CASAMod12HeliAeroFlightControlsB112aSR20201001-SR PDF
Document Details
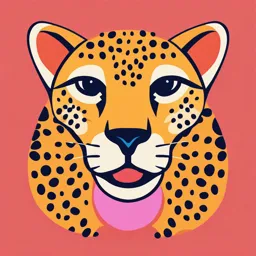
Uploaded by TolerableOrbit
null
2020
Aviation Australia
Tags
Summary
This document is a student resource about helicopter aerodynamics and flight controls, covering various topics like rotary wing theory, flight control systems, and rotor components. It includes definitions, study resources, and explanations of different concepts.
Full Transcript
Student Resource Subject B1-12a: Helicopter Aerodynamics and Flight Controls Copyright © 2020 Aviation Australia All rights reserved. No part of this document may be reproduced, transferred, sold, or otherwise disposed of, without th...
Student Resource Subject B1-12a: Helicopter Aerodynamics and Flight Controls Copyright © 2020 Aviation Australia All rights reserved. No part of this document may be reproduced, transferred, sold, or otherwise disposed of, without the written permission of Aviation Australia CONTROLLED DOCUMENT 2020-10-01 B1-12a Helicopter Aerodynamics and Flight Controls Page 2 of 8 CONTENTS CONTENTS............................................................................................................................................ 3 DEFINITIONS......................................................................................................................................... 4 STUDY RESOURCES............................................................................................................................... 5 Topic 12.1 Rotary Wing Theory of Flight......................................................................................... 6 Topic 12.2.1 Flight Control Systems and their Operation............................................................... 7 Topic 12.2.2 Main Rotor Heads (MRH)............................................................................................ 7 Topic 12.2.3 Main and Tail Rotor Blades......................................................................................... 8 2020-10-01 B1-12a Helicopter Aerodynamics and Flight Controls Page 3 of 8 DEFINITIONS Define To describe the nature or basic qualities of. To state the precise meaning of (a word or sense of a word). State Specify in words or writing. To set forth in words; declare. Identify To establish the identity of. List Itemise. Describe Represent in words enabling hearer or reader to form an idea of an object or process. To tell the facts, details, or particulars of something verbally or in writing. Explain Make known in detail. Offer reason for cause and effect. 2020-10-01 B1-12a Helicopter Aerodynamics and Flight Controls Page 4 of 8 STUDY RESOURCES B1-12a Student Handout. 2020-10-01 B1-12a Helicopter Aerodynamics and Flight Controls Page 5 of 8 INTRODUCTION This subject explains how basic rotary wing aerodynamics is applied to a variety of different helicopter designs and types and the effects of various rotor configurations in flight. The purpose, function and operation of basic helicopter flight controls is explained in detail. On completion of this topic you should be able to: Topic 12.1 Rotary Wing Theory of Flight Define the following terms Air density Angle of attack Axis of rotation or shaft axis Blade loading Centrifugal force Collective pitch Coning angle Cyclic pitch Disc loading Feathering Lift thrust vector resultant Node Pitch angle Relative airflow Thrust or virtual axis Tip path plane. Describe vortex ring state, settling with power, over-pitching and their relationships. Explain torque reaction and describe its effect on directional control of a helicopter. Explain gyroscopic precession and describe how its effect is used in the control of the main rotor disc to provide forward, sideways and rearward flight. Explain the dissymmetry of lift and describe the design feature that is used to control it. Define Corriolis effect. Describe the design features (lead/lag hinges and underslung rotor) that are used to relieve stresses created by Corriolis effect and describe how these features achieve that result. Describe ground effect and translational lift and their relationship. Define translating tendency and describe the two methods (mast offset and cyclic rigging) of correcting this tendency. Describe the reason why rotor blades have built-in twist. Describe what blade tip stall is and why it results in a nose pitch-up of the helicopter. Describe the aerodynamic features of helicopter autorotation. Describe the areas of the disc that provide rotor drive and overall lift during the autorotation. 2020-10-01 B1-12a Helicopter Aerodynamics and Flight Controls Page 6 of 8 Describe static and dynamic stability and state why most helicopters are considered statically stable and dynamically unstable Topic 12.2.1 Flight Control Systems and their Operation Explain the layout, operation and maintenance requirements of a typical cyclic control system and its components (cyclic stick to pitch-change rod inclusive). Explain methods used for helicopter pitch attitude compensation with cyclic application. Explain the layout, operation and maintenance requirements of a typical collective control system and its components (collective lever to pitch-change rod inclusive). Explain methods used for rotor RPM compensation with application of collective control. Explain the principles of operation of a swashplate and state its effect on tip path plane. Explain operational methods (tail rotor, bleed air, fan aerodynamic and contra- rotating) of achieving directional/anti-torque control.. Explain the operation of flight controls by the following methods: Manual Hydraulic Pneumatic Electrical and Fly by Wire. Explain the operation and effect of: Trim control Fixed and adjustable stabilisers and Artificial feel Explain balancing and rigging of flight controls. Topic 12.2.2 Main Rotor Heads (MRH) Describe the physical features of the various main rotor head (MRH) designs and be able to state which features accommodate the flapping, feathering, leading and lagging actions of the main rotor blade. Identify the various types MRH dampers and the describe methods used to dampen vibration. Describe the mounting, inspection and maintenance of main rotor heads. Describe the construction, operation and application of elastomeric bearings in main rotor heads. Describe the various methods used to dampen vibration. 2020-10-01 B1-12a Helicopter Aerodynamics and Flight Controls Page 7 of 8 Topic 12.2.3 Main and Tail Rotor Blades Describe the construction, attachment and material used in typical wooden, metal and composite main and tail rotor blades. Describe typical inspections/maintenance of main rotor blades. Describe the built-in crack detection methods used on main rotor blades. (BIM and BIS). 2020-10-01 B1-12a Helicopter Aerodynamics and Flight Controls Page 8 of 8 TOPIC 12.1.1: ROTARY WING THEORY OF FLIGHT Table of Contents List of Figures................................................................................................................................... 4 TOPIC 12.1.1: ROTARY WING THEORY OF FLIGHT......................................................................... 7 Newton’s First Law........................................................................................................................... 7 Newton’s Second Law...................................................................................................................... 8 Newton’s Third Law.......................................................................................................................... 9 Terminology................................................................................................................................... 10 Air Density.................................................................................................................................. 10 Angle of Attack........................................................................................................................... 10 Inflow Angle................................................................................................................................ 11 Axis of Rotation.......................................................................................................................... 11 Shaft Axis.................................................................................................................................... 12 Flapping...................................................................................................................................... 12 Feathering.................................................................................................................................. 12 Disc Loading................................................................................................................................ 12 Blade Loading............................................................................................................................. 12 Solidity........................................................................................................................................ 13 Lead-Lagging (Dragging)............................................................................................................. 13 Coning Angle............................................................................................................................... 13 Feathering Axis........................................................................................................................... 13 Chord Line.................................................................................................................................. 14 Blade Angle (also known as the Pitch Angle).............................................................................. 14 Relative Airflow.......................................................................................................................... 14 Induced Flow.............................................................................................................................. 14 Blade Angle and Angle of Attack................................................................................................ 14 Tip Path...................................................................................................................................... 14 Tip Path Plane............................................................................................................................. 14 Disc Area..................................................................................................................................... 14 Collective Pitch........................................................................................................................... 15 Cyclic Pitch.................................................................................................................................. 15 Ground Effect............................................................................................................................. 15 Gyroscopic Precession................................................................................................................ 15 Cyclic Pitch Angle (Blade Angle)................................................................................................. 15 2020-09-24 B1-12.1.1 Rotary Wing Theory of Flight Page 1 of 67 Training Material Only Coriolis Effect.............................................................................................................................. 15 Lift.............................................................................................................................................. 16 Drag............................................................................................................................................ 16 Blade Stall................................................................................................................................... 17 Total Rotor Thrust Vector Resultant........................................................................................... 18 Node........................................................................................................................................... 19 Anti-Node................................................................................................................................... 19 Centrifugal Force........................................................................................................................ 20 Centripetal Force........................................................................................................................ 20 Turning....................................................................................................................................... 20 Basic Helicopter Theory............................................................................................................ 23 Vortex Ring State............................................................................................................................ 24 Over-Pitching.................................................................................................................................. 26 Anti-Torque Systems Tail Rotors.................................................................................................... 27 NOTAR (NO TAil Rotor).................................................................................................................. 28 Fenestron....................................................................................................................................... 28 Twin Rotor Design.......................................................................................................................... 29 Tandem, Contra-rotating and Intermeshing.............................................................................. 29 Helicopter Control.................................................................................................................... 30 Cyclic Control (Column).................................................................................................................. 30 Collective Control (Lever)............................................................................................................... 30 Controls Total Rotor Thrust........................................................................................................ 30 Yaw Control (Pedals)....................................................................................................................... 31 Swashplate..................................................................................................................................... 32 Dissymmetry of Lift......................................................................................................................... 35 Gyroscopic Procession.............................................................................................................. 37 Gyroscopic Procession Theory........................................................................................................ 37 Phase Lag........................................................................................................................................ 38 Advance Angle................................................................................................................................ 38 Coriolis Effect................................................................................................................................. 40 Coning............................................................................................................................................ 41 Underslung Rotor........................................................................................................................... 42 Ground Effect................................................................................................................................. 43 Translational Lift............................................................................................................................. 45 2020-09-24 B1-12.1.1 Rotary Wing Theory of Flight Page 2 of 67 Training Material Only Translating Tendency..................................................................................................................... 45 Blade Twist..................................................................................................................................... 47 Blade Tip Stall................................................................................................................................. 48 Autorotation............................................................................................................................ 50 Vertical Autorotation................................................................................................................. 50 Aerodynamics of Autorotation...................................................................................................... 50 Pilot Control of the Driving Region................................................................................................ 52 Gliding Autorotation...................................................................................................................... 53 The Three Phases of Autorotation................................................................................................. 54 The Entry.................................................................................................................................... 54 The Steady State Descent.......................................................................................................... 55 Deceleration and Touchdown.................................................................................................... 56 The Tail Rotor in Autorotation....................................................................................................... 57 Helicopter Axes of Stability...................................................................................................... 58 Positive Static and Dynamic Stability............................................................................................. 59 Negative Static and Dynamic Stability........................................................................................... 60 Neutral Static and Dynamic Stability............................................................................................. 60 Hovering Stability (Pitch/Roll)........................................................................................................ 61 Longitudinal Stability...................................................................................................................... 61 Lateral Stability............................................................................................................................... 62 Directional Stability (Hovering)...................................................................................................... 62 Directional Stability (Forward Flight)............................................................................................. 63 Methods Used to Reduce the Dynamic Instability........................................................................ 63 Stabiliser Bars................................................................................................................................. 64 Use of Fixed and Adjustable Stabilisers......................................................................................... 65 Horizontal and Vertical Stabiliser Angle......................................................................................... 65 Horizontal Stabiliser....................................................................................................................... 66 Vertical Stabiliser........................................................................................................................... 67 2020-09-24 B1-12.1.1 Rotary Wing Theory of Flight Page 3 of 67 Training Material Only List of Figures Figure 1: Newton’s First Law............................................................................................................ 7 Figure 2: Newton’s Second Law....................................................................................................... 8 Figure 3: Newton’s Third Law........................................................................................................... 9 Figure 4: Air density....................................................................................................................... 10 Figure 5: Inflow angle..................................................................................................................... 11 Figure 6: Axis of rotation................................................................................................................ 11 Figure 7: Shaft axis......................................................................................................................... 12 Figure 8: Blade loading................................................................................................................... 12 Figure 9: Coning angle.................................................................................................................... 13 Figure 10: Feathering axis................................................................................................................ 13 Figure 11: Disc area.......................................................................................................................... 15 Figure 12: Lift/drag........................................................................................................................... 16 Figure 13: Blade stall........................................................................................................................ 17 Figure 14: Thrust vector resultant.................................................................................................... 18 Figure 15: Nodes.............................................................................................................................. 19 Figure 16: Centripetal force............................................................................................................. 20 Figure 17: Turning............................................................................................................................ 21 Figure 18: Acceleration.................................................................................................................... 22 Figure 19: Angle of bank................................................................................................................... 22 Figure 20: Lift/thrust resultant......................................................................................................... 23 Figure 21: Vortex ring state - graphical............................................................................................ 24 Figure 22: Vortex ring state - pictorial............................................................................................. 24 Figure 23: Induced flow.................................................................................................................... 25 Figure 24: Over-pitching................................................................................................................... 26 Figure 25: Tail rotor.......................................................................................................................... 27 Figure 26: NOTAR............................................................................................................................. 28 Figure 27: Fenestron........................................................................................................................ 28 Figure 28: Contra-rotor.................................................................................................................... 29 Figure 29: Tandem rotor.................................................................................................................. 29 Figure 30: Cyclic control................................................................................................................... 30 Figure 31: Collective operation........................................................................................................ 31 Figure 32: Yaw Control..................................................................................................................... 31 Figure 33: Swashplate...................................................................................................................... 32 2020-09-24 B1-12.1.1 Rotary Wing Theory of Flight Page 4 of 67 Training Material Only Figure 34: Helicopter blade rotation positions................................................................................. 34 Figure 35: Rotor head....................................................................................................................... 34 Figure 36: Dissymmetry of lift.......................................................................................................... 35 Figure 37: Blade tip speed................................................................................................................ 36 Figure 38: Blade deflection.............................................................................................................. 37 Figure 39: Phase lag......................................................................................................................... 38 Figure 40: Swashplate...................................................................................................................... 39 Figure 41: Coriolis effect.................................................................................................................. 40 Figure 42: Conservation of angular momentum.............................................................................. 40 Figure 43: Coning.............................................................................................................................. 41 Figure 44: Coning angle.................................................................................................................... 41 Figure 45: Underslung rotor............................................................................................................. 42 Figure 46: Ground effect - vortices.................................................................................................. 43 Figure 47: Ground effect - induced flow.......................................................................................... 44 Figure 48: Transitional lift................................................................................................................ 45 Figure 49: Translating tendency....................................................................................................... 46 Figure 50: Built-in bias to correct translating tendency.................................................................. 46 Figure 51: Without blade twist........................................................................................................ 47 Figure 52: With blade twist.............................................................................................................. 47 Figure 53: Lift distribution along blade span................................................................................... 48 Figure 54: Stall area.......................................................................................................................... 49 Figure 55: Lift and drag vectors during autorotation....................................................................... 51 Figure 56: Autorotative forces......................................................................................................... 52 Figure 57: Autorotative forces in glide............................................................................................. 53 Figure 58: Lift and drag vectors during powered flight................................................................... 54 Figure 59: The entry......................................................................................................................... 55 Figure 60: The steady state descent................................................................................................ 56 Figure 61: The flare.......................................................................................................................... 57 Figure 62: Lateral stability (main rotor)........................................................................................... 58 Figure 63: Longitudinal stability(main rotor)................................................................................... 58 Figure 64: Directional stability(tail rotor)......................................................................................... 59 Figure 65: Positive static and dynamic stability............................................................................... 59 Figure 66: Negative static and dynamic stability............................................................................. 60 Figure 67: Neutral static and dynamic stability............................................................................... 60 2020-09-24 B1-12.1.1 Rotary Wing Theory of Flight Page 5 of 67 Training Material Only Figure 68: Pitch and roll................................................................................................................... 61 Figure 69: Longitudinal stability....................................................................................................... 61 Figure 70: Lateral stability................................................................................................................ 62 Figure 71: Directional stability......................................................................................................... 62 Figure 72: Vertical stabiliser............................................................................................................. 63 Figure 73: Helicopter........................................................................................................................ 63 Figure 74: Stabiliser bar.................................................................................................................... 64 Figure 75: Stabiliser bar with weights.............................................................................................. 64 Figure 76: Stabiliser bar with mini-blades........................................................................................ 65 Figure 77: Horizontal and vertical stabiliser.................................................................................... 65 Figure 78: Horizontal stabiliser #1................................................................................................... 66 Figure 79: Horizontal stabiliser #2................................................................................................... 66 Figure 80: Vertical stabiliser............................................................................................................. 67 2020-09-24 B1-12.1.1 Rotary Wing Theory of Flight Page 6 of 67 Training Material Only TOPIC 12.1.1: ROTARY WING THEORY OF FLIGHT Newton’s First Law All bodies at rest or in uniform motion along a straight line will continue in that state unless acted upon by an outside force. Newton’s first law defines the principle of inertia, which means that bodies tend to keep doing what they are doing. If they are “doing” anything at all while in motion, the path the body travels are a straight line. If change is required, then a force must be applied to achieve that change. For example, getting a locomotive moving down a track requires a force which would be greater than the force required to get a small car rolling along a level road. The fundamental physical difference between a locomotive and a compact car is their mass, Mass means the amount (or quantity) of matter in a body; it is directly proportional to inertia. Thus, to change the state of rest of any body, a force is required that must be proportional to the mass of that body. The larger the mass and thus the greater its inertia, the greater the force required. A body’s inertia does not change unless its mass changes. A helicopter at sea level or at altitude, flown fast or slow, has the same inertia, provided its mass does not change. The term inertia is often confused with momentum; Momentum considers not only the mass of the body concerned, but also the velocity at which it travels. Bodies at rest cannot have momentum, although they do have inertia. For a given mass within a body, the faster it travels, the greater its momentum. When a helicopter travels faster, its momentum increases, and a greater force is required to bring it to a halt. Alternatively, if its velocity stays the same, but there are more people on board, then momentum increases, this time because of the increase in mass and again, a greater force is required to bring the aircraft to a stop. The greater the mass of a body, the greater its inertia and the greater the force required to change its slate at rest or uniform motion along a straight line. This principle applies no matter where the body is or whether it is moving fast, slow or not at all. For a given mass, however, if it has velocity it will have momentum as well as inertia. The greater the velocity, the greater the momentum and the greater the force required to change its state of uniform motion along a straight line. Figure 1: Newton’s First Law 2020-09-24 B1-12.1.1 Rotary Wing Theory of Flight Page 7 of 67 Training Material Only Newton’s Second Law Force is proportional to Mass times Acceleration - F = M x A. To accelerate a body at a given rate, the force used must be proportional to the mass of that body. Alternatively, if a given mass must be accelerated at a higher rate, then the force required must be greater. The accelerated air (the induced flow) through a rotor system, which produces the required force for sustained flight, is a good example of this law in action. If the amount of air is increased, then its mass is greater, and as a result the acceleration required can be reduced to provide the same upward force. Alternatively, if the aircraft is heavier and the force required to keep it airborne is greater, then for the same mass of air processed through the rotor disc, its acceleration needs to be greater. Figure 2: Newton’s Second Law 2020-09-24 B1-12.1.1 Rotary Wing Theory of Flight Page 8 of 67 Training Material Only Newton’s Third Law For every action there is an, equal and opposite reaction. Newton’s third law is often misused by assuming that the word action means force. One force is not always equally opposed by another. Only when no acceleration takes place, either in terms of speed or direction, could one say that all forces are equal and opposite and only then could one say that to each force there is an equal and opposite force. When a helicopter hovers at precisely one height, all actions (and in this case “forces”) have equal and opposite reactions, but this applies only so long as there is no accelerated movement up/down, left/right or fore/aft. Figure 3: Newton’s Third Law 2020-09-24 B1-12.1.1 Rotary Wing Theory of Flight Page 9 of 67 Training Material Only Terminology Air Density The number of air molecules per unit volume. Air density is primarily influence by atmospheric pressure, air temperature and moisture content. Rotor thrust is dependent on lift production. Density of air has a strong influence on lift produced. Reduced air density causes the helicopter pilot to struggle with: A requirement for larger angles of attack A worsening Total Rotor Thrust (TRT)/Rotor Drag Ratio A requirement for more power The helicopter’s maximum operating altitude and maneuverability are therefore directly related to these limiting factors. Figure 4: Air density Angle of Attack A symmetrical aerofoil (and many helicopters blades are of the symmetrical shape) that is asked to produce practical lift must be inclined to some extent to the relative airflow so that the air’s deflection over the top of the aerofoil is greater than that underneath. The inclination of the aerofoil’s chordline to the airflow is known as the aerofoil’s angle of attack. Angle of attack and induced flow are inversely proportional for a given blade section and rotor RPM angle of attack: The angular difference between the chord of the blade and the relative airflow (also known as relative wind). 2020-09-24 B1-12.1.1 Rotary Wing Theory of Flight Page 10 of 67 Training Material Only Inflow Angle The inflow angle is the angle between the plane of rotation and the resultant airflow (relative airflow). Figure 5: Inflow angle Axis of Rotation The line through the rotor head at right angles to the Plane of Rotation (POR). The blade actually rotates around this axis. This axis tilts with the disc. Figure 6: Axis of rotation 2020-09-24 B1-12.1.1 Rotary Wing Theory of Flight Page 11 of 67 Training Material Only Shaft Axis The Shaft Axis is the centreline through the rotor shaft (mast). Only when the plane of rotation is exactly perpendicular to the shaft axis will the axis of rotation coincide with the shaft axis. Figure 7: Shaft axis Flapping Movement of a blade in the vertical sense relative to the plane of rotation. Feathering The movement of the blade about its axis (which results in pitch angle changes). Disc Loading The gross weight of the helicopter divided by the disc area, expressed as lb./sq. inch or kg/m2. Since disk area is not constant in flight, it follows that disk loading cannot be constant Blade Loading The gross weight of the helicopter divided by the combined area of the helicopter blades, expressed as above. Since blade area does not alter, blade loading must be a constant in flight (ignoring weight and g changes). Figure 8: Blade loading 2020-09-24 B1-12.1.1 Rotary Wing Theory of Flight Page 12 of 67 Training Material Only Solidity The ratio of total blade area to disk area. Solidity is a function of ability to absorb power from the engine and potential to provide rotor thrust. Lead-Lagging (Dragging) Movement of the blade forward or aft in the plane of rotation. Coning Angle The angular difference between the spanwise axis and the plane of rotation. It may also be defined as the angular difference between the feathering axis and the tip plane path. Figure 9: Coning angle Feathering Axis The straight-line axis between the root of the blade and its tip about which the blade can alter its blade angle. Figure 10: Feathering axis 2020-09-24 B1-12.1.1 Rotary Wing Theory of Flight Page 13 of 67 Training Material Only Chord Line The straight line between the chord of the blade and the plane of rotation. This angle may be altered by the pilot through movement of the collective lever or through the cyclic control. Blade Angle (also known as the Pitch Angle) The angular difference between the chord of the blade and the plane of rotation. This angle may be altered by the pilot through movement of the collective lever or through the cyclic control. Relative Airflow The resultant airflow to each blade due to its speed/direction and the flow induced downwards. Induced Flow The mass of air that is forced down by the rotor action. Blade Angle and Angle of Attack The angle between the chord of the blade and the plane of rotation is known as the blade angle or pitch angle and is controlled through the collective pitch control. If the pilot pulls the collective lever up blade angle increases, and if the pilot pushes the collective lever down, the blade angle decreases. The airflow is in the plane of rotation and as long as no other airflows interfere, the blade angle is also the angle of attack. The blade angle does not affect the direction from which rotational speed (VR) occurs. Whether the plane of rotation is parallel to the ground or tilted, VR will always be parallel to the plane of rotation. Tip Path The circular path described by the tips of the rotor blades. Tip path plane is parallel to the plane of rotation which acts through the rotor head. A pilot may alter this plane through movement of the cyclic control. Tip Path Plane The path with in which the tips of the rotor blades travel. It is parallel to the plane of rotation which acts through the rotor head. A pilot may alter this plane through movement of the cyclic control. Disc Area The area contained within the tip path plane. In flight, this area is not a constant since it is affected by the coning angle of the blades. 2020-09-24 B1-12.1.1 Rotary Wing Theory of Flight Page 14 of 67 Training Material Only Figure 11: Disc area Collective Pitch Pitch angle of all main rotor blades is varied equally and simultaneously. Cyclic Pitch Pitch angle of the main rotor blades is varied individually during a cycle of revolution of the rotor disc. Ground Effect A beneficial gain in lifting power when operating near the ground and gaining an air cushion underneath the helicopter and disc. Gyroscopic Precession A characteristic of all rotating bodies. When a force is applied to the outside of a rotating body parallel to its plane of rotation, the effect of that force is felt 90° later in the direction of rotation. Cyclic Pitch Angle (Blade Angle) Varied by pilot movement of the cyclic control. The cyclic control controls the tilt of the rotor disc and therefore the direction of flight. Coriolis Effect Rotor systems that utilize the individual flapping hinge are subjected to the Coriolis effect to a greater degree than the seesaw system. Coriolis effect is the change in blade velocity to compensate for the change in distance between the centre of mass of the blade to the centre of the axis of rotation as the blade flaps. In other words, as each individual blade flaps upward on the advancing side, the centre of gravity moves closer to the axis of rotation (the mast). This has a tendency to accelerate the blade in much the same manner as a figure skater accelerates a spin by moving his arms inward to the centre of the axis of rotation. 2020-09-24 B1-12.1.1 Rotary Wing Theory of Flight Page 15 of 67 Training Material Only Lift The force produced by the aerofoil that is perpendicular to the relative wind and opposes gravity. The lift is developed by the rotor blade according to Bernoulli's Principle, which simply states that as velocity is increased, the pressure is decreased. This principle creates a low pressure at the top of the rotor blade, while the bottom of the blade has an increased pressure. This applies to both symmetrical and asymmetrical aerofoils. Whenever lift is produced, drag is also produced. Lift is that component of the total aerodynamic force which is perpendicular to the relative airflow. Drag The force which tends to resist the aerofoil’s passage through the air. Drag is always parallel to the relative wind and perpendicular to lift. Drag is the force that tends to slow down the rotor when the angle of attack is increased in order to produce more lift. Drag varies as a square of velocity. Figure 12: Lift/drag 2020-09-24 B1-12.1.1 Rotary Wing Theory of Flight Page 16 of 67 Training Material Only Blade Stall Stall is the condition during which the streamlined flow of air separates from the camber of the blade and reverse flow occurs, resulting in an almost complete loss of lift. This should never occur if the helicopter is being flown within the prescribed flight envelope. As the angle of attack increases, lift increases until the stall angle is reached, provided the velocity remains the same. However, as the angle of attack is increased the lift increases, and so does drag. Because of this increase in drag, the rotor blades have a tendency to slow down. If this should occur the stall angle will be reached prematurely. Blade stall is corrected by reducing collective pitch and increasing engine/rotor RPM simultaneously. If blade stall occurs close to the ground, it will be almost impossible to correct with catastrophic results. Pilots should always ensure that they do not exceed the helicopter’s limitations as stated in the Aircraft Flight Manual. Figure 13: Blade stall 2020-09-24 B1-12.1.1 Rotary Wing Theory of Flight Page 17 of 67 Training Material Only Total Rotor Thrust Vector Resultant The rotor thrust is dependent on lift production. The resultant vector is known as total reaction. Total Reaction (TR) shown in the figure is required to provide the opposing force to weight, but the diagram clearly shows that the TR does not act in line with weight; it “leans back” from the vertical. Thus, a component of the total reaction must be vectored that does act in line with and opposite to weight. That component vector is called Rotor Thrust (RT); it is the force produced by each blade section to overcome part of the helicopter weight. When the rotor thrusts of all the blade sections are combined, Total Rotor Thrust (TRT) acting through the top of the mast provides the force (or component force) that overcomes the weight of the helicopter. Figure 14: Thrust vector resultant 2020-09-24 B1-12.1.1 Rotary Wing Theory of Flight Page 18 of 67 Training Material Only Node A node is a point on a standing wave where the wave has minimum amplitude, or points of no displacement. From Module 2 Physics, you will recall that standing waves result when two sinusoidal waves of the same frequency are moving in opposite directions in the same space and ‘interfere’ with each other. In a standing wave the nodes are a series of locations at equally spaced intervals where the wave amplitude is zero. In a helicopter, a resonance might exist but not be much of a problem. For example, a fuselage may be primarily excited by a rotor to bend vertically in a ‘humpback’ mode. Two points, known as ‘nodes’, do not move. If the seats are on or close to a node, then the crew or passengers will experience a smooth flight even though parts of the fuselage behind and ahead of them may be getting a rougher ride Figure 15: Nodes This is important as vibration can affect not only the aircraft but also cause physical discomfort and fatigue to the crew. The amount of vibration that the human body can withstand varies, but at 4 to 7 hertz the lungs and heart are bouncing vertically with the diaphragm acting as a spring. At around 12 hertz the spinal vertebrae begin to vibrate bouncing off the discs between the individual vertebrae. Good seats will tend to lessen the spinal vibration but either situation is best avoided if crew fatigue and discomfort are to be avoided. Generally, the human body tolerates up- down vibrations more poorly than side-to-side and fore-aft vibrations. More will be discussed about this phenomenon in Topic 12.3.2 Vibration Terminology. Anti-Node This is the point in a vibration range where vibration is at its maximum. An anti-node is the opposite of a node. 2020-09-24 B1-12.1.1 Rotary Wing Theory of Flight Page 19 of 67 Training Material Only Centrifugal Force The force that pulls a spinning object away from its centre of rotation. Centripetal Force The force that opposes centrifugal force and acts toward the centre of a circle. Centrifugal force, determined by rotor RPM, acts in line with the plane of rotation and tries to reduce the conning angle (For a constant radius, centrifugal force is equal to centripetal force). Figure 16: Centripetal force Turning An object travelling on a curve must have a force pulling it towards the centre of the curve. That force is centripetal force. The requirement for centripetal force in a turn is met by total rotor thrust, provided that it is tilted in the direction of the turn. Thus, the helicopter must be banked to make a coordinated turn. The tilted total rotor thrust in a banked turn provides two component forces: The vertical component equal and opposite to weight, which ensures that the helicopter's altitude in the turn remains constant Centripetal force Since total rotor thrust must perform an extra task in providing CPF, it follows that its amount must increase in a turn. This is achieved by increases in blade angles (up collective), which adversely affects the total rotor/thrust/rotor drag ratio. As a result, more power must be applied to maintain rotor RPM, especially at higher angles of bank. 2020-09-24 B1-12.1.1 Rotary Wing Theory of Flight Page 20 of 67 Training Material Only One of the main differences between hovering and forward flight is parasite drag: there is essentially no parasite drag when hovering. Parasite drag increases with the square of the speed increase, which means that the force required to overcome parasite drag must become progressively greater as airspeed increases. The force which overcomes parasite drag is provided by total rotor thrust because, when tilted forward and appropriately increased, it will produce: A vertical component equal and opposite to weight Thrust, in the direction of flight, to deal with parasite drag When the disc is made to tilt forward initially with forward cyclic, the fuselage attitude remains the same briefly. Parasite drag has not yet formed or is still insignificant. The slightly increased total rotor thrust (from a touch of up collective) is inclined forward and produces a nose-down moment around the aircraft centre of gravity. Figure 17: Turning During the accelerating phase, prior to achieving equilibrium total rotor thrust and the resultant are not in line or acting through the aircraft centre of gravity. Any increase in forward speed must be accompanied by a change in disc attitude so that increased total rotor thrust (oriented forward) continues to oppose the increased resultant of weight (a constant) and parasite drag (an increasing variable). The fuselage attitude also changes so that ultimately total rotor thrust, and the weight/parasite drag resultant continue to act through the helicopter's centre of gravity (as shown by the line X - Y). 2020-09-24 B1-12.1.1 Rotary Wing Theory of Flight Page 21 of 67 Training Material Only Figure 18: Acceleration Relationship between angle of bank and requirement for total rotor thrust to provide the vertical component and centripetal force. Figure 19: Angle of bank 2020-09-24 B1-12.1.1 Rotary Wing Theory of Flight Page 22 of 67 Training Material Only Basic Helicopter Theory The main rotor disc is responsible for both lift and thrust, and that thrust can be delivered to cause forward, sideways, or backward motion. Development of lift by use of rotating aerofoils Main rotor disc provides lift and thrust Tail rotor opposes torque reaction developed by main rotor Figure 20: Lift/thrust resultant 2020-09-24 B1-12.1.1 Rotary Wing Theory of Flight Page 23 of 67 Training Material Only Vortex Ring State Vortex ring state, or settling with power, is potentially hazardous because it places the helicopter into a descent at an increasing rate. What’s worse, the recovery action from a vortex ring state may involve even more loss of height. Pilots must use considerable caution when operating at low height if conditions likely to lead to vortex ring state are present. It doesn’t take much to bring on a vortex ring state. Imagine a helicopter hovering at altitude. The relative airflow onto each blade section is dependent upon the induced flow and local rotational speed (VR), both of which are greatest near the blade tip. Assuming that the ratio of rotational velocity to induced flow is more or less constant over the entire blade, then the direction of the relative airflow is about the same throughout the blade. Because of the higher blade angle at the root through wash-out, however, the angle of attack at the root is larger than that at the tip. Figure 21: Vortex ring state - graphical Vortex ring state can develop when the helicopter has: A low or zero airspeed (below translational lift speed) Some power in use (collective input) A rate of descent that is too fast for the aircraft type Figure 22: Vortex ring state - pictorial 2020-09-24 B1-12.1.1 Rotary Wing Theory of Flight Page 24 of 67 Training Material Only The combinations mentioned above are encountered under the following flight conditions. All of these flight conditions require that an induced flow be present, some power be in use and interference to the induced flow by airflow from below the aircraft be present: A powered descent at low or zero airspeed at a “high” rate of descent Loss of height during a harsh flare Executing a quick stop downwind A squashing recovery, when power is applied at the end of an autorotation A downwind approach Any factors that involve high collective settings in the hover or slow flight, such as high gross weight, high altitude, high density altitude and maneuvers, encourages vortex ring state to occur sooner. Figure 23: Induced flow Symptoms of vortex ring state are: Aircraft vibration and cyclic stick shake A random yawing, rolling and pitching increasing the rate of descent A reduction in cyclic stick effectiveness The recommended recovery technique for vortex ring state is: Cyclic forward (and/or slightly to one side) to increase airspeed Brief pause, to allow the airspeed to build up Apply power The key to recovery from vortex ring state is recognizing the condition and acting before it develops. It can have the same catastrophic effects as blade stall, if not corrected in time. 2020-09-24 B1-12.1.1 Rotary Wing Theory of Flight Page 25 of 67 Training Material Only Over-Pitching Over-pitching is different from recirculation (vortex ring state) even though the symptoms have similarity. While over-pitching can occur at any altitude and at various stages of flight, it is more likely to occur when approaching a hover or during a hover. If the rotor RPM decreases during those phases (for whatever reason), total rotor thrust reduces and an unwary pilot might attempt to restore the rotor thrust by pulling up collective. This action invariably tilts the total reaction away from the axis of rotation, worsening the total rotor thrust/rotor drag ratio. Thus, up collective will only result in further decay in rotor RPM. Decaying rotor RPM also causes the helicopter blades’ coning angles to increase. The consequence of this is that, firstly, the disc becomes smaller so that total rotor thrust falls off. Secondly, large coning angles cause rotor thrust to point inward so that smaller vertical components become available to overcome the helicopter’s weight. Figure 24: Over-pitching The only recovery action from over-pitching and restoring rotor RPM is to roll on throttle and simultaneously lower the collective lever. This will invariably cause the helicopter to lose some height. Over-pitching may occur when approaching a high-altitude landing site if the power required to hover is not available. As airspeed decreases and the need for power increases, the helicopter’s descent rate builds when the engine cannot supply the power required. Pilots who then instinctively pull up collective to arrest the sink rate are in serious trouble. The high inflow angles and associated rotor drag quickly decay the rotor RPM and the stage is set for over-pitching. The best scenario ends in a hard landing, while the worst scenario ends in a full rotor stall, at which point the helicopter virtually falls out of the sky. 2020-09-24 B1-12.1.1 Rotary Wing Theory of Flight Page 26 of 67 Training Material Only Anti-Torque Systems Tail Rotors A single rotor helicopter will try to rotate under its rotor in the opposite direction, (Newton’s third law), unless fitted with a tail rotor. This rotor’s thrust leads to a tendency to drift in that direction unless opposed by mast offset or cyclic rigging. Tail rotor system: Counters torque reaction Provides directional control Maintains balanced flight conditions Provides directional control during autorotation Figure 25: Tail rotor 2020-09-24 B1-12.1.1 Rotary Wing Theory of Flight Page 27 of 67 Training Material Only NOTAR (NO TAil Rotor) Is the name of a helicopter system which avoids the use of a tail rotor. The system uses a fan inside the tailboom to build a high volume of low-pressure air, which exits through two slots and creates a boundary layer flow of air along the tailboom utilizing the Coanda effect. The boundary layer changes the direction of airflow around the tailboom, creating thrust opposite the motion imparted to the fuselage by the torque effect of the main rotor. Directional yaw control is gained through a vented, rotating drum at the end of the tailboom, called the direct jet thruster. Figure 26: NOTAR Fenestron A normal rotor has a wake that requires some distance to contract to its final size which is only 70% of the rotor diameter. By putting a duct around the rotor, the wake is effectively matured in the duct and undergoes no further contraction downstream, thus the Fenestron can produce the same total thrust for the same power as a tail rotor 30% larger. For this reason, the Fenestron is always integrated into a generously sized cambered vertical stabiliser that can take over the job of torque compensation in forward flight. This has the advantage of decreasing the loads on the blades and the drive system - which increases the life of the system. Figure 27: Fenestron 2020-09-24 B1-12.1.1 Rotary Wing Theory of Flight Page 28 of 67 Training Material Only Twin Rotor Design Twin, or counter-rotating designs are where one disc rotates clockwise and the other disc rotates counterclockwise. Tandem, Contra-rotating and Intermeshing Figure 28: Contra-rotor Figure 29: Tandem rotor On the tandem, contra and intermeshing configured helicopters, directional stability is dependent upon which rotor is producing the most amount of thrust in a particular direction. Note on the tandem mounted twin rotor designs such as the CH-47 Chinook helicopter (illustrated), yaw pedal input from the pilot has a control effect on the aft rotor. 2020-09-24 B1-12.1.1 Rotary Wing Theory of Flight Page 29 of 67 Training Material Only Helicopter Control Cyclic Control (Column) A control called the “cyclic” controls the plane of the rotor disc and provides pitch and roll control, using the principle of gyroscopic precession. Moving the cyclic control left/right, fore/aft or any combination thereof tilts the swashplate, which changes the blade angle of the individual blades. For instance, in a two- bladed rotor, moving the cyclic forward decreases the blade angle on the right blade and increases the blade angle on the left blade. Although moving the cyclic alters the tilt of the total rotor thrust, the amount of total rotor thrust is not affected. Cyclic merely points the total rotor thrust in any required direction, it doesn't increase or decrease it. Figure 30: Cyclic control Collective Control (Lever) Controls Total Rotor Thrust As the lever is raised, all rotor blade angles increase simultaneously or ‘collectively’. Can Incorporate: Anticipator Twist Grip Collective Control Pulling the collective lever up moves the swashplate vertically, so that all blades obtain the same increase in blade angle. Similarly, pushing the collective down decreases the blade angle to all blades. Variations in blade angle change the amount of total rotor thrust produced. Accordingly, changes in collective cause changes in total rotor thrust (but they do not alter total rotor thrust orientation). An increase in all blade angles (up collective) under most conditions increases rotor drag and may decrease rotor RPM. To facilitate maintenance of rotor RPM, a correlating unit (a cam-link arrangement) is fitted between the collective control and the throttle, increasing power automatically and avoiding a loss of rotor RPM whenever collective is pulled up. The correlating unit decreases power when collective is pushed down. 2020-09-24 B1-12.1.1 Rotary Wing Theory of Flight Page 30 of 67 Training Material Only On piston engine helicopters the twist-grip type throttle at the end of the collective is predominantly an engine RPM fine tune control, even though its use has some influence on manifold pressure. In most modern helicopters a governor is fitted to automatically maintain the required engine RPM and therefore rotor RPM. Figure 31: Collective operation Yaw Control (Pedals) A set of foot pedals adjusts the blade angles on all the tail rotor blades, (like a collective), to provide yaw control, similar pedals provide the same function on NOTAR and dual rotor aircraft. Figure 32: Yaw Control 2020-09-24 B1-12.1.1 Rotary Wing Theory of Flight Page 31 of 67 Training Material Only Swashplate The swashplate is primarily a thrust bearing incorporating a stationary lower plate and a rotating upper plate. It transmits the pilot’s cyclic and collective control inputs to the rotor disc. The cyclic tilts the disc in the desired direction of flight. The collective lever changes each blade’s blade angle. Figure 33: Swashplate Having discussed the anti-torque control, we must now consider the collective control and cyclic control. Both controls perform their functions via a swashplate arrangement (also known as the control orbit) that varies in design from one helicopter to another. The following are principles common to most swashplates. The swashplate arrangement consists of two circular or angular plates (or stars) fitted horizontally one above the other. A ball bearing arrangement separates the two plates and allows horizontal (circular) movement between them. The lower plate is fixed in terms of rotation but has the ability to move up and down and tilt in any given direction. It is referred to as the stationary or non- rotating plate (or star). Pilot inputs alter the vertical position of the plate through the collective control and the tilt of the plate through the cyclic control. 2020-09-24 B1-12.1.1 Rotary Wing Theory of Flight Page 32 of 67 Training Material Only Above the stationary plate is the rotating plate which, as the name implies, has freedom to rotate. Since the rotating plate always follows the orientation of the stationary plate, any pilot input to the stationary plate is passed on to the rotating plate above it. The rotating plate is connected to each individual blade via pitch links to pitch horns fitted to the leading edge of each blade. Thus, the rotating plate can alter the blade angle of each blade. A counterclockwise rotor in forward flight, such as that in the figure below, experiences forward cyclic at position A causing the blade over the tail to reduce its pitch angle. This action is simply the result of the swashplate position which feeds the cyclic input through to the blade in question. The angle of attack of that blade lessens and the blade starts to descend. When descending, airflow created by the descent causes the relative airflow onto the blade to come from lower down. As the blade progresses on its circular path, blade angles decrease (due to swashplate orientation) so that the rate at which the blade descends increases whist the angle of attack essentially does not change. When at position B the blade angle reaches its minimum value and starts to increase. This is to ensure that the rate of going down is reduced so that the rate becomes zero when the blade is over the nose at position C. (If the blade angle were allowed to decrease beyond B, the blade would not stop its descent over the nose of the aircraft.) Thus, at position B the rate of downward movement is maximum and lessens as the blade approaches the front. At position C the rate at which the blade moves down becomes zero and the disc has reached its lowest point over the nose of the aircraft. The blade angle increases here (due to the orientation of the swashplate). As a consequence, the blade’s angle of attack briefly increases, the relative airflow comes from higher up (because of the blades ensuing climb) and the angle of attack returns to its original value. The blade angle continues its increase so that the rate of the blade’s upward movement increases. At position D the blade reaches maximum blade angle and the rate of upward movement is also maximum. From here on, the blade angle decreases so that the rate of upward movement decreases. Returning to position A the rate of upward movement becomes zero, the blade reaches its high point over the tail, and the next sequence starts. 2020-09-24 B1-12.1.1 Rotary Wing Theory of Flight Page 33 of 67 Training Material Only Figure 34: Helicopter blade rotation positions Pitch Angle Forward cyclic causes the plane of rotation to tilt forward under the Influence of changes in blade angle while angles of attack remain essentially constant. The rate at which blades move down and up will be greatest when blade angles are smallest and largest, respectively. Having established the sequence for forward flight, the same principles apply for movement in any other direction. Figure 35: Rotor head 2020-09-24 B1-12.1.1 Rotary Wing Theory of Flight Page 34 of 67 Training Material Only Dissymmetry of Lift Dissymmetry of lift results after a cyclic input changes the plane of rotation (forward in this case) and forward movement has begun. (For the moment we will not consider the changes in blade angle that continue taking place as long as the cyclic stick is held in a steady forward position.). The term dissymmetry of lift refers to the uneven production of rotor thrust across the rotor disk. Once the rotor disc tilts forward, the aircraft moves away from the hover and goes through transition (movement from the hover to forward flight and forward flight back to the hover). Because one rotor blade moves forward and the other blade moves back relative to the helicopter as forward airspeed is gained, the advancing blade operates at its rotational velocity plus the airflow created by forward airspeed, while the retreating blade operates at its rotational velocity minus the airflow created by forward airspeed. Figure 36 shows a counterclockwise rotor flying towards the top of the page. It assumes that the velocity caused by rotation of the blade section is 400 knots and the aircraft forward speed is 20 knots. The forward speed creates an airflow that moves onto the entire helicopter at a speed equal to the forward velocity. In Figure 36, this movement is represented by six arrows of 20 knots each. The advancing blade section on the right experiences 400 knots from rotor RPM plus the 20 knots created by forward airspeed, an effective relative airflow of 420 knots. The retreating blade on the left experiences an airflow caused by rotation minus the airflow from forward speed: 400 knots minus the 20 knots caused by forward velocity, producing an effective relative airflow of 380 knots. Lift production is the result of a given angle of attack and velocity. A higher velocity on the advancing blade produces more lift than the reduced velocity on the retreating blade. Consequently, the total rotor thrust produced on the advancing half of the disc is greater than that produced on the retreating half and the helicopter rolls to the retreating side, which is dissymmetry of lift. Thus, dissymmetry of lift is caused by the airflow created by aircraft airspeed or caused by the wind when the helicopter is stationary such as when hovering. If the speed of the helicopter increases, say to 50 knots, or if the wind is stronger, dissymmetry of lift increases. Figure 36: Dissymmetry of lift 2020-09-24 B1-12.1.1 Rotary Wing Theory of Flight Page 35 of 67 Training Material Only Blade flapping, (either hinged or flex), equalises lift across the disc. As the aircraft moves into forward flight, one half of the disc begins to create more lift than the other half due to the increased airflow on the advancing blade side and reduction on the retreating side. This is called dissymmetry of lift and if left unchecked will cause the helicopter to roll over. It was Juan de Cierva (pioneer of the Autogyro) who incorporated the flapping hinge into each blade, eliminating this problem. The flapping hinge allows each blade to move freely about its vertical axis, or to move up and down. This movement is referred to as flapping. Since more lift is created by the advancing blade, the blade has a tendency to flap up. This decreases the angle of attack and reduces the amount of lift on the advancing side of the disc. At the same time, with less lift being created on the retreating blade, the retreating blade takes a more horizontal position (flaps down). This increases the angle of attack and the amount of lift on the retreating side of the disc. Flapping to equality. Figure 37: Blade tip speed 2020-09-24 B1-12.1.1 Rotary Wing Theory of Flight Page 36 of 67 Training Material Only Gyroscopic Procession Gyroscopic Procession Theory Deflection takes place 90° in the direction of rotation from the input force Side input = aft or forward deflection depending on the direction of rotation Angle of Attack continuously changing An Alternate Explanation of Cyclic Action If a horizontally rotating rotor disc reorients itself so that its low point is over the nose of the helicopter, then the greatest input for downward movement must be made 90° beforehand in accordance with the principle of gyroscopic precession. Thus, the blade angle must be at the lowest on the right-hand side of the helicopter, where the rate of downward movement must be greatest, and beyond which the downward velocity decreases. If the disc shall have its high point over the tail, the greatest input for upward movement must be made 90° beforehand, on the left-hand side. That is where the blade angle must be greatest and beyond which the rate of upward movement decreases. Figure 38: Blade deflection 2020-09-24 B1-12.1.1 Rotary Wing Theory of Flight Page 37 of 67 Training Material Only Phase Lag The operation of the cyclic control was stated that if the rotor disc is to be lowest over the nose of the helicopter, the greatest input in flapping down must be made 90° beforehand, that is, minimum pitch angle midway on the advancing side. The principle of gyroscopic precession then causes the cyclic input to act 90° further on in the direction of rotation. Thus, a cyclic input causes the disc attitude to change, the blade reaching its highest and lowest position 90° later than the point where the maximum increase and decrease of cyclic pitch are experienced. This phenomenon is known as phase lag. Figure 39: Phase lag Advance Angle If moving the cyclic control causes the swashplate to tilt in the same direction, and, if in response to changes in pitch angles, the rotor disc tilts 90° out of phase, then the disc will also be 90° out of phase with the cyclic stick movement. For instance, forward cyclic would cause a roll to the right or left depending on main rotor rotation. To overcome this problem, the orientation of the swashplate and attachments of the pitch links are altered in such a way that forward cyclic always causes the main rotor to tilt forward and a similar response applies for flight in any direction. Using forward cyclic for forward flight, the pitch angle of the blade on the pilot's right must be at its minimum on counterclockwise rotating main rotors. The leading edge of that blade (at that position) is lowest down (or the trailing edge highest up). If the swashplate has the same tilt as the main rotor, then the pitch link must be attached to the front of the rotating plate. The lowest position of the swashplate then pulls the leading edge of the advancing blade lowest down. 2020-09-24 B1-12.1.1 Rotary Wing Theory of Flight Page 38 of 67 Training Material Only The angular difference between the centre of the swashplate and the point on the swashplate where the pitch link is attached in advance of the blade to which it relates is known as advance angle. The advance angle is the difference between the attachment point of the pitch link on the swashplate and the blade to which it refers. Thus, the advance angle in this case is 90°. Forward cyclic causes the swashplate to have its low point at the front (and the high point at the rear), as shown in Figure 39 B. The pitch link pulls the leading edge of the blade on the pilot's right (the advancing blade) as low as it can go (minimum pitch angle). The 90° precession (phase lag) rule then causes the main disc to orient down in front (and up at the back). The tilt of the main rotor, the tilt of the swashplate and movement of the cyclic stick are in the same sense, fully compensating for phase lag. If the cyclic stick movement is not in sympathy with the swashplate tilt, then the advance angle has to be adjusted. If the cyclic is 45° out of phase with swashplate orientation, the advance angle has to be 45° to fully compensate for phase lag. Figure 40: Swashplate 2020-09-24 B1-12.1.1 Rotary Wing Theory of Flight Page 39 of 67 Training Material Only Coriolis Effect Conservation of angular momentum (Coriolis Effect) influences the rotational velocity of a blade when it flaps up or down. Any section of a blade travels on a given radius at a given velocity and because of this; each section (and ultimately the entire rotor blade) has a certain angular momentum that tends to be retained. Figure 41: Coriolis effect When the radius on which the centre of gravity of the blade travels is reduced, such as when the blade flaps up or cones upward, the centre of gravity moves in towards the axis of rotation (circumference A changes to B in Figure39), the blade travels in a smaller circle. The blade will then increase its rotational velocity (VR) to conserve angular momentum (in this smaller circle). When a blade flaps down its centre of gravity moves out from the axis of rotation onto a larger radius and its velocity decreases. See Figure 42. Figure 42: Conservation of angular momentum 2020-09-24 B1-12.1.1 Rotary Wing Theory of Flight Page 40 of 67 Training Material Only Coning The ability to flap vertically, either through a hinge or flexibility, results in coning. The blades move upwards due to the vector addition of the lift and centrifugal forces. Rotor RPM must be maintained above a minimum value to avoid excessive coning. Figure 43: Coning Coning angle, governed by the resultant of combined rotor thrust of the blade, and centrifugal force acting through the blade's centre of gravity. Disc area is affected by coning angle. Generally speaking, the larger the rotor disc the greater the total rotor thrust produced. Although the diameter of a given rotor disc ought to be determined by blade length (a fixed value), the influence of the coning angle is to reduce the size of the disc to some degree. The coning angle is dictated by two forces, the combined rotor thrust of the blade and centrifugal force. In the picture you see that the combined rotor thrust of the blade, acting at right angles to the feather axis, tries to increase the coning angle. Figure 44: Coning angle Centrifugal force, determined by rotor RPM, acts in line with the plane of rotation and tries to reduce the coning angle. (For a constant radius, centrifugal force is equal to centripetal force.) 2020-09-24 B1-12.1.1 Rotary Wing Theory of Flight Page 41 of 67 Training Material Only Thus, the resultant of a blade's total rotor thrust, and centrifugal force determines the coning angle. If the rotor thrust increases and centrifugal force remains the same, the coning angle increases, and disc area becomes smaller. Similarly, if the centrifugal force increases (when rotor RPM increases) while rotor thrust remains the same, the coning angle decreases, and disc area becomes larger. Without centrifugal force, coning angles would continue to increase until the blades finally meet directly above the mast. The result would be most unfortunate. It is therefore very important that you never exceed rotor RPM limits, especially the low limit, determined by the manufacturer. (See how we always come back to blade stall?) With VR (rotor RPM) practically a constant, if mass increases then centrifugal force increases and, for a given rotor thrust produced by the blade, the coning angle decreases. Thus, by adding mass to a rotor blade, centrifugal force increases with beneficial results for the coning angle. This technique is used in some "high inertia blades" (when a few ounces of lead are placed inside the blade tips) which travel at considerable speed. The addition of mass at the tips increases the moment of inertia of the blades (which increases angular momentum) so that for the same rotor RPM, the blades operate at a smaller coning angle compared to blades without weights at their tips. Underslung Rotor The underslung rotor system involves attaching the blades to the rotor head lower than the top of the rotor mast. This design reduces vibrations within the rotor and minimises lead-lag tendencies, thereby reducing stress on rotor head components. Figure 45: Underslung rotor 2020-09-24 B1-12.1.1 Rotary Wing Theory of Flight Page 42 of 67 Training Material Only Flapping up or down affects the position of a blade’s centre of gravity from the axis of rotation. For instance, flapping up brings the centre of gravity in closer to the axis of rotation and, through conservation of angular momentum, the particular blade speeds up, moving forward on the lead- lag hinge (Coriolis Effect) if such a hinge is fitted. The underslung rotor system tends to reduce the effect, and, through that, it reduces stress on lead-lag links, or on the fore-aft strength of the blades and attachments. (On some two-blade rotor systems, the flexibility of the mast also assists in reducing the effect of lead-lag forces.) Ground Effect When flying a helicopter flies near the earth’s surface, the rotor downwash is unable to escape as readily as it can when flying higher and creates a ground effect. Figure 46: Ground effect - vortices 2020-09-24 B1-12.1.1 Rotary Wing Theory of Flight Page 43 of 67 Training Material Only When hovering in ground effect the angle of attack is slightly less, the amount of total rotor thrust is the same as the gross weight, the blade angle is smaller, the power required to overcome the reduced rotor drag (or torque) is less and the collective control lever is lower than when hovering out of ground effect. This conclusion applies equally to flight in ground effect other than the hover, but the effect is not as great. Figure 47: Ground effect - induced flow 2020-09-24 B1-12.1.1 Rotary Wing Theory of Flight Page 44 of 67 Training Material Only Translational Lift Although the ground effect is lost as the helicopter gains forward speed, a new force makes its appearance. This is called translational lift, an additional lift which is obtained when entering horizontal flight due to the increased efficiency of the rotor system. The inflow of air into the rotor during forward flight increases. The increase in flow also increases the mass of air at the rotor disc which in turn increases lift. Although the increase takes place any time the helicopter moves horizontally, it is readily noticed at an airspeed of 15 to 20 miles per hour. The additional lift which is available at this is referred to as effective translational lift. It might also be noted that since this is an effect of air speed, it may happen at various points, including hover, if the wind velocity is great enough. Also note that this additional lift will eventually be cancelled by the increased drag of the fuselage. Figure 48: Transitional lift Translating Tendency You can see in Figure 49, that one part of the main rotor torque couple (the forward part) points to the right and the tail rotor thrust points to the right as well. The other part of the main rotor torque couple (the part behind the mast) points to the left. As soon as the helicopter lifts off, there is a tendency for the aircraft to drift to its right under the influence of these uneven forces (two to the right and one to the left). This movement is known as translating tendency, also referred to as tail rotor drift. Translating tendency must be corrected by moving the cyclic to the left so that the disc tilts slightly left and stops the drift to the right. 2020-09-24 B1-12.1.1 Rotary Wing Theory of Flight Page 45 of 67 Training Material Only Figure 49: Translating tendency Some designers incorporate methods that automatically correct for tail rotor drift. The most common ones are: 1. Construction of the main rotor mast so that it tilts slightly to one side and the rotor disc is automatically orientated to that side. 2. The use of a “bias” in the cyclic control mechanism. This involves an arrangement in the cyclic control linkage which holds the cyclic stick slightly to the left, so that the pilot does not have to place it there. Figure 50: Built-in bias to correct translating tendency Translating tendency occurs anytime in flight when power is in use and it must be corrected. In forward flight and especially at speeds approaching cruise speed or higher, the directional stability of the aircraft reduces the requirement for anti-torque so that tail rotor drift becomes less significant. 2020-09-24 B1-12.1.1 Rotary Wing Theory of Flight Page 46 of 67 Training Material Only During a maximum performance takeoff from a confined area, however, where the power use is high and the airspeed is low, if tail rotor drift is not corrected properly, the aircraft tends to drift to its right. The reverse applies when a large amount of tail rotor thrust is used to maintain a constant heading in a hover and the throttle is closed rapidly (to simulate engine failure). A rather abrupt drift to the left then occurs if the disc is not levelled (with right cyclic) as the power is reduced. Blade Twist In viewing the main rotor from the top in a no-wind hover condition, it is quite evident that different parts of the rotor are moving at different speeds. The fastest portion is at the tip of the rotor with the least amount of speed at the root portion of the blade. Figure 51: Without blade twist The blade will often have a twist built into it, in order to improve the lift characteristics of the rotor throughout the blade. The twist will increase from the tip to the root. This twist will increase the angle of attack of the slower portions of the blades thus increasing the total lift of the blade. Figure 52: With blade twist 2020-09-24 B1-12.1.1 Rotary Wing Theory of Flight Page 47 of 67 Training Material Only Figure 53: Lift distribution along blade span Blade Tip Stall The helicopter rotor blades, like any aerofoil, are subject to stall. However, a stall of the rotor is quite different from that of the fixed wing. As a brief review it was learned that in forward speed the advancing blade is moving at a faster speed than the retreating blade. As the speed of the helicopter increases, this speed differential becomes greater. Because of the dissymmetry of lift, the retreating blade will be seeking a higher angle of attack than the advancing blade. This, coupled with the low airspeed of the retreating blade, can lead to blade tip stall. An aerofoil may stall due to any of the following reasons: Insufficient airspeed Too great an angle of attack Heavy wing loading In a helicopter flying at 200 miles per hour, the advancing blade will have a tip speed of approximately 600 miles per hour, while the retreating blade tip speed is reduced to 200 miles per hour. At this point, the root areas are producing no lift. The retreating blade must continue to seek a higher angle of attack in order to maintain lift. Even though the blade has a twist built into it, the inflow of air into the rotor will be such that it will increase the angle of attack at the tips. This is due to the tilting of the rotor and its relationship to the inflow of air to the rotor. It is not possible, however, to predict at what point the rotor will stall each time due to the forward speed because several other factors must also be considered. One of these is wing loading. It is more likely for the blade to stall under heavy loads than under light loads. Heavy loading will only decrease the speed at which the stall will occur. Other factors, such as temperature, altitude, and maneuvers must also be considered. For these reasons a stall may occur at rather low operating speeds. 2020-09-24 B1-12.1.1 Rotary Wing Theory of Flight Page 48 of 67 Training Material Only In Figure54, a rotor system is shown with the stall area marked. It can be seen that as the tip enters the stall condition, only a few inches are involved; but as the blade continues, several feet towards the middle of the blade travel in the stall area, and then it will move out toward the tip. Figure 54: Stall area The indication of a stall condition will first be a vibration as each blade passes through the stall region. The beat could be 2:1, 3:1, or 4:1 depending upon the number of blades in the rotor system. If the stall continues, the helicopter will pitch up. Although the stall will occur on the left side of the helicopter, due to gyroscopic precession, the result will be at the tail of the helicopter, which will pitch the nose up. When a stall is experienced, the corrective action is to reduce forward speed, reduce pitch, and increase rotor speed if possible; but the important factor is always to unload the rotor system. 2020-09-24 B1-12.1.1 Rotary Wing Theory of Flight Page 49 of 67 Training Material Only Autorotation Autorotation is the process of producing lift with freely rotating aerofoils by means of aerodynamic forces which result from the upward flow of air. Autorotation can be subdivided into two groups: Vertical Autorotation Gliding Autorotation Vertical Autorotation During normal powered flight, the pitch angle of the blades is set relatively high, and considerable engine power is required rotate them through the air. When engine power is lost, the helicopter will start to descend, and the up-flow of air through the rotor, will overcome the inertia of the blades in a very short period of time, and begin to push the blades backwards. No lift will be produced with the rotor turning in this direction. If, however, the pilot realizes the loss of power quickly enough and is able to reduce the collective to its minimum before the up-flow of air starts to rotate the blades backwards, then the rotor will begin to autorotate in the forwards direction and produce lift. Aerodynamics of Autorotation To understand how lift can be produced without the aid of power, it is necessary to understand how the angle of attack of the airflow on the blade section varies. Three factors combine to determine the angle of attack on a helicopter rotor blade. Rate of Descent - the up-flow of air through the rotor will increase the angle of attack, in the same way as it does on the blade that flaps-down during "flapping to equality". Forward speed of the aerofoil - this is dependent on the rotational speed of the rotor, forward speed of the helicopter (neglect this for the time being), and most important of all, the distance radially from the centre of rotation. Pitch angle of the aerofoil - this is dependent on the blade pitch setting on its feather axis, and the twist in the blade (also a function of the distance from the centre of rotation) In undertaking the following velocity vector exercise, it is important to remember the following facts: Drag always acts in the same direction as the "relative wind" Lift always acts perpendicular to the "relative wind" and drag Total Aerodynamic Force (TAF) is the vectorial sum of lift and drag 2020-09-24 B1-12.1.1 Rotary Wing Theory of Flight Page 50 of 67 Training Material Only Figure 55: Lift and drag vectors during autorotation It can be seen then, that in vertical autorotation, the rotor disk can be divided into three regions. The driven region - is nearest to the blade tips and normally consists of about 30 % of the radius. The TAF in this region is inclined slightly behind the vertical and hence tries to slow down the rotor. It does however provide considerable lift. The driving region - sometimes called the "Positive Autorotative Region", normally lies between about 25 to 70 % of the blade radius. The TAF in this region is inclined slightly forward of the vertical, and, whilst providing lift, also drives the blade forwards, and overpowers the negative effect of the driven region. The stall region - includes the inboard 25 % of the rotor blade. It operates above the stall angle of attack, and causes drag which tends to slow the rotor. 2020-09-24 B1-12.1.1 Rotary Wing Theory of Flight Page 51 of 67 Training Material Only Figure 56: Autorotative forces Pilot Control of the Driving Region Consider the following factors: 1. Which way would the autorotative regions shift if the pilot increased the collective pitch? 2. Which way would the autorotative regions shift if the rotor speed were reduced? If the pilot were to increase the collective pitch slightly, all regions would shift towards the tip of the rotor blade. However the stalled region would not now be fully stalled, and it would be true to say that a "driving region" nearer the tip has a larger area than nearer the root, but consider what might happen if now, an unforeseen factor increased the pitch even further (such as cyclic pitch control for steering towards a suitable landing spot, or a gust of wind). In this case the positive "driving region" may shift off the end of the blade altogether. It is essential that the rotor is not allowed to decelerate to stationary, otherwise it may start to rotate backwards, and autorotation would be extremely difficult, if not impossible to restore. By designing the centre part of the blade as the "driving section" then, a safety factor is provided, and allows for cyclic steering, gusts of wind etc, etc. 2020-09-24 B1-12.1.1 Rotary Wing Theory of Flight Page 52 of 67 Train