Brain Structure-Function PDF
Document Details
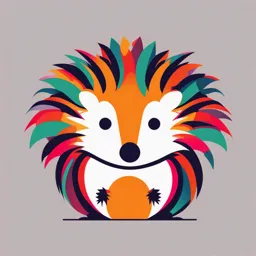
Uploaded by PatientMaple556
Maastricht University
Tags
Summary
This document discusses the relationship between brain structure and function. It covers different brain regions like the cerebellum, hemispheres, brainstem, and four lobes (frontal, parietal, temporal, and occipital), along with their functions. It also examines examples of brain damage, such as the case of Phineas Gage and lobotomies, highlighting their effects on cognitive abilities and personality. Finally, the document explains cognitive control and its key characteristics in detail.
Full Transcript
Brain Structure-Function relationship LG1) How do dissociation logic lesion studies work? The study of the link between cognitive process and specific brain areas by studying individuals with brain lesions Using lesion studies and dissociation logic, scientists can map brain functions an...
Brain Structure-Function relationship LG1) How do dissociation logic lesion studies work? The study of the link between cognitive process and specific brain areas by studying individuals with brain lesions Using lesion studies and dissociation logic, scientists can map brain functions and better understand the organization of cognitive processes Insights help in development of targeted treatments and rehabilitation strategies for brain injuries Single Dissociation: Shows one function is a:ected, while a related function is not Double Dissociation: Confirms two functions are independent by showing each can be impaired alone è Stronger evidence; confirms clear independence, by showing that each function can be e?ected separately, proving they rely on di?erent brain systems > Single Dissociation alone might be unclear whether the two functions are truly separate or if one is just “harder” to disrupt LG2) What are the di:erent brain regions and their functions? Cerebellum (“little brain”): Contains more than half of the brains neurons è Around 69 billion out of the 89 billion we have Essential for smooth and precise motor control Helps with coordinating and regulating various functions, especially those related to balance and movement è If damaged your movements will become uncoordinated and halting Hemisphere: Separates the brain in two halves Although functions in each brain are almost the same the hemispheric lateralization suggests some functions are more dominant in one hemisphere than the other Brainstem: Consist of three parts: 1. Midbrain o Parts of the midbrain are involved in pain modulation o Plays a role in processing auditory and visual information and in orienting reflexes 2. Pons (latin:bridge) o Main connection between brain and cerebellum o Important for some eye movement, movements of the face o Modulate arousal and pain 3. Medulla o Essential for life o Houses cell bodies of many of the 12 cranial nerves, providing sensory and motor innervations to o Controls vital functions: Heart rate, blood pressure, respiration (breathing), arousal The 4 Lobes: Frontal Lobe: 1. Prefrontal Cortex: Executive functions, decision-making, emotional regulation 2. Primary Motor Cortex: Voluntary movement control 3. Premotor Cortex: Movement planning and coordination 4. Broca´s Area: Language production 5. Supplementary Motor Area: Movement initiation and coordination 6. Orbitofrontal Cortex: Risk assessment, decision-making, and social behavior 7. Frontal Eye Fields: Eye movement control and visual attention Parietal: Receive and interpret signals 1. Primary Somatosensory Cortex: Touch, temperature, and pain 2. Somatosensory Association Cortex: Interpretation of sensory input, hand-eye coordination 3. Superior Parietal Lobule: Spatial awareness, body position 4. Inferior Parietal Lobule: Integrates sensory info for complex tasks like language and math Temporal: 1. Primary Auditory Cortex: Sound processing 2. Wernicke’s Area: Language comprehension 3. Hippocampus: Memory formation 4. Amygdala: Emotional processing 5. Fusiform Gyrus: Facial and object recognition Occipital: 1. Primary Visual Cortex (V1): Basic visual processing 2. Visual Association Areas: Complex visual interpretation 3. Dorsal/Ventral Pathways: Location/motion (dorsal) and object recognition (ventral) Unitsensory cortex Multisensory cortex The Diencephalon: 1.Thalamus Divided into two parts – one on left hemisphere one on right Both parts are connected by a bridge of gray matter called massa intermedia receives sensory information from the body and directs it to the appropriate regions of the cerebral cortex for further processing (except for some olfactory inputs) plays a role in coordinating motor signals between the cerebral cortex and other parts of the brain involved in regulating the sleep-wake cycle and levels of consciousness è helps modulate the brain’s alertness and attention levels by controlling the flow of sensory information during sleep 2. Hypothalamus maintains internal balance by regulating body temperature, hunger, thirst, and fluid balance monitors blood parameters and initiates responses to keep these parameters within narrow limits Through its connection to the pituitary gland it releases and regulates hormones that influence growth, stress responses, reproduction, and metabolism plays a role in emotional responses, as it is connected to the limbic system LG3) What are the names of the a:ected areas and their functions? Phineas Gage: a?ected area: Ventromedial – ventromedial prefrontal cortex - located in the frontal lobe Function: - helps process and regulate emotions in response to social interactions, contributing to socially appropriate behavior, empathy, and moral judgment - crucial for decision-making processes - essential for understanding reward value and risk assessment - supports moral judgment by helping individuals understand the social implications of actions and behaviors - self-referential thinking, such as reflecting on one’s own beliefs, past experiences, and sense of identity - supports the ability to suppress impulsive reactions in favor of more considered, adaptive responses “Tan”: A?ected area: Broca´s Area Located in the frontal lobe on the part of the left hemisphere Function: Language Production HM A?ected area: medial temporal lobe – hippocampus Function: Memory formation LG4) Two di:erent examples of brain damage and their e:ects Lobotomy: Lesional surgery on the brain -> Now-Obsolete-Surgical procedure ( surgical procedure is no longer used) Developed by António Egas Moniz in 1936 Mostly performed in 1930s-1950s Severing connections between prefrontal cortex and deeper brain areas ( like the thalamus and limbic system, which are involved in emotion ) Goal: To reduce symptoms of mental illnesses, especially extreme mood swings, hallucinations and aggression 1. Prefrontal Lobotomy (Standard): Small holes were drilled into the skull on the sides or top, then a surgical tool was used to cut brain tissue 2. Transorbital Lobotomy (Ice-Pick Lobotomy): Long, thin instrument (looked like an ice-pick) was inserted through the eye socket -> pushed up to the frontal lobe -> moved around to damage the neural pathways ->this method was quicker and often done with minimal anesthesia Result: - Personality Change: Patients could become apathetic, lose interest in life or become indi?erent - Loss of Cognitive Abilities: Decision-making, social skills and emotional expression were often impaired - Physical Side E:ects: Some patients experienced seizures, incontinence ( lack of control over urination) or other physical issues due to brain damage Split Brain: Sometimes done to treat severe epilepsy Corpus Callosotomy: Severing of the corpus callosum ( bundle of nerve fibers that connects the brain´s two hemispheres ) This e?ectively isolates the two hemispheres preventing them from communicating with each other Procedure limits seizure activity to one hemisphere, making it more manageable EOects of a Split Brain: splits visual perception, but does not create two independent conscious perceivers within one brain - Functions remain with a split brain, but each hemisphere can only process information from the side of the body they control directly o information presented to one side of the body or one visual field may only be processed by the opposite hemisphere o For example: if an object is shown to the left visual field, it is processed by the right hemisphere. Since the right hemisphere lacks direct language capabilities, the patient may not be able to name the object, even though they can draw or recognize it by touch. -> each hemisphere can process information independently but only the left hemisphere can express it verbally - Split-brain studies have provided valuable insights into lateralization of brain function - describes how specific functions are localized to either the left or right hemisphere of the brain LG5) Example of a single and double dissociation Single Dissociation: Double Dissociation: Episodic memory vs. procedural memory Hippocampus vs basal ganglia, cerebellum Cognitive control 1. Explain the case of Phineas Gage (what was the actual explanation) 1848: survived a massive injury by an iron rod (6 kg, 1.09 m in length, maximum diameter 31.75 mm, 6 mm at the tip) which went through his left cheek and head caused by an accidental explosion Early Reconstructions: Bigelow (1850): - Drilled a whole through a “ordinary skull” to enlarging it until the iron rod would fits through to simulate gages lesion - Could not recreate gages lesion, since his was smaller Damasio (1994): - Reconstructed how the rod went through gages skull with a 3D computer- generated “standard skull” - Concluded rod exited gages skull on the right side - suggesting it passed through the sagittal sinus superior (SSS) along with the prefrontal area, orbitofrontal cortex, and anterior part of the cingulate gyrus bilaterally New Insights Gages medical records didn´t match with the previous studies didn´t match Findings: - brain injury was limited to the left frontal lobe - did not involve the ventricular system - Only the medial and lateral orbitofrontal and dorsolateral prefrontal areas of the left frontal lobe were directly impacted by the incident (areas associated with decision-making and impulse control - Injury aRected approximately 11% of his white matter and 4% of his grey matter è Relatively low percentage of damaged suggest that crucial parts of the brain and pathways stayed in tact - Recovery: - (changes in behavior my be a bit exaggerated) Gage later on adapted and worked successfully as a stagecoach driver in Chile 2. What is cognitive control? also known as executive function ð Refers to a set of psychological processes enabling goal-oriented behavior ð Allows individuals to use their perception, knowledge, and objectives to influence their choices and action eJectively Key characteristics: Goal-Oriented Behavior: Cognitive control provides the mechanism by which goals shape § Maintaining focus on relevant information § Inhibiting distractions or irrelevant stimuli § Monitoring process towards goal § Flexibly shifting between sub-goals if needed Dependence on Working Memory: connecting perception, long-term memory, and action to facilitate decision- making and goal-directed behavior Neurological Basis: § Prefrontal cortex plays a crucial role, particularly in accessing and maintaining active representations of information § Dorsolateral Prefrontal Cortex (DLPFC): Implements top-down control by managing attention, planning, and working memory § Anterior Cingulate Cortex (ACC): Acts as a monitoring system, detecting conflicts or errors and signaling the need for increased control Conflict detection & Regulation: § When competing or mutually exclusive representations (e.g., actions or thoughts) arise, the ACC identifies the conflict § In response, areas like the DLPFC are activated to reduce the conflict and optimize performance 3. Examples of cognitive control 1. Stroop Task 2. Action Inhibition Task: Description: Participants are required to stop a pre-planned action, when a stop-signal is presented Role of Cognitive Control: task tests the ability to halt automatic responses, demonstrating inhibition Brain Activity: § right inferior frontal gyrus (IFG) plays a key role in initiating inhibitory control 3. N-Back Task: Description: Participants are shown diRerent stimulus in a sequence, their task is to identify the earlier presented stimuli N Role of Cognitive Control: § Requires maintaining task-relevant information (working memory). § Involves ignoring irrelevant stimuli and updating memory representations Brain Activity: § Dorsolateral Prefrontal Cortex (DLPFC) is heavily involved, showing heightened activity when task diRiculty increases 4. Decision Making and Value Assessment: Description: Evaluating the trade-oRs between immediate and delayed rewards, such as choosing between a smaller, immediate reward or a larger, delayed one Role of Cognitive Control: Involves suppressing impulsive decisions in favor of long-term goals Brain Activity: § The orbitofrontal cortex (OFC) encodes the value of options § The lateral prefrontal cortex (LPFC) interacts with the OFC to prioritize long-term over short-term reward 5. Dynamic Filtering Task: Description: Tasks requiring selective attention to relevant information while ignoring irrelevant data Role of Cognitive Control: Filtering irrelevant stimuli and maintaining focus on relevant cues Brain Activity: § The lateral prefrontal cortex ( LPFC) facilitates dynamic filtering, enhancing the activation of task-relevant representations while suppressing irrelevant ones 6. Multitasking: Example: Managing unrelated goals, such as driving while conversing Role of Cognitive Control: ERiciently switching attention between tasks and maintaining priorities Experimental Insights: Experienced multitaskers, such as avid video game players, often perform better due to strengthened cognitive control networks 4. The Prefrontal Cortex’s role in cognitive control Two Prefrontal control systems First: LPFC, OFC, and FP, supports goal-oriented behavior constitute a working memory system that recruits and selects task- relevant information. planning; simulating consequences; and initiating, inhibiting, and shifting behavior Second: MFC guiding and monitoring behaviour works in tandem with the rest of the prefrontal cortex, monitoring ongoing activity to modulate the degree of cognitive control needed to keep behaviour in line with current goals. 5. Can brain lesions cause personality changes? Personality refers to the unique set of characteristics, patterns of thinking, feeling, and behaving that distinguish one individual from another. It encompasses a person’s consistent traits, emotional responses, attitudes, and behaviors over time and across different situations. Personality is shaped by a combination of biological factors (such as genetics), environmental influences (like upbringing and experiences), and social interactions. It is often studied through theories like the Big Five personality traits (openness, conscientiousness, extraversion, agreeableness, neuroticism) or psychoanalytic, humanistic, and behavioral perspectives. EZects of frontal lobe lesions in patients: Perseveration→ Patients that persist in a response even after being told that it is incorrect Apathetic Distractible impulsive unable to make decisions Unable to plan actions Unable to understand the consequences of their actions Unable to organise and segregate the timing of events in memory Unable to remember the source of their memories Unable to follow rules. disregard social conventions unable to complete a plan socially inappropriate disinhibition syndrome -> damage to ventral and medial portions of the frontal lobe -> normal cognitive functions, perform normally on laboratory tests of response selection and working memory -> symptoms: constant movement which is not channeled toward productive activities, euphoric or manic with abnormal sense of humor, fail to respond to social cues 6. How does the Stroop task work? (role, conditions, etc) The Stroop task involves naming the ink colour of a word. In congruent conditions, the word and ink colour match (e.g., “RED” in red ink), while in incongruent conditions, they differ (e.g., “RED” in blue ink). Naming the ink colour in incongruent conditions requires more cognitive control to overcome the automatic tendency to read the word. The task is used to study conflict monitoring and cognitive control processes. Monitoring, inhibition and shifting rules is needed ACC detects conflict, DLPFC resolves if by enhancing attention to the relevant stimulus dimension (color) ACC -> detects errors and monitors conflicts DLPFC -> prioritizes the instructed task over the automatic task 7. Which brain areas are involved in cognitive control? primary motor cortex secondary motor cortex (lateral premotor cortex, supplementary motor area) prefrontal cortex (PFC) lateral prefrontal cortex (LPFC), frontal pole (FP), orbitofrontal cortex (OFC), medial frontal cortex (MFC)) 8. Try to understand what the diagram means. Colored error lines -> feedback loops ACC plays important role to detect conflict -> when it detects mismatch it signals the need for increased control -> DLPFC resolves the conflict Psychiatry & Behavioral Sciences, Stanford Medical School Care System, Palo Alto, CA and Dr. Ahmad Salehi, Dept. of Language and Courtesy of Dr. Sarah Moghadam, VA Palo Alto Health Lateralization Silencing the Inner Voice We all have an inner voice: that sometimes-annoying mental narrator whose dialog wanders from calm abstraction (“What is love?”) to insistent directives (“Pizza now!”) to harsh self-criticism (“Way to go, fool!”). Some researchers, observing that the develop- 19 ment of a child’s sense of self is inextricably linked to the acquisition of language, sug- gest that the inner voice is essential for the self-awareness that defines our conscious- ness. Nevertheless, sometimes we wish that Inner-Me would just shut up for a while. But what would life be like if our inner voice actually were silenced? This is just what happened to Tinna Geula Phillips. Suddenly one day, Tinna lost the ability to com- municate in any of the six languages (yes, six) that she had mastered; a devastating neurological event. But even more profound was the immediate silencing of Tinna’s inner voice. For several months, Tinna was unable to process thoughts in the way we take for granted. Tinna’s internal silence had robbed her of a critical tool for organizing her activities, weighing her emotions, processing abstract concepts, and considering her memories. She would later describe the experience as a near-total loss of identity. What could have happened to cause Tinna to lose the ability to talk to anyone, even herself? Faces, coloration, smells, sounds—many species use physical and behavioral signals to engage in communication, the transmission of information between individuals. But we humans may be alone in our use of language, the highly specialized form of communication in which arbitrary symbols or behaviors are assembled and reas- sembled in almost infinite variety and associated with a vast range of things, actions, and concepts. Because the speakers of a language all understand the same strict set of rules, or grammar, language allows us to assemble and share information on any topic, linking thinkers of the past with those of the future. Our linguistic and aes- thetic perception of the world gives us a mental life that appears to be unique among animal species. In almost everyone, verbal abilities are especially associated with the left hemi- sphere of the brain, whereas the right hemisphere plays a special role in complex spatial cognition, our ability to process geometric relationships, to navigate, and to understand the spatial relationships between objects. We begin the chapter with the neuroscience of cerebral asymmetry, before turning to a more focused over- view of the acquisition and use of speech and language, and the brain mechanisms underlying this uniquely human capability. Much of what we know about human brain organization is derived from studies of people who have sustained strokes and other forms of brain injury, so we conclude with a section on the sometimes surprising ability of the damaged brain to recover and adapt. Go to Brain Explorer bn9e.com/be19 BRAIN ASYMMETRY AND LATERALIZATION OF FUNCTION The special role of the left hemisphere for language functions was well established by the mid-twentieth century, and it was often referred to as the dominant hemisphere, in recognition of the preeminence of language in our cognitive lives (Finger, 1994). However, the right hemisphere does not just idly sit within the skull, awaiting an oc- casional call to duty. In fact, most researchers have abandoned the notion of cerebral dominance in favor of models of hemispheric specialization, or lateralization. This emphasis implies that some functional systems are connected more to one side of the brain than the other—that is, that functions become lateralized—and that each hemisphere is specialized for particular ways of working. Keep in mind, however, that almost all types of behavior, from manual skills to intellectual activity, are per- formed better by the two hemispheres working together than by either hemisphere working alone. The idea that the two hemispheres are so different that they need separate instruction, or that people can have personalities that are “right-brained” (supposedly more random, intuitive, and creative) or “left-brained” (ostensibly logi- cal, sequential, and analytical), are flaky notions that lack any scientific basis. 19.1 The Left and Right Hemispheres Are Different Learning Objectives After studying this section, you should be able to: 19.1.1 Distinguish between communication and language. 19.1.2 Describe experimental techniques for studying the left and right hemispheres independently of one another. 19.1.3 Summarize the apparent cognitive specializations of each hemisphere as revealed by behavioral testing. 19.1.4 Speculate about the evolutionary origins of handedness, and discuss the relationship of handedness to cerebral lateralization. The discovery that some brain functions are lateralized should not be especially sur- prising; after all, other body organs also show considerable asymmetry between the right and left sides. For example, in all vertebrates the heart is slightly to the left of the midline and the liver is on the right. Sometimes people with a defect in one of the genes involved will develop with their organs reversed, a condition called situs inversus (Casey and Hackett, 2000). When we study the behavior of healthy people, cerebral lateralization of function is masked by the rich neural connections between communication!Information transfer the hemispheres: they communicate with each other so quickly and so thoroughly between two individuals. that they seem to act as one. But by studying people whose hemispheres have been language!The most sophisticated disconnected, researchers have been able to study the functioning of each hemi- form of communication, in which a set of sphere in isolation from the other. arbitrary sounds, tokens, or symbols can be arranged according to a grammar in Disconnection of the cerebral hemispheres reveals order to convey an almost limitless variety their individual specializations of concepts. Some rare and unfortunate people develop severe epilepsy that is very difficult to grammar!All of the rules for usage of control with medication. Their frequent seizures start in one hemisphere and then a particular language. spread to the other hemisphere through the corpus callosum —the huge white mat- lateralization!The tendency for the ter pathway consisting of hundreds of millions of axons connecting the two hemi- right and left halves of a system to differ spheres. A treatment of last resort is to surgically split the two hemispheres, severing from one another. In cerebral lateralization, one cerebral hemisphere is specialized for the corpus callosum to prevent the spread of the seizure discharges from one hemi- a particular intellectual function. sphere to the other. Due to its extreme invasiveness, and the development of better corpus callosum!The main band of pharmacological alternatives, split-brain surgery has remained a very rare procedure, axons that connects the two cerebral with most people receiving only partial splits (transection of a minority of the callo- hemispheres. sum). Only about ten people with complete transections of the corpus callosum have split-brain individual!An individual been fully studied by researchers (A. M. Gazzaniga, 2008). In these split-brain indi- whose corpus callosum has been viduals, the operation had its expected effect in reducing the frequency and severity severed, halting communication between of their seizures. But it also changed their behavior in ways that presented a unique the right and left hemispheres. research opportunity: by analyzing the cognitive, perceptual, emotional, and motor 632! C HAP T E R 1 9 behavior of split-brain individuals, researchers were able to catalog the individual specializations of the newly isolated cerebral hemispheres. In Nobel Prize–winning research begun in the 1960s, Roger Sperry applied tech- niques that he had perfected in split-brain cats to the study of split-brain humans. The researchers realized that by exploiting the organization of the sensory systems, they could direct stimuli exclusively to one hemisphere or the other. For example, stimuli felt with the left hand, or seen only in the left visual field, stimulate activity in sensory neurons of the right hemisphere. Normally, information about the stimuli would immediately be shared with the other hemisphere, but because the corpus callosum is cut in split-brain individuals, most of the information received by one hemisphere of the brain remains trapped there and cannot travel to the other hemi- sphere. By controlling stimuli in this fashion—selectively presenting them to one hemisphere or the other—the experimenter can test the processing specializations of each hemisphere in isolation. In some studies of split-brain individuals, words were projected to either the left or the right hemisphere; that is, printed stimuli were presented in either the right or the left side of the visual field. The results were dramatic. (A) Control participant Split-brain individuals could easily read and Fixation point visual Right v verbally communicate words projected to the Left ld i field sual fi e left hemisphere (via the right visual field), but no such linguistic capabilities were evident At the optic chiasm, when the words were directed to the right half of the fibers “Key” hemisphere (FIGURE 19.1). Although the right from each eye cross hemisphere in split-brain individuals does over, so the left Language hemisphere sees the have minor linguistic capabilities—for ex- right visual field, center ample, recognition of very simple words, and and vice versa. processing of emotional content in verbal ma- terial (Zaidel, 1976)—in most people, vocabu- Intact lary and grammar are the exclusive domain of corpus the left hemisphere (left-handers occasionally callosum show a reversed asymmetry). The capabilities of the “mute” right hemisphere had to be tested by nonverbal means. For example, a picture of a key might be projected to the left visual field and so reach only the right cortex. The individu- Visual cortex als would then be asked to touch several (B) Split-brain participant 19.1 Testing a Split-Brain Individual! Words or pictures projected to the left visual field activate the right visual cortex. (A) In “Key” intact individuals, activation of the right visual cortex excites corpus callosum axons, which “?” transmit verbal information to the left hemi- sphere, where the information is analyzed and language is produced. (B) In split-brain indi- viduals, stimuli from the left visual field reach the right-hemisphere visual cortex via the sub- cortical visual pathways (they are independent Severed corpus of the corpus callosum). However, the split callosum corpus callosum prevents right-hemisphere visual areas from communicating with the lan- guage areas of the left hemisphere, so verbal responses to the stimuli are impossible (left). In contrast, split-brain individuals are able to respond verbally to stimuli appearing in the right visual fields, because interhemispheric transfer is not required (right). LANG UAG E AN D LATERAL IZAT ION ! 633 19.2 Callosal Agenesis!In con- (A) Corpus callosum (B) trast to the typical brain in part A, the brain in part B failed to develop any vestige of a corpus callosum. Despite the absence of the hun- J. M. Tyszka et al., 2011. J Neurosci 31: 15154–15162; dreds of millions of axons that pass images courtesy of Lynn Paul and Mike Tyszka between the hemispheres through the corpus callosum, the activity of the two hemispheres of this indi- vidual remained coordinated. different objects that they could not see and hold up the correct one. Such a task could be performed correctly by the left hand (controlled by the right hemisphere) but not by the right hand (controlled by the left hemisphere). So in this case, the left hemisphere literally does not know what the left hand is doing! In general, these and other studies with split-brain individuals provided early evidence that, in most people, the right hemisphere is specialized for processing spatial infor- mation. Right-hemisphere mechanisms are also crucial for face perception, for processing emotional aspects of language, and for controlling attention (as we discussed in Chapter 18). As many as one in 4000 people are born either partially or totally lacking a cor- pus callosum. Although some individuals will show some behavioral consequenc- es of this callosal agenesis (FIGURE 19.2), in general they lack the constellation of striking neuropsychological changes evident in surgical split-brain individu- als (Sotiriadis and Makrydimas, 2012). Somehow, the developing nervous system compensates for the loss of the main connection between the hemispheres, per- haps by strengthening alternative subcortical routes of transmission (Tyszka et al., 2011). This observation highlights the incredible plasticity of the developing brain. The two hemispheres process information differently in most people Most research on brain asymmetry in healthy people focuses on two sensory mo- dalities: hearing and vision. That’s because researchers have devised clever proce- dures for directing auditory or visual stimuli mostly to one hemisphere or the other and then inferring hemispheric specializations from the behavioral responses made by the participants. THE RIGHT-EAR ADVANTAGE!Through earphones, we can present different sounds to the two ears at the same time; this process is called dichotic presentation. So, for example, the study participant may hear a particular speech sound in one ear and, at the same time, a different vowel, consonant, or word in the other ear. The task for the participant is to try to identify or recall both sounds. Although this procedure may seem designed to produce confusion, most data from dichotic presentation dichotic presentation!The simul- experiments Behavioral indicate Neuroscience 9E that right-handed persons identify verbal stimuli delivered to taneous delivery of different stimuli to Breedlovethe right ear more accurately than verbal stimuli simultaneously presented to the Sinauer Associates Dragonfly Media Group the right and the left ears. left ear. This result is described as a right-ear advantage for verbal information. In tachistoscope test!A test in which Breedlove9e_19.02.ai contrast, about 50% of left-handed people reveal a reverse pattern, showing a left-ear Date 07-23-19 stimuli are very briefly exposed in either advantage—more-accurate performance for verbal stimuli delivered to the left ear. the left or right visual half field. As a consequence of the preferential connections between the right ear and left planum temporale!A region of hemisphere, the right-ear advantage for verbal stimuli is probably a reflection of the superior temporal cortex adjacent to left hemisphere’s specialization for language (FIGURE 19.3). Although we can nor- the primary auditory area. mally use either ear for processing speech sounds, speech presented to the right ear 634!C HAP T E R 19 19.3 The Right-Ear Advantage in Dichotic Presentation!(A) A word “ma” delivered to the left ear results in stronger stimulation of the right auditory (A) cortex. (B) A word delivered to the right ear results in stronger input to the left hemisphere. (C) When words are delivered to both ears simultaneously, the word to the right ear is the one usually perceived because the right ear has more-direct connections to the left hemisphere. (After D. Kimura, 1973. Left hemisphere Auditory speech zone cortex Sci Am 228: 70–78.) ma ma in dichotic presentation tests exerts stronger control over language ma mechanisms in the left hemisphere than does the speech simulta- neously presented to the left ear (Kimura, 1973). The competition between the left- and right-ear inputs is the key; presentation of Brainstem speech stimuli to one ear at a time (monaural presentation) does auditory Left Right not produce a right-ear advantage. And the right-ear advantage regions in right-handers is restricted to particular kinds of speech sounds: Although sounds presented to either ear reach both sides of the those made for consonants like b, k, m, and p produce a right-ear ef- brain, the auditory information is mostly processed by the fect, but vowel sounds do not (Tallal and Schwartz, 1980). contralateral hemisphere (see Chapter 6). So, verbal information presented to the left ear is first processed by the right auditory cortex and then transmitted to speech systems in the left VISUAL PERCEPTION OF LINGUISTIC STIMULI!A visual hemisphere. Participant repeats the word. analog to the dichotic listening task is the tachistoscope test, in which a specialized apparatus (named, surprisingly, a tachistoscope) “pa” (B) is used to present stimuli very briefly to either the left or right visual field (see Figures 10.10, 10.11, and 19.1). If the stimulus exposure lasts less than 150 milliseconds (ms) or so, the input is received by only the contralateral hemisphere because there is not enough time for the eyes to shift their position to fix the stimulus in central vision. In healthy humans, of course, information may subsequently be passed to the other hemisphere via the corpus callosum, but the pa pa important thing is that, as with dichotic presentation, the stimuli have preferential access to one hemisphere. Most tachistoscopic studies confirm the general division of la- bor between the hemispheres. Verbal stimuli (words and letters) are recognized more accurately when they are presented to the right visual field (and therefore left hemisphere) than when they are pre- sented to the left visual field/right hemisphere. Conversely, non- Verbal information presented to the right ear is processed verbal visual stimuli (such as faces or geometric forms) presented by the left auditory cortex and then passed directly to speech systems within the same hemisphere. to the left visual field/right hemisphere are better recognized than the same stimuli presented to the right visual field/left hemisphere. “pa” (C) Simpler visual processing, such as detection of light, hue, or simple patterns, is performed equivalently by both hemispheres. The left and right hemispheres differ in their auditory specializations Anatomical studies of primary auditory cortex in the left and right hemispheres are consistent with the view that the two play differ- ma pa ma ma pa ent roles in auditory perception. In one early postmortem study of auditory cortex, the planum temporale —an auditory region on the superior surface of the temporal lobe—was found to be larger in the left hemisphere than in the right in most of the brains studied (FIGURE 19.4A AND B) (Geschwind and Levitsky, 1968). In only 11% of adults was the right side larger. The planum temporale in- cludes part of Wernicke’s area, which as we’ll see shortly is crucial When conflicting information goes to both ears, the information for speech, so it seems likely that the larger left planum temporale to the right ear reaches the left hemisphere’s speech system first. Subject repeats only the right-ear information. is related to that hemisphere’s language specialization. Direct evi- dence of this relationship, however, remains somewhat elusive: in one MRI study, for example, asymmetry of the planum temporale did not correlate with direct measures of language lateralization (Dorsaint-Pierre et al., 2006). LA NG UAGE AN D LATERAL IZ ATION ! 635 (A) (B) Anterior (C) Hemispheres: Left- Temporal pole Right- Left Right If we view the hemisphere hemisphere Musician brain from above as if the overlying cortex were trans- Primary Primary parent, we see that auditory auditory in the musician cortex cortex with perfect pitch the planum temp- Planum Planum orale (red) is much temporale temporale larger in the left hemisphere than in the right. Nonmusician Posterior In the nonmusician, 19.4 Structural Asymmetry of the Human Planum Temporale!(A) This diagram the asymmetry of shows the orientation of the brain section in part B. (B) The planum temporale (yellow) the planum temp- is on the upper surface of the human temporal lobe. (C) MRI images from the brain of orale is much less distinct. a musician with perfect pitch (top) and the brain of a nonmusician (bottom) show some differences: in the musician, the left planum temporale is larger. (After G. Schlaug et al., 1995. Science 267: 699–701.) Courtesy of Gottfried Schlaug As in adults, the left planum temporale is larger than the right in the brains of infants (Wada et al., 1975), in agreement with the observation that speech ac- tivates the left cerebral hemisphere more than the right in infants as young as 3 months. This provides more evidence that cerebral specialization for language probably begins prenatally without the need of speech experience, because the asymmetry is evident before any substantial such experience has occurred. In fact, even in human fetuses of just 12–14 weeks of gestation, a variety of genes show asymmetrical expression in the brain (T. Sun et al., 2005). The planum temporale tends to be larger on the left than on the right in chimpanzees too (Gannon et al., 1998), and Broca’s area—another cortical speech zone, as we’ll see a little later—is larger on the left than on the right in chimps, bonobos, and gorillas, as well as in humans (Cantalupo and Hopkins, 2001). Furthermore, just as our left hemi- sphere is more activated than the right when we hear speech rather than other sounds, the left hemisphere of monkeys is more activated than the right when they hear monkey vocalizations rather than human speech (Poremba et al., 2004), and their perception of monkey vocalizations is far more impaired by left- than right-hemisphere damage (Heffner and Heffner, 1984). These results suggest that other primates also possess a left-hemisphere specialization for some forms of communication (but not necessarily language). In contrast, the auditory areas of the right hemisphere play a major role in the per- ception of music. Musical perception is impaired particularly by damage to the right hemisphere (S. Samson and Zatorre, 1994), and music activates the right hemisphere more than the left (Zatorre et al., 1994). But perfect pitch (the ability to identify any musical note without comparing it to a reference note) seems to involve the left rather than the right hemisphere. Schlaug et al. (1995) made MRI measurements of the pla- num temporale in three kinds of participants, all right-handed (because the larger size of the left planum temporale is seen especially in right-handed individuals): (1) musicians with perfect pitch, (2) musicians without perfect pitch, and (3) nonmusi- cians. The size of the left planum temporale was twice as large in musicians with perfect pitch than in nonmusicians (FIGURE 19.4C). The size of the left planum temporale in musicians without perfect pitch was intermediate, but closer to that of nonmusicians. Because perfect pitch requires both verbal ability (to name the pitch) and musical ability, perhaps it is not surprising to find that, like language, perfect pitch is associated with the left hemisphere. Despite these data, we cannot assign the perception of speech entirely to the left hemisphere and the perception of music entirely to the right hemisphere. We have seen that the right hemisphere can play a role in speech perception even in people in whom the left hemisphere is dominant for speech. In addition, the 636! C H AP T E R 19 perception of emotional tone-of-voice aspects of language, termed prosody, is prosody!The perception of emotional a right-hemisphere specialization. Furthermore, although damage to the right tone-of-voice aspects of language. hemisphere can impair the perception of music, it does not abolish it. Damage to both sides of the brain can completely wipe out musical perception (S. Samson and Zatorre, 1991). Thus, even though each hemisphere plays a greater role than the other in different kinds of auditory perception, the two hemispheres appear to collaborate in these functions, as well as in many others. Handedness is associated with cerebral lateralization Various surveys of hand usage, such as the incidence of left-handed writing in Amer- ican college populations (Spiegler and Yeni-Komshian, 1983), suggest that 10–15% of the population is left-handed. This predominance of right-handedness in humans is probably an ancient trait. In ancient cave paintings, people were generally portrayed clutching objects in their right hands, and Stone Age tools seem to be shaped for the right hand. Skull fractures of animals preyed on by ancient humans are usu- ally on the animal’s left side, so anthropologists conclude that most attackers held a club in their right hand. Handedness is a trait we share with our primate rela- tives: population-level hand preference is observed in gorillas, chimps, and bonobos (they’re mostly right-handers) and orangutans (mostly lefties) (Hopkins et al., 2015; P. F. MacNeilage et al., 2009). Handedness directly reflects cerebral lateralization by virtue of the preferential connection of each hand with the contralateral hemisphere. Some scientists be- lieve that handedness, and the underlying hemispheric asymmetry with which it is associated, originated in the differential use of the limbs for many routine tasks. Picture early humans hunting. One hand holds the weapon and provides power; the other is used in more-delicate guidance or body balance. Perhaps powerful throwing, a strongly right-handed behavior in most right-handed people, was a particularly important evolutionary development, providing obvious advantages in hunting and defense. In time, the left-hemisphere neural specializations need- ed for programming throwing movements may have been co-opted to control lan- guage as well (Watson, 2001). However, other vertebrates—including toads (Val- lortigara et al., 1999), crows (Hunt et al., 2001), walruses (Levermann et al., 2003), and chimpanzees (Corp and Byrne, 2004)—have been found to show preferences for using one limb or the other for particular behaviors, suggesting that handed- ness has additional adaptive advantages. (For other examples of the evolution of asymmetry, see A STEP FURTHER 19.1: EVOLUTION FAVORS ASYMMETRY UNDER SOME CONDITIONS on the website.) Some researchers go further, arguing that hemispheric asymmetry reflects an ancient and ubiquitous left-right division of labor that, as a matter of neural processing efficiency, arose in the first vertebrates, hundreds of millions of years ago (P. F. MacNeilage et al., 2009). This view is bolstered by the discovery that in mice, left-hemisphere neurons differ from their right-hemisphere counterparts in both the expression of neurotransmitter recep- tors and physiological functioning (M. M. Kohl et al., 2011; Shipton et al., 2014). Handedness may have a genetic component, but it doesn’t appear to be deter- mined by a single gene. One possibility is that handedness is governed by genes that play a role in asymmetry throughout the body, ranging from the shape of internal organs to the pattern of hair whorls on the scalp (Corballis, 2014; Klar, 2003). One gene that may contribute to handedness is LRRTM1 (Francks et al., 2007; Leach et al., 2014), a gene associated with glutamate neurotransmission that is also implicated in schizophrenia. Perhaps the co-occurrence of schizophrenic symptoms in a small minority of left-handers helped give rise to the historical negative view of left-handedness. Additionally, several studies have found that one form of the gene PCSK6 is associated with right-handedness; PCSK6 has a role in the development of the left-right axis in mammals, including the asymmetrical placement of internal organs (Brandler and Paracchini, 2014). Because left-handedness is unusual, historical accounts attribute various nega- tive characteristics to left-handed people, and the Latin word for “left-handed”— sinistra—provides the root of not only the English word sinistral, meaning L ANG UAGE AND LATERALIZ ATION ! 637 “left-handed,” but also sinister. The view that left-handed people are somehow ab- normal was not uncommon in the past and even found occasional support in re- search, such as the observation that left-handedness may be more frequent in clinical populations than in the general population (Silva and Satz, 1979), perhaps as a re- sult of developmental reorganization after brain injury. However, studies of achieve- ment, ability, and cognitive function in thousands of school-age children conclu- sively showed that left-handed children do not differ from right-handed children on any measure of cognitive performance (Hardyck et al., 1976). Techniques that allow the study of each hemisphere in isolation, such as the Wada test, have reliably shown that as in right-handers, language is vested in the left hemisphere in the great major- ity of left-handers (BOX 19.1). However, those rare individuals in whom language is known to be lateralized to the right hemisphere are more likely to be left-handed B OX 19.1 The Wada Test Given the incidence of language (A) impairment after unilateral brain dam- 2 …temporarily shuts down the age, scientists estimate that 90–95% cerebral hemisphere on the of humans have a left-hemisphere same side, thereby revealing specialization for language. But how the functions performed by that hemisphere. can we be certain which hemisphere is specialized for language (or other func- tions, such as spatial memory or music Right Left hemisphere hemisphere “I can still talk perception) in people without brain just fine, but I can’t damage? For example, it can be crucial seem to hold my to understand an individual’s specific left arm up.” lateralization prior to brain surgery. By injecting a short-acting anes- thetic (sodium amytal) into the carotid artery on one side (as shown in Figure A), it is possible to simulate a massive stroke, shutting down the entire hemi- sphere on that side for a few minutes 1 Injection of the (Wada and Rasmussen, 1960). This anesthetic sodium is just long enough to use behavioral amytal into the measures to document the specializa- carotid artery, via a catheter… tions of that hemisphere. The same can be done for the other hemisphere (B) 100 several minutes later. This Wada test confirms that most people have left-hemisphere spe- 80 Proportion of tested cialization for language, regardless individuals (%) of handedness. The reverse pattern 60 (right-hemisphere dominance for lan- guage) is rare, but when it occurs, it is 40 Language control more common in left-handed people Left hemisphere (Figure B). Comparable results can be Right hemisphere 20 Both hemispheres obtained in healthy people by using TMS (Knecht et al., 2002) or fMRI. 0 (B after C. M. Epstein et al., 2000. Left-handed Right-handed Neurology 55: 1025–1027.) people people 638! C HAP T E R 19 than right-handed. MRI studies likewise show that the planum temporale is more likely to show reduced or reversed asymmetry in left-handers (Steinmetz et al., 1991). There are some rare circumstances in which a change in handedness may oc- cur in adults. Two right-handed people who received double hand transplants, after losing their own hands in accidents, reportedly both became left-handers after the surgery (Vargas et al., 2009). One possible explanation is that the right hemisphere required less reorganization in order to take control of the contralat- eral hand and that reconnection of the left hemisphere to the new right hand was initially hampered by the strong preexisting cortical representation of the former right hand. After getting an early start, perhaps the right hemisphere/left hand simply outcompeted the left hemisphere/right hand. Of course, no one yet knows if this process bears any relationship to the normal establishment of hand prefer- ence, but it suggests a surprising degree of flexibility in handedness. 19.2 Right-Hemisphere Damage Impairs Specific Types of Cognition Learning Objectives After studying this section, you should be able to: 19.2.1 Itemize the types of deficits seen after right-hemisphere lesions, relating the deficits to specific anatomical locations within the hemisphere. 19.2.2 Describe prosopagnosia and summarize the brain’s face-processing network. When studied with behavioral or imaging techniques that highlight differences be- tween the hemispheres, people reliably demonstrate a right-hemisphere advantage for the processing of spatial stimuli. Geometric shapes and their relations, direction sense and navigation, face processing, imagined three-dimensional rotation of ob- jects held in the mind’s eye—these are a few examples of the kinds of stimuli that the right hemisphere preferentially processes. So it’s no surprise that right-hemisphere lesions—especially more-posterior lesions that involve the temporal and parietal lobes—tend to produce a variety of striking impairments of spatial cognition, such as inability to recognize faces, spatial disorientation, failure to identify objects by touch, or the complete neglect of one side of the body that we discussed in Chapter 18 (see Figure 18.19). The diversity of behavior changes following injury to the parietal lobe is related partly to its large expanse and its critical position, abutting all three of the other cortical lobes. The anterior end of the parietal region includes the postcentral gy- rus, which is the primary cortical receiving area for somatic sensation. In addition to alterations in touch sensitivity on the opposite side of the body, brain injuries in this area can produce deficits involving much more complex forms of sensory processing. For example, objects placed in the hand opposite the injured somato- sensory cortex can be felt but cannot be identified by touch and active manipula- tion. This deficit is called astereognosis (from the Greek a-, “not”; stereos, “solid”; and gnosis, “knowledge”). More-extensive injuries in the parietal cortex, beyond the primary somatosensory cortex of the postcentral gyrus, affect interactions between sensory modalities, such as visual or tactile matching tasks, which require the participant to visually identify an object that is touched or to reach for an object that is first perceived visually. astereognosis!The inability to In prosopagnosia, faces are unrecognizable recognize objects by touching and feeling them. Suppose one day you look in the mirror and someone unfamiliar is looking back at you. As incredible as this scenario might seem, some individuals develop exactly prosopagnosia!Also called face this problem following damage to specific brain sites. It’s a rare syndrome called blindness. A condition characterized by the inability to recognize faces. Acquired prosopagnosia (from the Greek prosop-, “face”; a-, “not”; and gnosis, “knowledge”), prosopagnosia is caused by damage or sometimes face blindness. People with prosopagnosia fail to recognize not only to the brain, particularly the fusiform their own faces but also the faces of relatives and friends. No amount of remedial gyrus. Developmental (or congenital) training restores their ability to recognize anyone’s face. In contrast, the ability to prosopagnosia is present from birth. LANG UAGE AN D LATERAL IZAT ION ! 639 Self Celebrity recognize objects may be retained, and the person may have no difficulty identifying familiar people by their voices. To people with prosopagnosia, faces simply lack mean- ing. No disorientation or confusion accompanies this con- dition, nor is there evidence of diminished intellectual abilities. Visual acuity is maintained, although the majority of people have a small visual-field defect—that is, an area of the visual field where they are blind. Most research indi- cates that the right hemisphere is more important than the left for recognizing faces. For example, shutting down the right hemisphere with anesthetic during the Wada test (see Box 19.1) can cause difficulty in recognizing faces, whereas Left hemisphere Composite face Right hemisphere anesthetizing the left hemisphere has less effect on facial anesthetized presented to participant anesthetized recognition. This effect can be especially evident for recog- nizing one’s own face (FIGURE 19.5). 5 5 Even with the left But when the Similarly, split-brain individuals do a better job of rec- hemisphere right hemisphere ognizing faces presented to the right hemisphere than to Number of participants 4 “asleep,” 4 is anesthetized, the left (M. S. Gazzaniga and Smylie, 1983). Still, data from participants participants recognize them- usually see the split-brain individuals and functional imaging tests make 3 3 selves in the celebrity face in it clear that both hemispheres have some capacity for recog- composite. the composite. nizing faces. Thus, although damage restricted to the right 2 2 hemisphere can impair face processing, the most complete cases of prosopagnosia are caused by bilateral damage. The 1 1 fusiform gyrus, a region of cortex on the inferior surface of the brain where the occipital and temporal cortices meet 0 0 Self Celebrity Self Celebrity (FIGURE 19.6), is a key component of a network specific to face recognition (Rezlescu et al., 2014) and seems to play 19.5 Use of the Right Hemisphere for Self-Face Recognition! a special role in recognizing stimuli within specific cate- Anesthetizing the left hemisphere in a Wada test (see Box 19.1) does gories (Grill-Spector et al., 2017; Weiner and Zilles, 2016). not interfere with study participants’ ability to recognize their own faces in pictures that are composites—of their faces and the faces Cases of prosopagnosia following brain damage almost al- of celebrities. But when the right hemisphere is anesthetized, the ways involve damage here. Prosopagnosia may be accom- participants’ facial recognition is impaired, especially for their own panied by additional forms of agnosia (an inability to iden- faces, leading to guesswork and more frequent identification of the tify items, in the absence of specific sensory impairments) composite faces as those of the celebrities. (From J. P. Keenan et (Gauthier et al., 1999). For example, bird watchers may lose al., 2001. Nature 409: 305, courtesy of Julian Keenan.) Frontal pole Temporal pole Brainstem 19.6 The Fusiform Gyrus!In this view from below the Fusiform brain, the cerebellum has been removed to reveal the region gyrus of cortex that normally lies opposite to it. The fusiform gyrus, at the juncture of the temporal and occipital lobes, is active during discrimination of objects in a large category, such as faces or birds or cars. Bilateral destruction of this region leads to prosopagnosia, the inability to recognize individual faces. Occipital pole 640! C HAP T E R 19 the ability to distinguish between avian species. Indeed, fMRI studies of healthy fusiform gyrus!A region on the inferior participants show that the fusiform region is activated not only when people are surface of the cortex, at the junction of identifying faces, but also when identifying birds, or cars, or individual members temporal and occipital lobes, that has been associated with recognition of faces. of many other categories (Gauthier et al., 2000). It remains to be seen exactly how many distinct categories are individually represented in the brain, and the extent agnosia!The inability to recognize to which brain regions other than the fusiform gyrus may participate (Connolly et objects, despite being able to describe them in terms of form and color. It may al., 2012; Haxby, 2006). occur after localized brain damage. Until recently, it was believed that prosopagnosia occurred solely as a result phoneme!A sound that is produced of brain damage (acquired prosopagnosia), but we now know that congenital (or for language. developmental) prosopagnosia—lifelong face blindness without brain damage—is surprisingly widespread. In fact, surveys indicate that about 2.5% of the general morpheme!The smallest grammatical unit of a language; a word or meaningful population is sufficiently impaired in processing faces to meet the criteria for con- part of a word. genital prosopagnosia (Duchaine et al., 2007; Kennerknecht et al., 2006), con- firming the widespread belief that some people are just “bad with faces.” This semantics!The meanings or interpretation of words and sentences congenital form of prosopagnosia appears to run in families, suggesting a genetic in a language. aspect to the disorder (Grüter et al., 2008). Congenital prosopagnosia is associated syntax!The grammatical rules for with diminished activation of the fusiform gyrus and reduced white matter con- constructing phrases and sentences nectivity in the ventral occipitotemporal cortex, in keeping with the anatomical in a language. findings in acquired prosopagnosia that we already discussed. At the other end pragmatics!In linguistics, the context of the spectrum, some people are exceptionally good with faces. These “super- in which a speech sound is uttered. recognizers,” who are about as good with faces as people with prosopagnosia are bad, are actively recruited by some police forces (Robertson et al., 2016; R. Russell aphasia!An impairment in language understanding and/or production that is et al., 2009). (You can learn more, and test your own facial recognition ability us- caused by brain injury. ing the Famous Faces test, at www.testmybrain.org.) 19.3 Left-Hemisphere Damage Can Cause Aphasia Learning Objectives After studying this section, you should be able to: 19.3.1 Distinguish among aphasia, apraxia, agraphia, and alexia. 19.3.2 Describe Broca’s area and the behavioral consequences of lesions that include this area.Identify Wernicke’s area, and describe the behavioral consequences of lesions that include this area. 19.3.3 Describe global aphasia, its causes and prognosis, and its relationship to concepts of personal identity. A human language is made up of basic speech sounds, or pho- nemes, that are assembled into simple units of meaning called unfathomable morphemes (FIGURE 19.7). Morphemes are assembled into words (the word unfathomable, for example, consists of the morphemes un-, fathom, and -able), which have meaning, termed semantics Morpheme Morpheme Morpheme in linguistics. In turn, the words are assembled into meaningful un fathom able strings (which may be complete sentences or just phrases) accord- (“not”) (“understand”) (“capable of”) ing to the language’s syntax (grammatical rules). Our speech is colored and clarified both by the context of the utterance, known as pragmatics in linguistics, and by the emotional tone and em- phasis, called prosody that we add to the things we say. Two phonemes: Five phonemes: Four phonemes: “u,” “n” “f,” “a,” “th,” “o,” “m” “a,” “b,” “u,” “l” We have known for millennia that localized brain damage can disrupt our use of the basic speech sounds and grammar, and in severe cases it results in profound impairment of language In English, some phonemes are depicted as a abilities, known as aphasia. Medical texts of ancient Egypt de- combination of letters, such as “th.” Words are scribe speech problems in people who had received blows to the strung together according to the grammatical side of the head (Finger, 1994), and many individual cases of rules of a language—the syntax—to communicate meaning to others. speech loss after strokes, war wounds, and other brain injuries were recorded over the years. Some 25–50% of all people who 19.7 Anatomy of a Word!A word can be broken down have a significant stroke will experience aphasia as a primary into morphemes—these are simple units of meaning and not symptom; that’s why speech difficulty is considered to be one of the same as syllables, which relate to pronunciation. Each the core warning signs of a stroke (see Figure 2.22), along with morpheme can be defined as an aggregate of phonemes, weakness or numbness on one side and dizziness, altered vision, which are simple speech sounds. LANG UAG E AN D LATERAL IZATION ! 641 paraphasia!A symptom of aphasia or confusion. And in agreement with the evidence of left-hemisphere specializa- that is distinguished by the substitution tion for language, 90–95% of aphasia cases are the result of damage to the left of a word by a sound, an incorrect word, cerebral hemisphere. Some individuals will lose almost all capacity for speech, an unintended word, or a neologism. but in cases where the left-hemisphere damage is less severe, the individual may neologism!An entirely novel word, instead show pronounced paraphasia —insertion of incorrect sounds or words— sometimes produced by a person along with labored, effortful speech production. At times entirely novel nonsense with aphasia. words—called neologisms —may be generated via the insertion or substitution of nonfluent speech!Talking with one or more phonemes. Conversation reveals another important aspect of speech: considerable effort, short sentences, its fluency or ease of production. Nonfluent speech is talking with considerable and the absence of the usual melodic effort, in short sentences, and without the usual melodic character of conversa- character of conversational speech. tional speech. agraphia!The inability to write. Most people with aphasia also have agraphia (trouble with writing) and alexia alexia!The inability to read. (trouble with reading). Brain damage that results in aphasia also produces a distinc- apraxia!An impairment in the ability tive motor impairment called apraxia (see Chapter 11), characterized by a great dif- to begin and execute skilled voluntary ficulty in executing precise sequences of movements that is not related to weakness, movements, even though there is no paralysis, a lack of coordination, or any kind of sensory problems. In fact, we’ll see muscle paralysis. shortly that some researchers view aphasia as being primarily the result of problems with motor mechanisms. Curiously, people with aphasia seem to outperform in one domain: they are better than unaffected people at detecting when someone is lying (Etcoff et al., 2000). Perhaps this sensitivity to lying results from a focus on facial fea- tures of speakers: their undamaged right hemisphere is important for face processing. Linking specific language disorders to damage in specific brain regions pro- vides clues about the organization of the left-hemisphere language network. FIGURE 19.8 shows some of the most important language-related regions of the left hemisphere of the brain. Damage in these regions tends to produce clusters of symptoms that define several distinct types of aphasia (TABLE 19.1; videos of Go to Activity 19.1 Speech and Language Areas of the Brain people with aphasia can be seen on the website). In the following sections, we look bn9e.com/ac19.1 in more detail at the three primary aphasias. Lesions of a left anterior speech zone cause nonfluent (or Broca’s) aphasia In the 1860s French neurologist Paul Broca (1824–1880) examined a man who had lost the ability to utter much more than the single syllable tan. Following postmor- tem analysis, Broca reported that this man, and other people with similar severe 19.8 Cortical Speech and Primary motor cortex Language Areas in Humans! Primary somatosensory cortex Lesions in the anterior frontal region called Broca’s area interfere with Supramarginal speech production; injury to an area gyrus of temporoparietal cortex called Wernicke’s area interferes with language comprehension; injury to the supramarginal gyrus interferes with repetition of heard speech. For most individuals, these language- related systems are found only in the left hemisphere. Angular gyrus Lesions in the left anterior Broca’s Damage to the temporoparietal area result in Wernicke’s area results in fluent nonfluent aphasia, aphasia, with impaired with reduced comprehension and garbled speech output. speech output. Primary auditory area 642!C HAP T E R 1 9 TABLE 19.1!Language Symptomatology in Aphasia Brain area Spontaneous Type of aphasia affected speech Comprehension Paraphasia Repetition Naming Nonfluent (Broca’s) aphasia Nonfluent Good Uncommon Poor Poor Fluent (Wernicke’s) aphasia Fluent Poor Common Poor Poor Global aphasia Nonfluent Poor Variable Poor Poor Conduction aphasia Fluent Good Common Poor Poor Subcortical aphasia Variable Variable Common Good Variable LLL R R R L R impairments of speech production, had sustained damage to a left inferior frontal Broca’s area!A region of the frontal region that now bears his name— Broca’s area (FIGURE 19.9). Left anterior lesions lobe of the brain that is involved in the that include Broca’s area often produce a type of aphasia known as nonfluent apha- production of speech. sia (or Broca’s aphasia). People with nonfluent aphasia have considerable difficulty nonfluent aphasia!Also called producing speech, talking only in a labored and hesitant manner. Reading and writ- Broca’s aphasia. A language impairment ing are also impaired. The ability to utter automatic speech, however, is often pre- characterized by difficulty with speech production but not with language served. Such speech includes greetings (“Hello”); short, common expressions (“Oh comprehension. It is related to damage my gosh!”); and swear words. in Broca’s area. In contrast to their difficulty with speech production, comprehension of language hemiplegia!Partial paralysis involving is relatively preserved in nonfluent aphasics. Because the primary and supplemen- one side of the body. tary motor cortex is close to Broca’s area (see Chapter 11), brain injuries that cause nonfluent aphasia often also cause hemiplegia —paralysis of one side of the body hemiparesis!Weakness of one side of the body. (usually the right side, which is controlled by the left hemisphere). Sometimes there is unilateral weakness, termed hemiparesis, rather than full paralysis. Seven years after a stroke, one person with nonfluent aphasia still spoke slowly, using mainly nouns and very few verbs or function words (a selective loss of action words, called averbia, sometimes occurs in nonfluent aphasia), and spoke only with great effort. When asked to repeat the phrase “Go ahead and do it if possible,” she could say only, “Go to do it,” with a pause after each word. Her pattern is typical of peo- Go to Media Clip 19.1 ple with extensive left anterior lesions. People with brain lesions as extensive as hers Broca's Aphasia tend to show little recovery of speech functions with the passage of time, but people bn9e.com/mc19.1 Damage in Broca’s area. 19.9 The Brain of “Tan”!Photo and MRI image of the preserved brain of M. Leborgne, who could only utter the syllable tan after his From N. F. Dronkers et al., 2007. 5 brain injury. Study of this brain and similar cases 3 led Paul Broca to identify a region in the anterior left hemisphere that is specialized for speech. Brain 130: 1432–1441 1 The damage in what is now known as Broca’s area is clearly evident in the photo (left) and in the horizontal MRI images (right) that correspond to levels 2 and 3 in the photo. Behavioral Neuroscience Behavioral Neuroscience 9E Behavioral Behavioral Neuroscience 9E 9E Breedlove Neuroscience 9E Breedlove Breedlove Breedlove Sinauer Associates Sinauer Associates Dragonfly Dragonfly Media Media Group Group L ANG UAGE AN D LATERAL IZ ATION ! 643 Sinauer Sinauer Associates Associates Dragonfly Dragonfly Media