASA Part Part 66 - Training Materials Only Introduction to Aircraft Magnetism and Its Effect on Compasses 142 Hard Iron 142 Soft Iron 142 Deviation Coefficients 143 Compass Compensation and Adjustment 147 Introduction to Compass Compensation and Adjustment 147 Methods of Compass Swinging 147 Remote Compass Compensation 147 Comparison Method 148 Electronic Compass Calibrator 148 Datum Compass 149 Compass Systems and Components Precautions 150 Introduction to Compass Systems and Components Precautions 150 Compass Swing Precautions 150 Compass Terminology 153 Terminology 153 Instrument Systems Other (11.5.1.4) 154 Learning Objectives 154 Angle of Attack Indication 155 Introduction to Angle of Attack Indication 155 Angle of Attack 155 Stall 156 Angle of Attack Indication 157 Angle of Attack Sensors 158 Angle of Attack Sensor Precautions 160 Stall Warning Systems 162 Stagnation Point 162 Vibrating Reed Operation 162 Vane-Operated Switch 163 Stall Warning Computer 166 Stall Avoidance Systems 168 Temperature-Measuring Systems 170 Introduction to Temperature-Measuring Systems 170 Temperature-Indicating Systems 170 Expansion Type (Liquid or Solid) 170 Bimetallic Temperature Indicator 171 Vapour-Pressure Type 172 2023-01-18 B1-11f Turbine Aeroplane Aerodynamics, Structures and Systems Page 8 of 356 CASA Part Part 66 - Training Materials Only Electrical-Type Temperature-Measuring Devices 174 Thermocouple Systems 179 Thermocouple Materials and Combinations 180 Specialised Resistance Temperature Systems 182 Radiation Type 187 Cabin Pressurisation 189 Introduction to Cabin Pressurisation 189 Cabin Altimeters 189 Altitude Alerting Systems 189 Accelerometer 190 Aircraft Clock 192 Glass Cockpit 194 Introduction to Glass Cockpit 194 In Commercial Aviation 194 General Aviation 194 Avionic Systems Auto Flight (11.5.2.1) 196 Learning Objectives 196 Flight Control 197 Axes of an Aircraft 197 Flight Control Surfaces 198 Manually Operated Flight Controls 199 Powered Flight Controls 201 Introduction to Powered Flight Controls 201 Power-Assisted Control 201 Power-Operated Control 201 Fly-by-Wire System 202 Automatic Flight Control System 203 Damping Systems 203 Artificial Feel 204 Autopilot 206 Introduction to Autopilot 206 Basic Capability 206 Closed Loop Servo System 207 Autopilot Elements 209 Sensing Elements 210 Computer 211 Command Elements 212 2023-01-18 B1-11f Turbine Aeroplane Aerodynamics, Structures and Systems Page 9 of 356 CASA Part Part 66 - Training Materials Only Flight Controller 213 Control Wheel Steering 214 Autopilot Override 215 Trim Indicator 215 Autopilot Disengage Switches 216 Flight Director Display 217 Flight Mode Annunciator 218 Output Elements 220 Autopilot Actuators 220 Electro-Pneumatic Actuator 220 Electro-Mechanical 221 AC Servomotor 223 Electro-Hydraulic Actuator 224 Torque Limiting 227 Introduction to Torque Limiting 227 Methods of Limiting 227 Autopilot Operation 229 Autopilot Engagement 229 Autopilot Modes 230 Autothrottle 233 Flight Management System 235 Avionic Systems Communication (11.5.2.2) 239 Learning Objectives 239 Intercom System 240 Sound 240 Basic Intercom System 240 Microphones 241 Speaker/Headset 244 Amplifier 245 Push to Talk 245 Aircraft Intercom System 245 Flight Interphone 246 Service Interphone 247 Cabin Interphone 248 Modes of Communication 249 Radio Transmission and Reception 251 Introduction to Radio Transmission and Reception 251 2023-01-18 B1-11f Turbine Aeroplane Aerodynamics, Structures and Systems Page 10 of 356 CASA Part Part 66 - Training Materials Only EM Waves 251 Modulation 251 Modulation Types 252 Frequency Bands 254 Aircraft Radio Communication Systems 257 HF Communications 257 Antennas 257 VHF Communications 258 UHF Communications 259 Radio Transceiver 259 Radio Control Panel 261 Electromagnetic Radiation Hazards 261 ARINC Control and Reporting System 263 Introduction to ARINC Control and Reporting System 263 Airborne ACARS Components 263 Satellite Communications 265 Introduction to Satellite Communications 265 Passenger Communications 266 Emergency Location Equipment 267 Introduction to Emergency Location Equipment 267 Emergency Locator Beacons/Transmitters 267 Underwater Locater Beacon 269 Cockpit Voice Recorder 269 Avionic Systems Navigation (11.5.2.3) 274 Learning Objectives 274 Summary 274 Navigation Principles 275 Latitude 275 Longitude 275 Plotting a Position 276 Nautical Mile 277 Knots 278 Compass Direction 279 Aviation Navigation 280 Pilotage 280 Dead Reckoning 280 Inertial Navigation System 282 2023-01-18 B1-11f Turbine Aeroplane Aerodynamics, Structures and Systems Page 11 of 356 CASA Part Part 66 - Training Materials Only Introduction to Inertial Navigation System 282 Accelerometer 282 Electronic Integrator 283 Stable Platform 284 Inertial Navigation System Components 285 Inertial Reference System 290 Introduction to Inertial Reference System 290 Ring Laser Gyro Principles 290 Doppler 291 Ring Laser Gyro 292 Inertial Reference Units 293 Radio Navigation 295 Introduction to Radio Navigation 295 Automatic Direction Finder 296 Introduction to Automatic Direction Finder 296 Loop Antenna 296 Sense Antenna Polar Diagram 297 Direction Finding Cardioid Diagram 298 Automatic Direction Finder System 300 Automatic Direction Finder Operation 302 VHF Omni-Range 305 VOR Principle of Operation 305 Aircraft VOR System Components 306 Distance Measuring Equipment 311 Introduction to Distance Measuring Equipment 311 Principles of Operation 311 Distance Measuring Equipment System Components 312 Electromagnetic Radiation Hazards 315 Instrument Landing System 317 Introduction to Instrument Landing System 317 Instrument Landing System Ground Components 317 Instrument Landing System Airborne Equipment 321 Instrument Landing System Operation 327 Global Positioning System 328 Introduction to Global Positioning System 328 GPS Position Fixing 329 Distance Measurement 331 2023-01-18 B1-11f Turbine Aeroplane Aerodynamics, Structures and Systems Page 12 of 356 CASA Part Part 66 - Training Materials Only Satellite Locations 332 Satellite Transmissions 332 Differential GPS 335 GPS Receivers 337 GPS Airborne Components 338 Radio/Radar Altimeter 343 Introduction to Radio/Radar Altimeter 343 Radar Altimeter Basic Principle 344 Components of a RADALT system 345 Electromagnetic Radiation Hazards 348 Ground Proximity Warning System 349 Introduction to Ground Proximity Warning System 349 Modes of Operation 349 Ground Proximity Warning System Function 349 Ground Proximity Warning System Operation 350 Enhanced Ground Proximity Warning System 351 Area Navigation 353 Introduction to Area Navigation 353 Area Navigation Computer 354 2023-01-18 B1-11f Turbine Aeroplane Aerodynamics, Structures and Systems Page 13 of 356 CASA Part Part 66 - Training Materials Only Instrument Systems Pitot Static (11.5.1.1) Learning Objectives 11.5.1.1 Describe the purpose and operating principles of pitot static instrument systems including altimeter, airspeed indicator and vertical speed indicator (Level 2). 11.5.1.1.1 Describe the basic composition of the regions of Earth's atmosphere with respect to gas composition, pressure, and temperature (S). 11.5.1.1.2 Recall the effects of blockages and leaks to pitot static sensing lines or ports (S). 11.5.1.1.3 Recall the safety precautions relating to maintenance on or around pitot static instrument systems (S). 2023-01-18 B1-11f Turbine Aeroplane Aerodynamics, Structures and Systems Page 14 of 356 CASA Part Part 66 - Training Materials Only The Atmosphere and Atmospheric Standards Atmosphere Air is a mixture of gases in the following proportions (% by volume): Composition of atmospheric gases 2023-01-18 B1-11f Turbine Aeroplane Aerodynamics, Structures and Systems Page 15 of 356 CASA Part Part 66 - Training Materials Only Atmospheric Pressure The atmosphere consists of a layer of air many kilometres thick. The weight of the atmosphere on the earth’s surface causes an air pressure of about 14.7 psi. This pressure progressively reduces with height (altitude) above the earth. Atmospheric pressure 2023-01-18 B1-11f Turbine Aeroplane Aerodynamics, Structures and Systems Page 16 of 356 CASA Part Part 66 - Training Materials Only Regions of the Atmosphere The atmosphere is classified into four regions based on the variation of temperature with altitude. Aircraft fly only in the troposphere and the lowest part of the stratosphere. Civil aircraft would rarely exceed altitudes of 45 000ft (nearly 14 km). Note that in English-speaking countries that have adopted the metric system of measurements, aircraft altitude is still measured in units of feet. Atmospheric regions 2023-01-18 B1-11f Turbine Aeroplane Aerodynamics, Structures and Systems Page 17 of 356 CASA Part Part 66 - Training Materials Only Troposphere The troposphere is the lowest layer of the atmosphere. All of the world’s weather is contained in the troposphere. Above that, conditions remain relatively constant. It extends from sea level to the tropopause. From the Earth’s surface, the temperature falls as altitude increases. This process continues up to a height where the temperature stabilises at about -56 °C. The tropopause is the height where this occurs. Air in contact with the earth is heated by conduction and radiation. As air heats, its density decreases. Decreased density causes air to rise; reduced pressure allows air to expand and cool as it rises. Air temperature and altitude Virtually all weather phenomena take place in the troposphere. The temperature in this region decreases rapidly with altitude, clouds form (due to water vapour) and there may be much turbulence because of variations in temperature, density and pressure. It is inherently unstable and constantly changing. 2023-01-18 B1-11f Turbine Aeroplane Aerodynamics, Structures and Systems Page 18 of 356 CASA Part Part 66 - Training Materials Only The Standard Atmosphere Since the temperature, pressure and density of the atmosphere varies from place to place and from day to day, it is necessary to have a standard set of conditions with which observed readings can be compared. This facilitates the correction of aircraft and engine performance calculations for any off- standard conditions. The set of standard conditions is usually known as the International Standard Atmosphere (ISA). In the aircraft industry, this is also known as the ICAO Standard Atmosphere. ICAO stands for International Civil Aviation Organisation. International standard atmosphere The amount of pressure change the pitot static instruments are required to measure is in the region of only around 10 psi overall, so the detecting elements are very sensitive. That 10 psi is the difference between sea level and approximately 40 000 feet, which translates to 40 revolutions of a 100-foot pointer on an altimeter, which again translates to about 4 revolutions per 1 psi. Therefore, if a sharp increase or decrease in pressure were applied to a pitot probe or static vent, pitot static instruments would be severely damaged and rendered unusable. At 10 000 feet, atmospheric pressure is ⅔ that of the pressure at sea level (10.1 psia). At 18 000 feet, atmospheric pressure is ½ that of the pressure at sea level (7.3 psia). 2023-01-18 B1-11f Turbine Aeroplane Aerodynamics, Structures and Systems Page 19 of 356 CASA Part Part 66 - Training Materials Only In the year 1643, Italian mathematician Evangelista Torricelli found that the pressure of the air bearing down on the liquid in the container forced it up the tube, and the measurement of the various lengths of the column of liquid was therefore a means of expressing the changes in air pressure. In order to have a tube of a manageable length, the heaviest of all liquids, mercury, was later used. Mercury barometers can give very accurate readings. They are costly and need special care in handling. The pressure of the atmosphere forces the mercury 29.92 inches (76 cm) up an inverted tube sealed at one end. The space above the mercury is a vacuum. As atmospheric pressure increases, the pressure is felt around the surface of the mercury in the bowl at the bottom, and the increased pressure forces the mercury higher up the tube. As atmospheric pressure decreases, less force is applied to the mercury column, so it drops lower in the tube. Measuring how high the mercury is suspended in the tube provides us with an indication of the atmospheric pressure. Mercury barometer If, for example, water was used in place of mercury, the tube would have to be much longer, as the water would be pushed up the tube to about 34 feet by the atmospheric pressure. A mercury barometer is not practical to mount in an aircraft, so another method is needed to measure atmospheric pressure. 2023-01-18 B1-11f Turbine Aeroplane Aerodynamics, Structures and Systems Page 20 of 356 CASA Part Part 66 - Training Materials Only Pressure Sensing Devices Aneroid Capsules For pressure measurements in an aircraft, it is standard practice to use elastic pressure-sensing elements, in which forces produced by applied pressures can be made to actuate mechanical and/or electrical indicating elements. Aneroid capsules measure very small changes in pressure with reference to a vacuum. Their maximum operating range is 0 psia or a vacuum up to about 17 psia. Aneroid stack They work as a push-pull arrangement, producing an output which is applied to linkages and gears driving an instrument pointer. The sense element of an aneroid barometer consists of an evacuated metal capsule, called an aneroid capsule; they are used to measure absolute pressure. As there is zero pressure inside the capsule and atmospheric pressure on the outside of the capsule, a strong leaf spring prevents its total collapse. As pressure around the outside of the capsule increases, it tends to compress the capsule against the spring pressure. As pressure decreases, for example, with a rise in altitude, the capsules opposition to the spring pressure lessens, so it tends to expand. Aneroid capsule 2023-01-18 B1-11f Turbine Aeroplane Aerodynamics, Structures and Systems Page 21 of 356 CASA Part Part 66 - Training Materials Only This type of aneroid can be calibrated to indicate barometric pressure, or by changing the scale markings to feet and extending the range of capsule movement, the aneroid barometer becomes an altimeter capable of indicating the height of an aircraft above sea level. While most aneroid capsules have all the air evacuated, some do contain a specific pressure of inert gas to help improve stability. Instruments can also use a few aneroid capsules, arranged in a stack format, which increases sensitivity and improves accuracy. Aneroid capsule stack inside instrument mounting frame The very small expansion and contraction needs magnification. The capsule will expand or contract around 0.01 mm for each millibar of pressure change. That means for an ascent from sea level to 30 000 ft, the capsule will expand around 7 mm. This linear movement needs to be transferred into rotary movement, using pivoted levers, sector gears and maybe a flexible cord, or very fine linked fusee chain to position a pointer over a dial. Altimeter cutaway showing aneroid capsule and instrument mechanism 2023-01-18 B1-11f Turbine Aeroplane Aerodynamics, Structures and Systems Page 22 of 356 CASA Part Part 66 - Training Materials Only Aneroid capsules used in aircraft to measure atmospheric pressure altitude and parameters with respect to a vacuum, or absolute pressure. Aneroid capsules are the basis of the following: Barometers Altimeters Manifold pressure gauges. A stack of aneroid capsules increases the sensitivity of the instrument, therefore improving accuracy. Capsules may be either evacuated (aneroid) or filled with a specific pressure of inert gas and exposed to the pressure to be measured. Aneroid capsules stack altimeter 2023-01-18 B1-11f Turbine Aeroplane Aerodynamics, Structures and Systems Page 23 of 356 CASA Part Part 66 - Training Materials Only Bellows Principle of Operation A bellows operates similar to an aneroid capsule except a bellows is generally not evacuated and is manufactured from more rugged material. An increase in pressure expands a bellows. A bellows type of element can be considered an extension of the corrugated diaphragm principle, and in operation, it bears some resemblance to a helical compression spring. It may be used for high, low or differential pressure measurement, and in some applications, a spring may be employed (internally or externally) to increase what is termed the ‘spring-rate’ and to assist a bellows to return to its natural length when pressure is removed. Differential bellows assembly The element is made from a length of seamless metal tube with suitable end fittings for connection to pressure sources or for hermetic sealing. By connecting two bellows, the centre point varies dependent upon pressure applied to each bellows. This works as a push-pull arrangement, producing an output which is applied to linkages and gears driving an instrument pointer. 2023-01-18 B1-11f Turbine Aeroplane Aerodynamics, Structures and Systems Page 24 of 356 CASA Part Part 66 - Training Materials Only Bellows can be used to measure high, low and differential pressures. Types of Bellows Differential Two bellows fed from different sources will create movement at the centre proportional to pressure difference. Sylphon One bellows is fed from a pressure source, and the other is evacuated (aneroid bellows). The resulting differential pressure is expressed as absolute pressure or psia. Differential pressure references a vacuum. This is where psia and psid overlap. Also, psig can be termed psid because the pressure measured is the difference between atmospheric and the pressure under test. In reality, consider what pressures are being monitored and what is used as a reference. A manifold pressure gauge is a typical example of a Sylphon-type bellows: an evacuated bellows is used to reference measured pressure to a vacuum. Temperature Compensation Like all substances, instrument sensing elements are affected by temperature changes. In a cold climate, an aneroid capsule would not be as malleable, so it would not expand and contract as readily as in a hot climate; therefore, the instrument readings will be influenced by temperature and not the pressure they are intended to measure. Temperature errors can occur at sea level between hot and cold days but will also be induced by altitude. Instruments’ movements must compensate for temperature changes. 2023-01-18 B1-11f Turbine Aeroplane Aerodynamics, Structures and Systems Page 25 of 356 CASA Part Part 66 - Training Materials Only Bimetallic Strips Two metals with differing temperature coefficients of expansion are bonded together, for example, brass and invar. Brass has a high coefficient of expansion, while invar has a low coefficient of expansion. At some datum temperature, the strip is straight. If the strip is heated, the brass expands more than the invar, which causes it to curl. If the strip is cooled, the brass contracts more than the invar, which causes it to curl the opposite way. Bimetallic strips and discs Bimetallic temperature sensors are used in temperature, liquid level and position indicators as temperature compensators or correctors in various instruments and mechanisms and to operate switch contacts in circuit breakers, fire detectors, thermostats and timers. 2023-01-18 B1-11f Turbine Aeroplane Aerodynamics, Structures and Systems Page 26 of 356 CASA Part Part 66 - Training Materials Only Temperature Compensating U-Shaped Bracket The pressure-sensing element of an altimeter compensates for changes in ambient temperature with a bimetallic U-shaped bracket, the open ends of which are connected to the top capsule by push rods. The temperature coefficient of the instrument is chiefly due to the change of elasticity of the capsule material with change of temperature. This in turn varies the degree of deflection of the capsule in relation to the pressure acting external to it. For example, if at sea-level the temperature should decrease, the elasticity of the capsule would increase; in other words, and from the definition of elasticity, the capsule has a greater tendency ‘to return to its original size’ and thus would expand and cause the altimeter to over-read. Aneroid capsule temperature compensation The effect of a decrease in temperature on the U-shaped bracket is to cause the limbs to bend inwards, and by the angular position of the pins, a corresponding downward force is exerted on the capsule assembly to oppose the error-producing expansion. The converse of the foregoing sequence will apply when an increase of ambient temperature occurs. Bimetallic U bracket temperature compensation 2023-01-18 B1-11f Turbine Aeroplane Aerodynamics, Structures and Systems Page 27 of 356 CASA Part Part 66 - Training Materials Only The bimetallic U bracket strip will not be affected by pressure change, so it will not oppose any capsule movement produced by a change in pressure but will simply act as a spring. 2023-01-18 B1-11f Turbine Aeroplane Aerodynamics, Structures and Systems Page 28 of 356 CASA Part Part 66 - Training Materials Only Pitot Static Systems Introduction to Pitot Static Systems The system of ports and tubing on the aircraft which supplies pitot and static pressure for the instruments is called the pitot static system. The pitot tube is an open tube which faces forward into the relative airflow in flight. It measures the ram pressure of the airstream. The static ports are openings at right angles to the relative airflow so that they will measure static pressure and not be affected by the speed of the aircraft. The static ports are most often located in pairs along the sides of the fuselage. On some aircraft, the static ports are along the sides or top and bottom surfaces of the pitot tube so that both pressures are measured with the same probe. A pitot/static tube typically includes static ports and electric heaters to prevent icing. This style of pitot probe will be covered later. Aircraft pitot static system 2023-01-18 B1-11f Turbine Aeroplane Aerodynamics, Structures and Systems Page 29 of 356 CASA Part Part 66 - Training Materials Only Basic pitot static system Pitot Probe A pitot probe in its simplest format consists of an open end of a tube projecting into the airstream. When the airstream comes to rest within the tube, it creates additional pressure called pitot pressure. Aircraft pitot tube 2023-01-18 B1-11f Turbine Aeroplane Aerodynamics, Structures and Systems Page 30 of 356 CASA Part Part 66 - Training Materials Only Pitot probe location The simple tube had a few disadvantages. Moisture in the airstream could collect in the tube and cause erroneous airspeed readings. A small calibrated drain hole allows this moisture to escape without affecting the sensed pressure. Any moisture has the potential to freeze, so a powerful electrical heater element negates this possibility. 2023-01-18 B1-11f Turbine Aeroplane Aerodynamics, Structures and Systems Page 31 of 356 CASA Part Part 66 - Training Materials Only Static Ports The detecting element used to measure static pressure is termed a static port. Aircraft may use static ports either incorporated in other detecting elements or individually as a static vent in the side of the fuselage. In some light aircraft, the vent may simply be a hole drilled in the fuselage skin, while for more complex aircraft systems, the skin has specially contoured metal vent plates mounted. The altimeter static port plumbing can be used to provide static pressure to the airspeed indicator. Static port construction Static port on fuselage 2023-01-18 B1-11f Turbine Aeroplane Aerodynamics, Structures and Systems Page 32 of 356 CASA Part Part 66 - Training Materials Only Static slots as part a pitot probe Independent static vents, when fitted, are always located in the skin of a fuselage, one on each side. They interconnect via a common manifold to average and minimise dynamic pressure effects due to yawing or sideslip of the aircraft. Static Port Precautions For aircraft that use fuselage-mounted static ports, normally an area around them is free of paint. It is important that this area is kept clean and free of scratches and other rough surfaces as airflow over these may cause pressure errors. The two screws in the unpainted area are for attachment of a test adaptor to allow testing of the static system. The screws must always be in position. 2023-01-18 B1-11f Turbine Aeroplane Aerodynamics, Structures and Systems Page 33 of 356 CASA Part Part 66 - Training Materials Only Altimeters Elements of an Altimeter An altimeter measures the prevailing atmospheric pressure, which surrounds the aircraft, and displays this pressure in units of feet above sea level. © Jeppesen Aneroid altimeter Elements of an altimeter 2023-01-18 B1-11f Turbine Aeroplane Aerodynamics, Structures and Systems Page 34 of 356 CASA Part Part 66 - Training Materials Only Detecting — Static vent or static chambers on a pitot/static probe Measuring — Aneroid Capsule or stack of aneroid capsules Coupling — Lever system Indicating — Sector gears, magnification gearing, pointer and dial.. Non-Sensitive Altimeter An aneroid capsule assembly mounts on a frame contained within a leak-tight case. The case has a single port at the rear, which connects to the aircraft’s static system. Changes in static pressure alter the capsule’s linear dimension. This change is magnified and converted to rotary motion using a sector and pinion assembly and drives a pointer over a scale marked in feet. Non-sensitive altimeter A non-sensitive altimeter only has one pointer which makes one complete revolution for each 10 000 ft in altitude. Non-sensitive altimeters are typically only used in small simple aircraft that don’t operate at high altitudes. 2023-01-18 B1-11f Turbine Aeroplane Aerodynamics, Structures and Systems Page 35 of 356 CASA Part Part 66 - Training Materials Only Altimeter face An example of the use of a non-sensitive altimeter is in a pressurised aircraft where it displays the height of the cabin, in thousands of feet, above sea level. In this situation, the interior of the altimeter casing can sense cabin pressure and not the outside static pressure. Cabin altimeter 2023-01-18 B1-11f Turbine Aeroplane Aerodynamics, Structures and Systems Page 36 of 356 CASA Part Part 66 - Training Materials Only Sensitive Altimeter Altimeters with two or three pointers or with a single pointer and a drum counter and a means to correct for local pressure deviations from International Standard Atmosphere (ISA) are sensitive altimeters. The internal mechanism of the sensitive altimeter consists of three diaphragms in series. This creates an altimeter which is sensitive to very small changes in pressure and which has a large enough range of measurement to read altitudes of 35 000 to 50 000 feet or more. Capsule movement is magnified and converted to rotary motion to drive a series of three pointers. Inside the case of the altimeter is a bimetallic device which gives automatic compensation for temperature changes to ensure accurate readings. There is a knob on the front of the altimeter which operates a mechanism that compensates for non-standard atmospheric pressure. © Jeppesen Sensitive altimeter The dial face has 10 equally spaced marks numbered 0 to 9. The larger pointer, known as the 100-foot pointer, rotates once around the dial for each 1000-foot change in altitude, so each segment represents 100 feet. The smaller second pointer, known as the 1000-foot pointer, will rotate once around the dial every 10 000 feet, so each segment represents 1000 feet. The third pointer, the 10 000-foot pointer or trace pointer, often of varying design, serves to eliminate ambiguity created by the second pointer. Here each segment represents 10 000 feet. This pointer might also incorporate a striped disc, or cross hatch flag, which will fully display in a window from sea level to 10 000 feet. Between 10 000 feet and 16 000 feet, the striped disc will gradually retract from view. The purpose of this display is to serve as a passive low-altitude warning device. 2023-01-18 B1-11f Turbine Aeroplane Aerodynamics, Structures and Systems Page 37 of 356 CASA Part Part 66 - Training Materials Only Sensitive Altimeter Operation The aneroid capsules of an altimeter are sealed at the factory. The case of the altimeter is connected to the static ports on the aircraft. The static pressure outside the aircraft is conducted to the instrument case by tubing and hose. If the aircraft climbs, the reduced pressure in the case causes the aneroid capsules to expand and move the pointers to indicate a higher altitude. The mechanism uses very delicate gearing which is designed in such a way that a very small movement of the diaphragm causes a large motion of the pointer or pointers. Under certain circumstances, it tends to stick, and some aircraft have a vibrator on the instrument panel or in the altimeter to prevent this sticking. Barometric Adjustment Knob Barometric scale and adjustment knob Altimeters are calibrated to the standard atmosphere, but because local conditions are rarely standard, they have a ready means of adjusting the mechanism to allow for local variations. This is done by a knob that sets the altimeter’s barometric correction mechanism and dial to the local sea- level pressure. Both types of sensitive altimeter have the provision to display and set the prevailing pressure allowing the altimeter to read 0 feet at sea level when corrected for local sea level pressure. The barometric pressure setting, displayed in millibars and/or inches of mercury through a window on the dial, responds to a barometric pressure-setting knob on the front of the case. Turning the knob alters the barometric pressure setting display and the altitude reading. A sensitive altimeter is critical for maintaining safe vertical separation between aircraft and terrain, from take-off, enroute and until touch down. The ability to set the pressure reading on an altimeter forms an important part of flight operating techniques. 2023-01-18 B1-11f Turbine Aeroplane Aerodynamics, Structures and Systems Page 38 of 356 CASA Part Part 66 - Training Materials Only Atmospheric pressure information relayed to pilots by air traffic control is part of the ‘Q’ code of communication. With all altimeters in a specific area set at the same barometric pressure setting, pilots have confidence of their terrain clearance and their ability to maintain vertical separation with other aircraft. 2023-01-18 B1-11f Turbine Aeroplane Aerodynamics, Structures and Systems Page 39 of 356 CASA Part Part 66 - Training Materials Only Altimeter Q Code Settings QFE The QFE code refers to setting the barometric pressure prevailing at an airfield to make the altimeter read zero feet when on the ground at that airfield. This is regardless of the airfield’s elevation above sea level. QNH The QNH code refers to setting the barometric pressure to make the altimeter read airfield elevation above sea level on landing and take-off. The pressure set is a value reduced to mean sea level in accordance with ISA. The setting is only valid near the airfield and is useful for determining terrain clearance. Enroute navigation will require QNH updates as they apply to the region the aircraft is transiting. Engineers are interested in QNH, as together with outside air temperature and plotted on a chart, it is possible to calculate the density altitude figure required to determine engine performance. QNE The QNE code refers to the barometric setting on the altimeter of the ISA sea level value of 1013.25 millibars (hPa). The QNE code applies whenever an aircraft flies above the ‘transition altitude,’ which is an altitude set by traffic control authorities. Above the ‘transition altitude,’ altitudes become flight levels. For example, 15 000 feet becomes FL150. Altimeter pressure settings 2023-01-18 B1-11f Turbine Aeroplane Aerodynamics, Structures and Systems Page 40 of 356 CASA Part Part 66 - Training Materials Only Airspeed Indicator Introduction to Airspeed Indicator An airspeed indicator provides an indication of an aircraft’s speed through the surrounding air. Indicated airspeed is a measure, displayed in knots (nautical miles per hour), of the difference between the dynamic pressure (known as pitot pressure) and static pressure with regard to airflow. Airspeed indicator Capsule assembly 2023-01-18 B1-11f Turbine Aeroplane Aerodynamics, Structures and Systems Page 41 of 356 CASA Part Part 66 - Training Materials Only The pressures are equal when the aircraft is stationary on the ground. However, movement of the aircraft through the air causes the dynamic pressure to become greater than that in the static pressure. The airspeed indicator provides important information for the pilot to be able to maintain control of the aircraft. With too low an airspeed, the aircraft will stall, and with too high an airspeed, the aircraft will suffer from structural damage. As such, airspeed equates to the aerodynamic loading of the aircraft. Airspeed is not a value measured to facilitate navigation or to calculate an estimated time of arrival at a destination, as an airspeed indicator does not provide an indication of ground speed. Difference between indicated airspeed and ground speed An aircraft could be flying with a tailwind of 70 knots and airspeed of 100 knots. This means the aircraft effectively has a groundspeed of around 170 knots. Conversely, if an aircraft was flying into a 70-knot headwind doing 100 knots, its ground speed would effectively be only 30 knots. Although time taken to reach a destination would vary greatly in the two above examples, the aircraft’s aerodynamic loading or flying characteristics would be the same for both examples. 2023-01-18 B1-11f Turbine Aeroplane Aerodynamics, Structures and Systems Page 42 of 356 CASA Part Part 66 - Training Materials Only Elements of an Airspeed Indicator Airspeed indicator elements Detecting – Pitot probe and Static vents Measuring – Differential Capsule or stack of differential capsules Coupling – Lever system Indicating – Sector gears, magnification gearing, pointer and dial. 2023-01-18 B1-11f Turbine Aeroplane Aerodynamics, Structures and Systems Page 43 of 356 CASA Part Part 66 - Training Materials Only Airspeed Indicator Construction and Operation An airspeed indicator consists of a cylindrical airtight case, connected to the static line of the aircraft’s pitot static system. Inside the case, a capsule assembly made of phosphor bronze or beryllium copper mounts onto the instrument frame. A capillary tube connects the capsule’s interior to the pitot line of the aircraft’s pitot static system through a leak tight connection on the back of the instrument case. The capsule expands in response to the aircraft’s forward movement, and through levers, a rocking shaft and a sector gear and pinion assembly position the airspeed pointer over the scale. As aircraft increases in altitude, static pressure decreases; therefore, less pitot pressure is required to expand the capsule than would be required at sea-level, so the instrument readout is referenced to atmospheric pressure, providing an indication of differential pressure between pitot and static. Airspeed indicator construction The coupling element between the measuring and the indicating elements contains a temperature compensating ranging lever. This corrects for changes in the capsule’s elasticity with changes in temperature. An increase in temperature on the capsule material makes it more flexible so that it will expand further to move the ranging lever in the direction indicated by the solid arrows. As the ranging bar is in contact with the sector gear arm, the indicating element has the tendency to over-read. However, the increase of temperature has a simultaneous effect on the ranging bar, which, being of bimetal construction and because of the position of the invar portion, will deflect in the direction indicated by the dotted arrow, thus counteracting the capsule expansion and keeping the indicating element at a constant reading. 2023-01-18 B1-11f Turbine Aeroplane Aerodynamics, Structures and Systems Page 44 of 356 CASA Part Part 66 - Training Materials Only Airspeed indicator temperature compensation Airspeed Indicator Markings Airspeed indicator As an aid to pilots, an airspeed indicator fitted to a low-speed, low-altitude aircraft has various limitation markings painted on the dial. These markings are specific for the model of aircraft. A red radial line is the never exceed speed and refers to the maximum speed limited by structural considerations of the airframe. The acronym Vne refers to this limitation. 2023-01-18 B1-11f Turbine Aeroplane Aerodynamics, Structures and Systems Page 45 of 356 CASA Part Part 66 - Training Materials Only The stall speed, indicated by the low-speed end of the green or white ranges, is dependent on the configuration of the flaps and/or landing gear. The amber caution speed range would require smooth turbulent-free conditions to enable safe operation of the aircraft while the green range constitutes the normal operating speed of the aircraft. Maximum Allowable Airspeed Indicator Maximum allowable airspeed indicator For aircraft that can achieve high cruise altitudes, it is necessary to use a modified type of airspeed indicator instead of the conventional type mentioned above. As the aircraft enters the higher altitudes, there is a preference of using Mach instead of airspeed as the reference Vne (velocity — never exceed). On the maximum allowable airspeed Indicator, a red and white striped barber’s pole, driven by an aneroid bellows assembly, replaces the fixed red line. The calibration of the barber’s pole relates directly to the Vne for that particular aircraft type. As the aircraft climbs above about 23 000 feet, the barber’s pole starts to reduce the Vne to avoid the onset of transonic shock waves that can destroy lift generated on the wing. From this point on, the aircraft’s speed limitation becomes the Maximum Operating Mach Number (Mmo). 2023-01-18 B1-11f Turbine Aeroplane Aerodynamics, Structures and Systems Page 46 of 356 CASA Part Part 66 - Training Materials Only The relationship between the speed of sound and altitude On the indicator for a Beechcraft model, the barber’s pole is at 265 knots. This means that at lower altitudes, the aircraft’s Vne is 265 knots. The ISA speed of sound at sea level is 661.7 knots, and 265 knots is 40% of this speed. Therefore, when this aircraft operates at high altitudes, the Mmo will be Mach 0.4. For example, when the aircraft is at 30 000 feet, the barber’s pole will indicate a Mmo equivalent of 236 knots, and by 35 000 feet, the barber’s pole will be at 230 knots. 2023-01-18 B1-11f Turbine Aeroplane Aerodynamics, Structures and Systems Page 47 of 356 CASA Part Part 66 - Training Materials Only Airspeed Indicators for High-Speed Flight With the advent of the gas turbine, the propulsive power available made it possible to attain greater flying speeds. For aircraft flying near, at or beyond the speed of sound, in-flight behaviour becomes a crucial cause for concern due to the characteristics exhibited by airflow over an aerofoil. Safe flight at high speed depends on the aircraft’s aerodynamic profile and structural design overcoming, or minimising, the limiting effects of high velocity airflow and its associated forces. Since the speed of sound depends on atmospheric temperature and air density, suitable computation of pressure measurements can express the ratio of the speed of the aircraft V with the speed of sound α, with the resultant presented in a conventional manner. The ratio V/α, where V is the aircraft’s true airspeed and α is the speed of sound at the aircraft’s altitude, is known as ‘Mach number’ (M) and is displayed by a Machmeter. As a parameter, it has assumed great significance in practical aerodynamics. As the aircraft approaches the speed of sound, the air accelerating over the top of the wing surface will make that air closer to the speed of sound. A shock wave begins to form which disrupts the smooth airflow, causing the centre of pressure to start to shift aft. Critical Mach Number Critical Mach Number (Mcrit) is that Mach number at which supersonic airflow first occurs, at any point on the airframe of a specific aircraft type. Commercial jet aircraft will reach Mcrit at about M.73. Critical Mach number 2023-01-18 B1-11f Turbine Aeroplane Aerodynamics, Structures and Systems Page 48 of 356 CASA Part Part 66 - Training Materials Only Maximum Operating Mach Number To avoid potential loss of control of a subsonic aircraft, a Maximum Operating Mach Number (Mmo) value is determined so that at high altitudes there is a suitable safety margin prior to the increasing disruption caused by shock waves reaching a point where there is a risk of a high-speed stall or loss of control of the aircraft. Most commercial jet aircraft have a Mmo of about M.8 to M.87. Machmeter A Machmeter is a compound instrument that accepts two variables, airspeed and altitude, using them to compute the required ratio. The indicator has a pre-adjusted lubber mark located over the dial of the indicator which shows the Mcrit and Mmo for the aircraft to which it is fitted. The scale is marked in decimal points of Mach 1. Machmeter The first variable is airspeed, and therefore a mechanism based on the conventional airspeed indicator measures this in terms of the pressure difference p-s, where p is the total or pitot pressure and s the static pressure. The second variable is altitude, and an aneroid capsule sensitive to static pressure provides this information. Deflections of the capsules of both mechanisms drive the indicator pointer through a series of rocking shafts and levers, with the dividing function of the altitude unit accomplished by an intermediate sliding rocking shaft. The altimeter mechanism is calibrated to QNE and modifies the magnification of the airspeed capsules output. Although the speed of sound is variable with respect to temperature, the altitude measurement provides the equivalent temperature in accordance with the ISA chart previously shown. 2023-01-18 B1-11f Turbine Aeroplane Aerodynamics, Structures and Systems Page 49 of 356 CASA Part Part 66 - Training Materials Only Machmeter mechanism 2023-01-18 B1-11f Turbine Aeroplane Aerodynamics, Structures and Systems Page 50 of 356 CASA Part Part 66 - Training Materials Only Mach Airspeed Indicator To save space on the panel, it is possible to combine a Machmeter and airspeed indicator into one instrument. The use of pneumatic power, and/or servomotors using amplified signals from an air data computer, drives the indicator. The indicator below operates pneumatically with the airspeed capsule driving the airspeed pointer and the aneroid capsule driving the Mach disc. Currently, this indicator shows airspeed of 425 knots and Mach.7, with the Mmo red line at.826. The knob on the front can position the two bugs on the perimeter of the dial. Mach airspeed indicator (MASI) 2023-01-18 B1-11f Turbine Aeroplane Aerodynamics, Structures and Systems Page 51 of 356 CASA Part Part 66 - Training Materials Only Vertical Speed Indicators Introduction to Vertical Speed Indicators A Vertical Speed Indicators (VSI) or Rate of Climb (ROC) indicator is a very sensitive differential pressure gauge designed to indicate the rate of altitude change by measuring variations in static pressure. The mechanism drives a pointer over a scale calibrated in Feet per Minute (FPM). The scale has a zero FPM position at the 9 o’clock position, and clockwise rotation from this point shows as a climbing rate of change. Anticlockwise movement of the pointer from zero indicates a descending rate of change. The scale graduation is often nonlinear, with the scale more open near the zero point so that minor altitude rate changes are presented with better readability. Depending on the performance characteristics of the aircraft, the VSI fitted will have a range normally at 2000, 4000 or 6000 feet per minute. Vertical speed indicator (VSI) 2023-01-18 B1-11f Turbine Aeroplane Aerodynamics, Structures and Systems Page 52 of 356 CASA Part Part 66 - Training Materials Only Vertical Speed Indicator Construction The mechanism, housed in a leak tight case, connects to the static pressure system of the aircraft. Inside the case, the measuring element consists of a capsule sensing element and metering unit, a coupling element consisting of a rocking shaft and sector gear and an indicating element of a pinion and pointer assembly. The metering unit vents static pressure to the interior of the case through a calibrated leak. A capillary tube supplies static pressure to the inside of the capsule. Vertical speed indicator construction 2023-01-18 B1-11f Turbine Aeroplane Aerodynamics, Structures and Systems Page 53 of 356 CASA Part Part 66 - Training Materials Only Vertical Speed Indicator Operation During straight and level flight, the static pressure is constant and there is no movement of air through the metering unit orifice, or change of pressure inside the capsule. The pointer is at the 0 feet per minute position on the dial. Vertical speed indicator – level flight When the aircraft climbs, the pressure inside the capsule decreases to a value less than that inside the instrument case. The differential pressure causes the capsule to compress, causing the lever link to rotate the rocking shaft and sector gear and rotating the pinion and pointer, which indicates a climb condition. At the same time, the pressure inside the case can move through the metering unit into the static system but at a much slower rate. Calibration of the leak rate, adjusted to maintain a difference between the pressure inside the capsule and that inside the case, is proportional to the rate of change of the outside air pressure. As the aircraft levels off, the differential pressure between the inside of the case and the inside of the capsule reduces until it equalises, causing the pointer to return to the zero position. 2023-01-18 B1-11f Turbine Aeroplane Aerodynamics, Structures and Systems Page 54 of 356 CASA Part Part 66 - Training Materials Only Vertical speed indicator – aircraft climbing During descent, the flow of static air is reversed, causing the capsule to expand as the pressure in the case is now less than that inside the capsule. The resulting deflection of the capsule results in the pointer being positioned over the descent section of the scale. Vertical speed indicator – aircraft descending 2023-01-18 B1-11f Turbine Aeroplane Aerodynamics, Structures and Systems Page 55 of 356 CASA Part Part 66 - Training Materials Only Instantaneous Vertical Speed Indicator The normal VSI has a great deal of lag. It takes several seconds for them to adjust to changes in static pressure to indicate the climb or descent rate. This is a problem in helicopters because the descent rate changes rapidly on approaches to landing, and if the lag is too long, the instrument is displaying out-of-date information. An Instantaneous Vertical Speed Indicator (IVSI) consists of the same basic elements of a VSI, but in addition, they employ an accelerometer unit, the design of which helps to create a more rapid differential pressure effect, especially at the initiation of a climb or descent. The result is a faster indication that the aircraft has changed its rate of climb or descent. The accelerometer consists of a small cylinder or dashpot, containing a piston held in balance by a spring and its own mass. The cylinder, connected to the capillary tube leading to the capsule, is open directly to the static pressure source. When a change in vertical speed occurs initially, the piston displaces under the influence of the vertical acceleration force, creating an immediate pressure change inside the capsule with a corresponding instantaneous response in the indicator pointer. For example, at initiation of a descent, the piston moves up and thereby decreases the volume of the chamber above the piston, resulting in an immediate increase of pressure inside the capsule. The accelerometer response decays after a few seconds, but by this time, the change in actual static pressure becomes effective so that the pressure differential produced by the metering unit performs in the conventional manner. Instantaneous vertical speed indicator (IVSI) construction 2023-01-18 B1-11f Turbine Aeroplane Aerodynamics, Structures and Systems Page 56 of 356 CASA Part Part 66 - Training Materials Only Effects of System Blockages Static Line Blockage Blockages can occur to either the pitot or the static lines by the ingress of water that can also freeze, kinks in the plumbing or by insects, for example, wasps, gaining access through unprotected ports while the aircraft is on the ground. The example below highlights the result when the static line becomes blocked. The static line provides information to all three instruments, and if the aircraft changes altitude after the time the blockage occurs, there will be no movement of the altimeter or vertical speed pointers. The effect of a blocked static line on the airspeed indicator is more complex. If an aircraft increases altitude, the airspeed indication will decrease, and the airspeed indication will increase as the aircraft reduces altitude. Refer to the upcoming topic on airspeed indicators to gain a better understanding as to why this happens. As the pressures experienced by the instruments are extremely low, it is unlikely that even a small amount of water will clear itself. Static line blockage A partial blockage or restriction in the static line will cause the following: A sluggish response from the altimeter A degraded, or no response, from the vertical speed indicator Providing the airspeed remains constant, the airspeed indicator will overread when the aircraft descends, and underread when climbing. 2023-01-18 B1-11f Turbine Aeroplane Aerodynamics, Structures and Systems Page 57 of 356 CASA Part Part 66 - Training Materials Only Static line partial blockage For aircraft fitted with an alternate static port, the selection of ‘alternate’ could bypass the blockage and allow the instruments to provide accurate indications again. Static line alternate source 2023-01-18 B1-11f Turbine Aeroplane Aerodynamics, Structures and Systems Page 58 of 356 CASA Part Part 66 - Training Materials Only Pitot Line Blockage Pitot tube blockages prevent the accurate indications of airspeed but have no effect on the altimeter or vertical speed indicator. After a blockage has occurred, a change in the aircraft’s speed will fail to display on the indicator. Changes to the aircraft’s altitude causes the airspeed indicator to show a false reading, with a climb causing an increase in speed indication, and a dive a decrease in speed indication. As an example, a blocked pitot line, caused by a wasp building a nest in an unprotected probe, causes no airspeed indication as the aircraft accelerates during its take-off run. If icing was the cause of the blockage, on the ground or in the air, the application of pitot heat could resolve the problem. Effects of Pitot Static System Leaks A pitot or a static line leak will cause errors in the indication of altitude and/or airspeed, and these errors compound if the aircraft is pressurised. In an unpressurised aircraft, a static leak may cause a standing error on the altimeter and airspeed indicator and has a minimal effect on the vertical speed indicator. A pitot line leak will reduce the effective ram air pressure sensed at the probe, resulting in an under-reading airspeed indication. A pressurised aircraft will attempt to bleed cabin air into the static system, and this would result in a lower altitude indication than the actual altitude. The airspeed indicator will also under read. The vertical speed indicator may operate normally, be slow to respond or even not respond at all, depending on the nature of the leak. Static line leak A pitot line leak would expose the airspeed indicator to cabin pressure, potentially adding to the ram air pressure and causing an overreading. 2023-01-18 B1-11f Turbine Aeroplane Aerodynamics, Structures and Systems Page 59 of 356 CASA Part Part 66 - Training Materials Only Pitot Static System Precautions System Precautions In most cases, it is necessary to provide a heating element for the pitot probe to prevent icing. Static ports mounted on the fuselage are generally not heated. The following information is intended to provide a general awareness of the standard maintenance practices that apply to all pitot static systems. Specific information in the aircraft’s maintenance manual always takes precedence. The pitot static probe is a delicate instrument, and care not to bend or twist the probe is necessary. Probe alignment is critical, as is the condition of all the openings. Pitot static probe While the aircraft is on the ground, probe covers, complete with a high visibility trailing warning flag, should be fitted at all times. This action provides protection for the probe, reduces the chance of injury to personnel, minimises the chances of water getting into the plumbing and removes the opportunity for wasps to enter the pitot port and cause a blockage by building a nest. 2023-01-18 B1-11f Turbine Aeroplane Aerodynamics, Structures and Systems Page 60 of 356 CASA Part Part 66 - Training Materials Only Probe protection Pitot Static System Precautions Example A typical example occurred in 2013 when an Airbus A330 suffered a rejected take-off in Brisbane, Australia, due to an airspeed indication failure which was only detected during the take-off roll. During the subsequent inspection, it was found that the captain’s pitot probe was almost totally obstructed by an insect nest, consistent with mud-dauber wasp nest residue. Airbus A330 wasp nest partial blockage of captain’s pitot probe 2023-01-18 B1-11f Turbine Aeroplane Aerodynamics, Structures and Systems Page 61 of 356 CASA Part Part 66 - Training Materials Only The residue was built up while the aircraft was on the ground over a two-hour period while the aircraft was parked at the loading gate. The pitot probe covers were not installed by maintenance staff during this time. While the ATSB Report AO-2013-212 in relation to this occurrence indicates that a mud dauber wasp nest can completely block a pitot tube inside two hours, CASA has received anecdotal evidence which indicates that the wasps can build a significant nest capable of completely blocking a pitot tube, vent or drain in around 20 minutes. Reference AIRWORTHINESS BULLETIN AWB 02-052 Issue 4 – 3 May 2018 Mud dauber wasp emerging from an uncovered pitot tube During leak tests of the pitot static system, it may be necessary to seal some of the holes on the probe or the fuselage mounted ports with plastic adhesive tap