Biology - Mod 5.pdf
Document Details
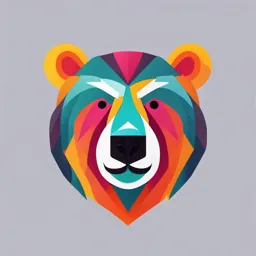
Uploaded by BeneficialSilicon
Tags
Full Transcript
Module 5: Heredity 5.1: Reproduction How does reproduction ensure the continuity of species? Reproduction ensures the continuity of species by ensuring the production of fertile offspring with genetic diversity for adaptability, through inheritance of genetic material. Organism → Survives...
Module 5: Heredity 5.1: Reproduction How does reproduction ensure the continuity of species? Reproduction ensures the continuity of species by ensuring the production of fertile offspring with genetic diversity for adaptability, through inheritance of genetic material. Organism → Survives → Growth → Reproduction → Inheritance of genes → Continuity [5.1.1] Mechanisms of Reproduction ASEXUAL AND SEXUAL REPRODUCTION Reproduction: The process of creating offspring, either sexually or asexually. ➔ Sexual reproduction: Reproduction which involves the combination of gametes, so that the offspring are genetically different to the parents. ➔ Asexual reproduction: Reproduction which does not involve the combination of gametes, so the offspring are identical to the parent. Advantages Disadvantages Sexual reproduction ✓ Variations in the population × Requires more time and lead to continuity of species energy to carry out ✓ There are more variations, × Requires a mating partner, so species are better able to can not be done individually adapt to changing selection pressures × Fewer offspring produced ✓ It is less likely that a selection pressure will remove all of the species Asexual reproduction ✓ Allows a species to rapidly × Lack of diversity between populate an environment species of a population. Variations, and therefore ✓ No requirement of mates adaptations, are limited ✓ Quick and not energy × Reduces ability to adapt to intensive, so it can be carried selection pressures out when the parent organism is under stress × May lead to extinction of the population, as one selection ✓ There is no requirement to pressure can wipe out all care for the offspring species, as there is no variation between them INTERNAL AND EXTERNAL FERTILISATION Fertilisation: The fusion of gametes in sexual reproduction. ➔ Internal fertilisation: Fertilisation where the combination of gametes occurs within the female body. ➔ External fertilisation:Fertilisation where the combination of gametes occurs in the external environment. INTERNAL ✓ Increased likelihood of × Fewer offspring are produced FERTILISATION fertilisation as the gametes are in over more time close proximity × Mating rituals are time ✓ Less gametes produced, consuming and postpone which reduces energy copulation expenditure × Higher risk of sexually ✓ Offspring have a greater transmitted diseases/ infections chance of survival as they are protected from the environment × Fertilisation, gestation and parental care demand energy from the parent × Breeding has to be paused for gestation (pregnancy) period EXTERNAL ✓A greater number of offspring × A large number of gametes FERTILISATION can be produced must be produced. This requires a lot of energy ✓ The behavioural process is simpler × Can only occur in aquatic environments ✓ Prevention of dehydration as it occurs in an aquatic environment × The gametes are more susceptible to the risks of the ✓ Reproduction can continue open environment without pause for gestation × External environmental ✓ Offspring are dispersed, conditions are unconstant, which meaning less competition may impact fertilisation ✓ Many gametes released, × Absence of parental care can increasing chance of fertilisation increase risk of predation and disease to offspring. ✓ No energy spent on parental care Comparison of internal and external fertilisation Characteristic Internal Both External Gametes Large number of Large number of both Released sperm, gametes Small number of eggs Fertilisation Occurs in female Sperm fertilises egg Occurs in external reproductive system aquatic environment Synchronisation Sperm is continuously Male and female of gamete produced, gametes released release Eggs are produced simultaneously over a cycle Chance of High Chance increases with Low fertilisation proximity of gametes Result of Zygote The development of a Zygote fertilisation (internal development) zygote requires a (external watery medium development) No. of offspring Small Both determined by: High (but survival rate is - No. of gametes (but survival rate is high) produced low) - Survival rate Breeding Low, Breeding frequency High frequency seasonal relies on environmental conditions Parental care Yes None - Care of zygote during development - Care of offspring after birth DEVELOPMENT Development: The growth of the zygote. When fertilised internally, development can be: ➔ Oviparous: External within an egg. ➔ Viviparous: Internal within the mother. ➔ Ovoviviparous: Internal within an egg within the mother. Unisexual reproductive ✓ Less energy required × Mating rituals required, which take system to maintain 1 set of time Separate male and female reproductive organs individuals with separate reproductive systems. Bisexual/ hermaphrodite ✓ Organism doesn’t need × A larger amount of energy is required reproductive system a partner of the opposite to maintain 2 sets of reproductive Organisms have both male sex to reproduce organs and female reproductive organs. ✓ Beneficial for organisms that are sedentary SEXUAL REPRODUCTION IN ANIMALS Gametes are produced by the parents by meiosis ↓ Each gamete is haploid (½ the total number of chromosomes) ↓ The sperm (male gamete) fertilises the oven/ egg (female gamete) ↓ The fusion of gametes results in a diploid zygote (has a complete set of chromosomes). The zygote has a unique combination of genetic material from both parents. Animal fertilisation can be internal or external. IN PLANTS Plants are bisexual. In angiosperms, the: MALE organs → Stamen (filament + anther) FEMALE organs → Pistil (stigma + style + ovary) Pollination Pollen (the male gamete) is produced in the stamen, and held up at the anther ↓ The pollen is removed from the anther by the wind or by insects. It is transferred to the pistil of the same flower(self-pollination) or another flower (cross-pollination) ⤷ [From the same plant or another one]. Fertilisation The pollen falls on the stigma, and a pollen tube grows through the stile to the ovary ↓ The pollen migrates through pollen tubes, to the ovules ↓ The pollen fertilises the ovules Development The fertilised ovule develops into a seed, containing the embryo ↓ The seed protects the embryo ↓ The fruit around it provides it with nutrients ↓ Germination When the fruit is removed, the seeds within it grow separate from the parent plant(s) ↓ The embryo lies dormant ↓ If on suitable soil, germination begins ↓ The embryo puts out its: ➔ Radical (first root) for absorption of water and nutrients ➔ Plumule (young stem) which develops leaves for photosynthesis ↓ The seed germinates into a seedling Methods of pollination METHOD ADAPTATIONS FOR METHOD Wind - Protruding stigma to catch more pollen - Light-weight, small pollen - Large pollen production - Flower is not colourful to conserve energy, as it doesn’t need to attract pollinators Animals - Colourful petals to attract pollinators ○ Insects ○ Birds - UV markings which insects are attracted to - Scent for attraction - Flower produces nectar to attract pollinators - Stigma and anthers within flower, energy not wasted in growing tall - Pollen is sticky, to attach to the pollinator and be dropped elsewhere Methods of seed dispersal METHOD ADAPTATIONS FOR METHOD Wind - Seeds are very light-weight Animals - The seeds are fruit bearing, meaning that it is eaten ○ Insects by animals ○ Birds - Once the fruit is eaten, the seed is either - Left to germinate - Passes through the digestive system, excreted and germinates Self-dispersal - The plant has an explosive mechanism, which propels the seed away from the mother plant - Propelling the seed to a different area reduces the competition for resources in one area, giving the offspring a greater chance of survival IN PROTISTS Haploid (n) protists Two haploid protist cells combine, and genetic material is combined in a new, fused nucleus. This produces a diploid zygote ↓ The zygote undergoes meiosis to produce 4 haploid daughter cells Diploid (2n) protists Adult cells undergo meiosis, producing 4 haploid gametes ↓ Gametes fuse during fertilisation to from a diploid zygote ↓ The diploid zygote will grow to maturity IN FUNGI Mitosis splits a diploid cell into haploid two daughter cells, which each contain a single set of chromosomes ↓ PLASMOLOGY: Two genetically different cells fuse together, combining their contents. This brings together two compatible haploid nuclei ↓ KARYOGAMY: The two haploid nuclei fuse into one diploid nucleus. This forms a zygote cell ↓ MEIOSIS: The zygote undergoes meiosis which produces haploid spores. These spores are distributed into the environment ASEXUAL REPRODUCTION IN PLANTS Vegetative propagation Method of asexual reproduction in plants, done by vegetative organs, which are modified parts of the plant. Perennating organs: Underground organs which store food. Modified part Vegetative organ Perrenating? Stem - Runners NO Underground stems that connect plants. - Rhizomes YES Horizontal stems that store nutrients. - Tubers NO Develop from swollen regions of a stem, or from buds/ eyes. - Bulbs YES Scales of bulb store food. New plants can grow from the bulb. Roots - Suckers NO Vertical growths that can resprout from underground. Apomixis (process) Generative tissue gives rise to plantlets asexually. Generative tissue includes: - Unfertilised ovules - Specialised leaf tissue. IN FUNGI Fragmentation Pieces of the fungal colony can break off and become separate colonies. Budding The nucleus of a fungal cell divides, and splits off from the rest of the cell by cytokinesis. Complete budding: Separation → new cell Incomplete budding: Bud stays attached to the mother → Keeps budding → hyphae → mycelium Spores Alternation of generation. Fungi can reproduce both sexually and asexually within one lifetime. 1. Haploid spores are generated from diploid sporophytes through meiosis 2. If the conditions are favorable, these spores will be released into the environment → Asexual reproduction 3. If the conditions are NOT favourable, the spores will form into haploid gametophytes a. Both male and female gametes are produced 4. Fusion of these gametes (fertilisation) produces zygotes, which are released into the environment → Sexual reproduction These zygotes can duplicate via mitosis, producing more sporophytes IN BACTERIA Binary fission A diploid (2n) mother cell splits into two diploid daughter cells which are genetically identical. A cell grows, reaching the limit of its SA:V ↓ The organism replicates its nucleoid and plasmid DNA (this ensures that each offspring retains a complete copy of the genome) ↓ The cytoplasm is pinched off at the area of cleavage ↓ A new cell wall is synthesised in this area IN PROTISTS Binary fission In the same way as bacteria, but without a cell wall. Budding Only a small group of amoeba called sarcodines can bud. A small outgrowth develops from the body of the parent sarcodine ↓ The offspring develop from this outgrowth and completely separates from the mother (no partial separation) [5.1.2] Fertilisation, Implantation, Pregnancy and Birth in Mammals CONTINUITY OF SPECIES Reproductive mechanisms ensure the success of producing offspring and nurturing them to reproductive maturity. This is so they can in turn reproduce and ensure the continuity of species through generations. Stage of development Continuity of species Internal fertilisation Ensures gamete fusion Implantation Protects embryo Pregnancy Provides embryo with nutrients Birth Occurs after the foetus is fully developed to ensure survival Lactation Sustains born offspring until it reaches reproductive maturity. SEX HORMONES Stages of sexual reproduction are regulated by hormones, ensuring reproductive success leading to the continuity of species. They are responsible for: - The development of reproductive organs - The development of secondary sexual characteristics In males In females ➔ Deepened voice ➔ Larger breasts ➔ Thicker body hair, and ➔ Widening hips more of it ➔ Growth of pubic hair ➔ Increased size & thickness of muscles and bones Types of sex hormones: ANDROGENS Main male hormone, but also present in females. The main androgen in males is called testosterone, produced by the testes. Androgen is responsible for the development of sperm, and secondary sexual characteristics in males. OESTROGEN Main female hormone, but also present in males. Involved with the development of the female reproductive system, and secondary sexual characteristics in females. PROGESTERONE Only in females. Ensures that the thickness of the endometrium is maintained during pregnancy. MENSTRUATION AND OVULATION Hormones involved Hormone Function Location produced 1. Follicle stimulating Maturation of follicles Pituitary gland (brain) hormone 2. Luteinising hormone Final maturation of follicles Development of corpus luteum 3. Oestrogen Development of sex organs Ovaries 4. Progesterone Controls pregnancy The process FSH rises, causing follicle cells to develop ↓ FSH boosts oestrogen production (positive feedback loop) ↓ Graafian follicle released during ovulation ↓ Oestrogen stimulates LH secretion ↓ LH inhibits FSH and oestrogen production ↓ LH causes dominant follicle to rupture and release egg ↓ Follicle develops into corpus luteum ↓ Corpus luteum produces progesterone and oestrogen ↓ LH and FSH production inhibited by progesterone. Prevents 2nd egg being released ↓ Endometrium thickens LH and FSH spike when follicle ruptures. Progesterone spikes when the corpus luteum is largest. Oestrogen spikes at both these times. If fertilised If NOT fertilised Zygote implanted into endometrium Corpus luteum degenerates ↓ ↓ Zygote produces Human Chorionic Gonadotropin Oestrogen and progesterone production stopped (hCG) ↓ ↓ Endometrium is shed (menstrual flow) hCG maintains corpus luteum to produce ↓ progesterone for 12 wks LH and FSH produced again ↓ ↓ The placenta develops fully and begins secreting Cycle repeats progesterone ↓ Corpus luteum degrades, the placenta maintains the pregnancy GAMETOGENESIS The formation of gametes from germinal epithelial cells. Spermatogenesis Germinal epithelium cells divide by mitosis (2n) ↓ mitosis They grow into primary spermatocyte (2n) ↓ meiosis I These split into 2 secondary spermatocytes (2n) ↓ meiosis II Each spermatocyte produces 2 spermatids (n) ↓ [Spermatids are provided nutrition by sertoli cells] ↓ differentiation Spermatids develop into spermatozoa (n) A total of 4 spermatozoa are produced in this process. Oogenesis Germinal epithelial cells divide by mitosis (2n) ↓ mitosis Grow into primary oocytes (2n) ↓ meiosis I (stop @ prophase I) Primary follicle formed (2n) ↓ Menstrual cycle starts @ puberty ↓ meiosis I Divides into secondary oocyte and a polar cell (n) ↓ meiosis II (mitosis) Secondary oocyte divides into an egg and another polar cell (n) ↓ An egg is formed and develops. The 3 polar cells are discarded FERTILISATION Gamete fusion and the production of a zygote The process Sperm are attracted to the egg by chemical signals ↓ Sperm pushes through the follicle layer around the egg ↓ The first sperm to break through binds to the zona pellucida ↓ Acrosome reaction triggered ↓ Acrosome bursts and digests the zona pellucida, allowing the sperm to enter the egg ↓ Cell membranes of the sperm and egg fuse ↓ Cortical reaction triggered ↓ Enzymes from the cortical granule are released, which harden the zona pellucida to stop more sperm entering ↓ The two nucleuses of the egg and the sperm undergo mitosis ↓ The entire cell divides, moving ½ of the egg’s chromosomes and ½ of the sperm’s chromosomes into separate cells ↓ Zygote created Breeding times SEASONAL BREEDERS CONTINUOUS BREEDERS The female animal is ready to mate at The female animal can mate at any time specific periods throughout the year during the year due to the continuous reproductive cycle Advantage: - The offspring are produced during Advantage: favourable environmental conditions - Offspring are continuously produced, ensuring population growth and continuity IMPLANTATION The embryo is embedded into the uterus. The placenta The foetus develops an umbilical cord and placenta. The placenta is a disk-shaped tissue which gets embedded into the uterus wall by placental villi. It connects the blood systems of the mother and the foetus without them fusing. Maternal → Foetus Foetus → Maternal - Oxygen - Carbon dioxide - Glucose - Urea - Water - Water - Minerals - Hormones - Antibodies - Hormones PREGNANCY Human development Zygote Morula Blastocyst Embryo Foetus Fertilised egg 16 unspecialised Mass of cells 8wks after Embryo develops cells - Inner forms fertilisation bone tissue. embro - Outer forms +8wks to birth placenta Hormonal control of pregnancy Embryos produce human chorionic gonadotropin (hCG). hCG prevents the corpus luteum from degrading, thus stimulating progesterone and maintaining a thickened endometrium. After 12 weeks, the placenta is fully developed. It takes over the production of progesterone and oestrogen, so the corpus luteum degrades. BIRTH When the offspring moves into the external environment from the mother’s reproductive system. Process of birth The cervix relaxes and widens ↓ amniotic sac bursts, releasing fluid ↓ baby comes out ↓ placenta is expelled (afterbirth) Hormonal control of birth Progesterone prevents contractions in order to maintain a stable environment for the growing foetus. In the last ⅓ of pregnancy, the placenta produces estriol. Estriol inhibits the production of progesterone, while promoting oxytocin production. Furthermore, the rise in oestrogen increases the number of oxytocin receptors in the endometrium. Oxytocin causes the uterine muscles to contract. Uterine contractions begin slowly, and increase due to positive feedback, becoming stronger until the baby is pushed out. LACTATION The production of milk to sustain the offspring after birth. Hormonal control of lactation The hormone prolactin stimulates the mammary glands to produce milk. ➔ High levels of oestrogen before birth stimulate the production of prolactin (positive feedback) ➔ High levels of prolactin inhibit milk production (negative feedback) Milk production will only begin with the decline of prolactin. This happens with the decline of oestrogen (ie. After the birth). [5.1.3] Manipulation of Plant and Animal Reproduction in Agriculture Scientific understanding of the mechanisms of reproduction allowed for the development of reproductive technologies. Reproductive technologies are any technologies which increase the rate of reproduction, ensure survival and thus continuity of species. Biotechnology is the manipulation of living organisms for human benefit. WHOLE-LEVEL MANIPULATION Selective breeding (plants & animals) Method: Humans breed together parents with desirable traits so that at least one of these traits appears in the offspring. Breeding can be: ➔ Intraspecific (between the same species) ➔ Interspecific (between different species) Advantages: Disadvantages: If there are desirable traits in the male Cannot guarantee the desired trait will be and the female, the offspring will most expressed likely also have at least one of these traits Reduces the genetic diversity of a population Offspring from interspecific breeding are sterile as their gametes are not viable, thus the trait can not be passed on further CELL-LEVEL MANIPULATION Artificial insemination (animals) Method: 1. Semen is collected from a male 2. A sexually receptive female is detected 3. Insemination performed by using an insemination gun to shoot semen into the female’s cervix Advantages: Disadvantages: Able to synchronise births Cannot guarantee the desired trait will be Ability to choose the mates increases expressed likelihood of passing down favourable Reduced genetic variation traits Ensurance of successful pregnancy increases yields Artificial pollination (plants) Method: 1. Pollen (male gamete) removed from the stamen of one plant 2. This pollen is applied to the stigma of another plant 3. Pollen fertilises the ovum Advantages: Disadvantages: Cross-breeding of favourable traits Cannot guarantee the desired trait will be Decreases likelihood of self-pollination expressed Ensures successful pollination of all Reduced genetic variation plants, increasing the yield Cloning (plants & animals) Method: 1. Somatic body cell of organism A is removed, and the nucleus is extracted 2. This nucleus is put into the denucleated egg of a donor 3. The egg is implanted into a surrogate mother 4. The offspring is a clone of A Advantages: Disadvantages: Cloned individuals have identical Clones have no adaptability requirements Expensive Ethical concerns The desired trait is guaranteed to be Clones have questionable health and life expressed expectancy GENE-LEVEL MANIPULATION Transgenesis (plants & animals) Method: 1. A gene for a specific trait is removed from a species 2. The transgene is held in recombinant plasmid 3. The gene is injected into the zygote of another species 4. The zygote + transgene is implanted into a surrogate mother 5. The offspring express the transgene in their phenotype Advantages: Disadvantages: Guaranteed expression of desired trait Transgenic organisms have low Increase yield adaptability Mixing of GM organisms with wild Reduces use of harmful chemicals populations Increases genetic diversity Trade issues with non-GMO countries ○ (as long as the transgenic Unknown side effects on humans organisms are not cloned) Transgenic organisms are usually cloned, decreases genetic variation IMPACT OF REPRODUCTIVE TECHNOLOGIES Initially, due to manipulation of reproduction, there are increased variations in a species. However, since the offspring with favourable traits are then cloned, variations decrease again. gene level species level ecosystem level ↓ variations → ↓ genetic diversity → ↓ species diversity → ↓ long-term biodiversity This limits the adaptability of a species to changing selection pressures, therefore limiting the continuity of species. 5.2: Cell Replication How important is it for genetic material to be replicated exactly? Genetic material refers to DNA. Because DNA replication produces genetically identical offspring, all members of a species have the same genome (collection of genes) but different variations of that gene (alleles). This means a species is defined by its genome. ➔ No two species have the same genome Therefore, exact DNA replication is important as it ensures that the correct genes are passed on from parent to offspring, so that the species can continue in the next generation. DNA replication → Inheritance of → Inheritance of → Continuity of species identical genes exact genome [5.2.1] The Processes Involved in Cell Replication THE CELL CYCLE G1 : Cell grows growth phase ↓ Volume increases faster than SA ↓ SA:V decreases ↓ S: DNA synthesis & replication synthesis ↓ phase Mutations ↓ G2 : Detection of mutations Growth ↓ phase 2 Correction of errors ↓ Nuclear Prophase, metaphase, division anaphase, telophase ↓ Cytoplasmic Cytokinesis division BEHAVIOUR OF CHROMOSOMES Before replication: Many genes → 1 DNA → 1 chromosome After replication: Many genes → 2 DNA → 1 chromosome DNA replicates, chromosomes don’t When 2 DNA are attached at the centromere, they are known as 2 sister chromatids. MITOSIS Location: Somatic (body) cells. Result: Diploid (2n) parent cell creates two diploid daughter cells. Function: Growth, repair, maintenance. INTERPHASE Chromosomes can not be seen individually, they are called chromatin material. ○ They are thin, long and coiled together. DNA replication takes place 46 chromosomes DNA replication 46 chromosomes → 46 DNA 92 DNA/ chromatids PROPHASE Nuclear membrane dissolves. Centriole divides and the halves move towards opposite poles of the cell. Individual chromosomes become visible. ○ Each is made of 2 chromatids due to DNA replication in interphase. METAPHASE Chromosomes align across the equator of the cell in a single file. Spindle fibers form which connect the centrioles to the centromeres of the chromosomes. ANAPHASE Spindle fibers contract. Chromosomes with one DNA are pulled to opposite poles of the cell. ○ They are no longer chromatids since the DNA molecules are not connected. TELOPHASE Nuclear division completes. Nuclear membrane forms around the chromosomes at each pole. 2 diploid, identical nuclei are formed. CYTOKINESIS Cytoplasm cleavages Cytoplasmic division 2 diploid, identical daughter cells are derived. MEIOSIS Location: Germline (sex) cells. Result: Diploid parent cell creates 4 haploid (n) daughter cells. Meiosis I: Reduction division. Creates haploid cells from diploid cells. Meiosis II: Maintains haploid number in daughter cells. Function: Gamete production for sexual reproduction. ↓ Ensures inheritance of the genome ↓ Source of variation ↓ Continuity of species INTERPHASE Chromosomes can not be seen individually, they are called chromatin material. ○ They are thin, long and coiled together. DNA replication takes place 46 chromosomes DNA replication 46 chromosomes → 46 DNA 92 DNA/ chromatids PROPHASE I Chromosomes with 2 chromatids appear. Chromosomes are arranged in homologous pairs. Nuclear membrane dissolves. Centrioles move towards the poles. Crossing over Non-sister chromatids cross over and exchange maternal and paternal genes across the chiasma. ○ Creates unique combinations of allele pairs on the same chromosome ○ This recombination of maternal and paternal genes causes variation Alleles are variations of the same gene. Alleles for a common trait are found on the same locus of a chromosome. If a segment does not cross over in the exact area, mutations occur. METAPHASE I Independent assortment Homologous pairs of chromosomes line up in double file along the cell's equator. The arrangement is random, and independent of other chromosomes. Random segregation Chromosomes of the homologous pairs move to the poles independently of each other. Create a unique combination of alleles in the gametes. Crossing over, independent assortment and random segregation are a source of genetic variation. ANAPHASE I Spindle fibers contract and pull chromosomes to the opposite poles. TELOPHASE I Nuclear division completes. 2 haploid, non-identical nuclei are formed. CYTOKINESIS I Cytoplasmic division completes. 2 haploid, non-identical cells are formed. Meiosis I completes. PROPHASE II N/A METAPHASE II Chromosomes line in a single file along the equator of the cell. ANAPHASE I Spindle fibers contract, pulling chromosomes to opposite poles. TELOPHASE II Nuclear division completes. 2 haploid non-identical nuclei are formed per daughter cell. CYTOKINESIS II Cytoplasmic division completes. 2 haploid, non-identical gametes are formed per daughter cell. In total, 4 haploid, non-identical gametes are formed. Mitosis vs. Meiosis MITOSIS MEIOSIS Type of cell produced Somatic Gamete No. of cells produced 2 4 Location Somatic cells Germline cells Function Growth and repair Gametes for sexual reproduction No. of chromosomes per Diploid Haploid daughter cell 2n → 2n 2n → n → n Degree of variation between Identical Large variation parent cell and daughter cells (Excluding variations due to (due to random segregation and mutations) crossing over of genes) DNA replication Yes, in interphase. Yes, in interphase. WATSON AND CRICK DNA MODEL History of the DNA model The W+C model of DNA was built in 1953. ➔ It was a double helix structure ➔ Scientists involved: James Watson, Francis Crick, Rosalind Franklin, Morris Wilkins Watson and Crick vs. Franklin and Wilkins Watson was a biologist Franklin was an x-ray Crick was a physicist crystallographer Perfect communication Wilkins produced DNA crystals Analytical skills Franklin found angles of diffraction using crystalised DNA to create a model Limited communication Nucleotide composition, pairing and bonding DNA and chromosomes have a 1:1 ratio since a chromosome is a molecule of DNA wrapped around histone proteins. Many genes → 1 DNA → 1 chromosome DNA is a 3D molecule made of a sequence of nucleotides. Unwrapped, it looks like this: Nitrogenous base + phosphate + sugar = Nucleotide The nitrogenous bases are: 1. Adenine (A) 2. Guanine (G) ⟶ Purines 3. Thymine (T) 4. Cytosine (C) ⟶ Pyrimidines They undergo the complementary base pairings: A=T [2 hydrogen bonds] G☰C [3 hydrogen bonds] Gene expression The process by which the genotype shows the phenotype is called gene expression. It happens through protein synthesis. GENE (DNA segment) ↓ Sequence of nucleotides/ nitrogenous bases ↓ Sequence of codons ↓(each codes for an amino acid) Sequence of amino acids ↓ Polypeptide chain ↓(one or more chains link) Specific protein ↓ Specific trait/ characteristic This is how each gene determines a trait. Many genes ⇒ many proteins ⇒ many traits DNA REPLICATION IN EUKARYOTES DNA replication occurs in the synthesis stage of interphase. DNA REPLICATION Importance Mitosis → DNA shared (same generation) ↗ DNA replication → identical DNA produced → cell replication ↘ Meiosis → DNA inherited (next generation) ↓ Passing genome on ↓ Recombination of genes ↓ Genetic variations ↓ Continuity of species The original strand 1 molecule of DNA has 2 complementary strands which go from 3’→5’ or 5’→3’. ➔ 3’ ⇒ ends in sugar ➔ 5’ ⇒ ends in phosphate The strands are antiparallel. The leading strand forms continuously from 5’→3’ towards the replication fork The lagging strand forms in fragments from 5’→3’ away from the replication fork The process of DNA replication INITIATION: Ligase relieves the strain on a twisted DNA helix ↓ Helicase uncoils and separates complementary strands ↓ ELONGATION ↙ ↘ Leading strand Lagging strand DNA polymerase (III) adds nucleotides RNA polymerase adds a RNA primer segments (primers) in the 5’ → 3’ towards to the strand, allowing DNA the replication fork. polymerase to bind. ↓ DNA polymerase also proofreads the strand DNA polymerase (III) adds to correct any base pair errors. Nucleotides next to the RNA ↓ primer in okazaki fragments. Ligase seals the gaps between ↓ DNA DNA polymerase (I) replaces The RNA primer with DNA and corrects any pairing errors. ↓ Ligase seals the gaps between DNA ↓ The RNA primer moves to the next area, and the cycle repeats until the entire lagging strand is formed. ↓ Strands recoil into a double-helix shape. 2 identical daughter strands produced. ↓ TERMINATION The 2 new DNA strands each contain 1 old template strand and 1 new strand. This is called semi-conservative replication. 5.3: DNA and Polypeptide Synthesis Why is polypeptide synthesis important? Polypeptide synthesis is important because it allows for gene expression to occur so that organisms can live. [5.3.1] DNA in Prokaryotes vs. Eukaryotes Prokaryotes Eukaryotes DNA shape Chromosomal DNA in tight Double helix coiled around histone supercoils around scaffold proteins. protein. - DNA wrapped around - For essential features histones to make nucleosomes Loop-like plasmid DNA. - For non-essential features Conservative replication Semi-conservative replication. DNA location Cytoplasm. Nucleus - Naked - Membrane bound NON-NUCLEAR DNA Some organelles (eg. Mitochondria, Chloroplasts) have their own DNA. Mitochondrial DNA (mtDNA) is identical through female lineage, and can be used to trace a genetic line. - Evolves more rapidly, as replication is not checked by repair enzymes - Abundant in every cell DNA vs. RNA DNA RNA Deoxyribonucleic acid Ribonucleic acid - Double helix - Single helix - Deoxyribose sugar - Ribose sugar - 4 bases - 4 bases - Adenine - Adenine - Thymine - Uracil - Guanine - Guanine - Cytosine - Cytosine - Stores genetic material - Converts the information stored in DNA - Undergoes replication into proteins - Doesn’t replicate mRNA Takes the coding sequence for specific proteins from DNA and transports to the ribosomes tRNA Carries amino acids to the ribosome. Specific types of amino acids correspond to the anticodon rRNA Form the ribosome. They move along the mRNA, and bind the tRNA [5.3.2] POLYPEPTIDE SYNTHESIS Function: To produce a polypeptide and then a protein Significance: Allows gene expression to occur Transcription RNA polymerase enzyme unzips a segment of the DNA helix (a gene) ↓ There are 2 strands: Non-coding and coding. To replicate the coding strand, mRNA forms against the non-coding strand. ↓ Pre-mRNA is created when free-floating nucleotides attach in complementary pairs. Instead of thymine, RNA uses uracil ↓ DNA rezips and never leaves the nucleus. Post-transcriptional modification The transcribed mRNA contains introns and exons. Exons ⇒ codes for amino acids Introns ⇒ non-coding regions Endonuclease enzymes cuts out the introns. The exons are connected by ligase enzymes to form mature mRNA. Translation A ribosome is composed of a large and small subunit, with 3 binding sites: E, P, A. Each codon (3 base pairs) of the mRNA has a complementary tRNA which carries an amino acid. Each codon codes for a specific amino acid. The order and number of amino acids determines the function of the protein. 1. 1. Initiation mRNA attaches to the small subunit. The starting codon has the sequence AUG ↓ A complementary initiator tRNA (carrying methionine) attaches to the A site and moves to the P site ↓ The tRNA of the next codon lands on the A site ↓ A polypeptide bond is created between the two amino acids ↓ tRNA drops it amino acid and moves to the E site as a free tRNA 2. Elongation The initiation tRNA is recycled in the cytoplasm ↓ The tRNA now carries 2 amino acids and moves to the P site ↓ The ribosome continues to read the mRNA ↓ tRNA lands on the A site, and a polypeptide bond is created between the amino acids of tRNA and ↓ tRNA drops its amino acid and moves to the E site, giving tRNA 3 amino acids ↓ This cycle continues as the polypeptide chain elongates 3. Termination The ribosome reaches a stop codon - UAG - UGA - UAA The stop codon doesn’t code for a protein, but it releases the polypeptide chain from the P site by releasing chemicals. MUTATIONS If a mutation occurs during DNA replication, when transcribed and translated it will lead to an incorrect amino acid in the polypeptide chain, creating an abnormal protein. This will impact the metabolism and thus the health of the organism. Base substitutions 1 base of the coding strand is replaced with an incorrect, non-complimentary one. This may: ➔ Cause no change → silent mutation ➔ Change 1 amino acid → missense mutation ➔ Shorten the chain → nonsense mutation Base insertions and deletions Bases are removed or added. ↓ This changes the reading frame, as bases are read in 3 (codons) ↓ Frame-shift mutation ↓ Significantly changes the amino acid sequence ↓ Non-functional protein ➔ GENES AND THE ENVIRONMENT ON PHENOTYPE Although the genotype is expressed as the phenotype, traits may be masked or changed by the environment. Genes + Environment = Phenotype The alteration may be temporary (usually) or permanent. Genes will only express traits if the environment is suitable. Examples: Having tall genes will not make an individual tall if there is not enough nutrients in the environment. The petals of hydrangea plants change colour based on soil pH acidic ⇒ blue alkaline ⇒ pink Siamese cats will grow dark colours on the tips of their bodies when the temperature decreases, and lose them when it increases. [5.3.3] Investigate the Structure and Function of Proteins in Living Things Polyribosomes Multiple ribosomes can form parts of the polypeptide chain, which come together to form the complete chain. Protein conformation One or more polypeptide chains can be structurally modified in the golgi apparatus to form specific proteins. Amino acids (monomers) → polypeptides → proteins (polymers) Types of proteins PROTEIN SHAPE FEATURES Primary protein - A single, linear chain of amino acids Secondary protein - A linear sheet of amino acids - Pleated into alpha helices or beta sheets Tertiary protein - A linear sheet of amino acids - Pleated into alpha helices or beta pleated - Folded into a 3D structure Quaternary protein - 2 or more proteins (of any structure) come together to form a single protein Once shaped, proteins are held together by hydrogen bonds. Each structure leads to the other, meaning that each protein will show characteristics of the shape before it. Types of proteins and functions TYPE OF PROTEIN FUNCTION OF PROTEIN Neurotransmitters Transport. [primary /secondary] Chemically transfer electrical impulses across the synapsis. Lysozymes Storage. [secondary] The fluid within the lysosome organelle. Enzymes Biocatalysts. [tertiary] Speed up biochemical reactions. Haemoglobin Transport. [quaternary] Transports oxygen Hormones Messengers. [quaternary] Chemical messengers which coordinate cell activity Antibodies Immunity. [quaternary] Flights pathogens for immunity Collagen Growth & repair. [quaternary] Muscle fiber which strengthens muscles 5.4: Genetic Variation How can the genetic similarities and differences within and between a species be compared? Genetic similarities and differences can be compared at a genetic level within species and at a species level between species. Within a population (intraspecific) : ➔ Similarities show common ancestry ➔ Differences show genetic diversity due to genetic variation Between populations (interspecific) : ➔ Similarities show evolutionary relationships ➔ Differences show species diversity due to species variation [5.4.1] Sources of Variation Genome: The complete set of genes in a species Gene pool: The complete set of alleles (gene variations) in a species. Genetic diversity: total of all the genetic characteristics in the genetic makeup of a species. Sources of genetic variation: Source Effect Crossing over Homologous chromosomes exchange genes, creating (prophase) new combinations of alleles in the chromosome. Independent assortment Homologous chromosomes arrange themselves and (metaphase I) separate independently, leading to random allele & combinations in the 4 non-identical gametes. Random segregation (anaphase I) Random fertilisation Non-identical male and female gametes fuse to create an offspring with a unique combination of alleles to form unique genotypes. Mutations Produce new alleles to increase the possible variations. Meiosis + Fertilisation + Mutations = recombination of genes ↓ Genetic variation ↓ Favourable adaptations ↓ Natural selection ↓ Reproductive maturity of fittest organisms ↓ Inheritance of favourable variations ↓ Genetic isolation ↓ Speciation (new species) ↓ Diversity Genetic diversity → variations in gene combinations Species diversity → variations of species in an area Biodiversity → variations of living things in an area ↓ Evolution Therefore, genetic variation leads to evolution. (Neo-Darwinism). [5.4.2] Mechanisms of Inheritance Chromosomes Autosome: contains genes responsible for body traits (skin, hair, eye colour) 1-22nd homologous pairs in humans. Sex-chromosomes: contains genes for gender and secondary sexual characteristics 23rd pair in humans - XX (females) - XY (males) Every body cell contains a complete set of homologous chromosome pairs. In humans, there are 23 pairs in total. MUTATIONS Mutations occur in both the somatic and germline cells. Mutations in somatic cells → expression in individual → no inheritance Mutations in germline cells → not expressed in individual → gametes → inheritance → expressed in offspring Gametes carry genetic variation, meaning that a trait will only be passed down if it occurs in the gametes. Inheritance only occurs in germline cells. AUTOSOMAL INHERITANCE Inheritance on germline cells where the genes inherited are on the 1st - 22nd pairs of chromosomes, and code for body characteristics. In a specimen, there is a gene for each trait, and 2 variations of this gene known as alleles. ➔ The alleles can be dominant or recessive. ➔ Dominant alleles are always shown over recessive ones. Eg. GT is the gene for height. T and t are the alleles (tall and short) T is dominant, t is recessive. P1: Parental generation The alleles of both parents Pure tall x Pure short TT x tt G1: Gametes Since only ½ of each parent’s genetic information is passed onto the offspring, they only have allele from each parent. F1: Filial generation 1 T T t Tt Tt t Tt Tt Offspring genotype: 100% heterozygous. Offspring phenotype: 100% tall. Simple dominance: One allele is completely dominant over the other. Codominance: Both alleles are dominant, so they both show without blending. Incomplete dominance: Both alleles are dominant, so they are blended in the phenotype. Multiple alleles: While there are only 2 alleles per genotype in an individual, some traits can be coded for more than 2 alleles. (Aka. Polygenic traits) If there are many variants, it is known as continuous distribution. If there are few variants, it is a discrete distribution. Example: Blood groups There are 3 alleles that code for blood types: A, B and O - O is recessive - A and B are both dominant over O, but equally dominant over each other Therefore, there are 4 blood groups for the genotypes created: IA → A IB → B i →O Genotype Alleles Phenotype IAIA IA Blood type A IAi IA and i IBIB IB Blood type B IBi IB and i ii i Blood type O IAIB IA and IB Blood type AB SEX LINKAGE Inheritance on germline cells where the genes inherited are on the 23rd pair of chromosomes. These genes code for gender and secondary sexual characteristics. Due to a mutation, an autosomal gene can be transferred to the X chromosome of the 23rd (sex) pair. ↓ It becomes an X-linked gene. ↓ It undergoes sex-linked inheritance XD > Xd > Y Female gametes → XX Male gametes → XY Never carried on the Y chromosomes Only carried on the X chromosomes X-chromosomes can be dominant or recessive, whereas Y chromosomes are always recessive. ➔ Therefore, a female can be a carrier ➔ Males can never be a carrier. If the allele for a sex-linked disease is recessive (e): XE = dominant f. allele Xe = recessive f. allele Y = m. allele (most dominant) (most recessive) Genotype Alleles Gender Phenotype XEXE XE Female Normal XeXe Xe Female Diseased XEXe XE Xe Female Carrier XEY XE Y Male Normal XeY Xe Y Male Diseased PEDIGREES Graphical representations of inheritance patterns of a trait in related individuals over generations. Patterns of inheritance Autosomal dominant ➔ Males and females affected Autosomal recessive ➔ Males and females affected X-linked dominant Male with trait: Female with trait: ➔ Passes onto all ➔ Passes to both daughters daughters and sons ➔ Passes onto no sons X-linked recessive Male with trait: Female with trait ➔ All daughters are ➔ All sons are affected carriers [5.4.3] Population Genetics The study of frequency of traits/ characteristics in a population. ↳ Genotype ↳ Phenotype ↳ Alleles Changes in frequency → Change to population → Genetic variation → Evolution Studying population genetics allows us to: Predict the survival of a species through genetic variation Conservation management Predict the inheritance of diseases and disorders in a population Trace evolution Mathematical model for frequency 𝑁𝑜 𝑜𝑓 𝑡ℎ𝑎𝑡 𝑎𝑙𝑙𝑒𝑙𝑒/ 𝑔𝑒𝑛𝑜𝑡𝑦𝑝𝑒/ 𝑝ℎ𝑒𝑛𝑜𝑡𝑦𝑝𝑒. 𝐹𝑟𝑒𝑞𝑢𝑒𝑛𝑐𝑦 𝑜𝑓 𝑠𝑝𝑒𝑐𝑖𝑓𝑖𝑐 𝑎𝑙𝑙𝑒𝑙𝑒/ 𝑔𝑒𝑛𝑜𝑡𝑦𝑝𝑒/ 𝑝ℎ𝑒𝑛𝑜𝑡𝑦𝑝𝑒 = 𝑇𝑜𝑡𝑎𝑙 𝑛𝑜. 𝑜𝑓 𝑎𝑙𝑙𝑒𝑙𝑒𝑠/ 𝑔𝑒𝑛𝑜𝑡𝑦𝑝𝑒𝑠/ 𝑝ℎ𝑒𝑛𝑜𝑡𝑦𝑝𝑒𝑠 Modelling populations Changes in a natural population can be identified when compared to an ideal population. ➔ Trends, patterns and limitations in the frequency data can be identified Population genetics: Shows how the gene pool changes over time. Genetic diversity: The total of all genetic characteristics in a species. Genetic variability: The tendency for genetic traits in a population to vary between individuals. Genome A collection of all genes responsible for all traits. The same for all members of a population. Gene pool Each gene has different variations or alleles. Collection of all the alleles in a population. Genotype Every individual inherits 2 alleles per trait. Each pair of alleles is a genotype. The genotype is different between members of a population. Phenotype Each genotype codes for 1 trait. The phenotypic expression of each genotype (or pair of alleles). SINGLE NUCLEOTIDE POLYMORPHISM (SNPs) When a nucleotide is replaced with an incorrect one during DNA replication, the alteration is called a single nucleotide polymorphism if it occurs in more than 1% of a population. Most SNPs occur in non-coding regions of DNA. If SNPs occur adjacent to each other on the same DNA molecule, they can be inherited together from a single parent. This group of SNPs is called a haplotype. Uses of SNPs Single nucleotide polymorphisms and short tandem repeats are genetic markers. They can be used as: ➔ Indicators of disease susceptibility ◆ Although not necessarily the cause of a disease ➔ Determine genetic relatedness ➔ Establish evolutionary relatedness 5.5: Inheritance Patterns in a Population Can population genetic patterns be predicted with any accuracy? Yes, using DNA analysis and genetic technology. [5.5.1] Technologies Used to Determine Inheritance Patterns The Human Genome Project Using DNA sequencing to find the order of nucleotides in every gene of the human genome. Polymerase chain reaction (PCR) DNA sequencing (Sanger method) Obtains a complete sequence of nucleotides for a specific gene or the whole genome. 1. DNA isolated from cell 2. Strands are separated using heat, so that terminator nucleotides can attach 3. PCR used to amplify DNA of different lengths a. Primer initiates beginning of replication b. DNA polymerase joins free-floating nucleotides c. The replication is stopped by tagged terminator nucleotides 4. The tagged nucleotides will eventually cover each base pair, creating replicated strands of differing lengths (inclusive of final base) 5. Gel electrophoresis is used to find the lengths (in base pairs) of the replicated strands 6. Laser beam used to identify the marker on each length of DNA 7. This shows the terminator nucleotide for each base pair 8. Thus, the order of the base pairs can be found by taking the complement of the terminator nucleotide DNA profiling Obtains band patterns using variable regions of DNA (STRs), which can be used to distinguish individuals. 1. DNA sample is extracted 2. DNA is amplified using PCR 3. Restriction endonuclease enzyme cuts out specific short tandem repeats (STRs) STRs are segments of DNA that are highly variable between individuals. 4. Segments undergo gel electrophoresis DNA placed into Electric current DNA travels based on Unique band gel wells passed size pattern created → → → The shorter the segment, the farther it travels 5. Radiolabelling probes are added 6. The band pattern is identified 7. Band patterns are unique to individuals (unique DNA profile) USES OF THE TECHNOLOGIES DNA sequencing Causes of genetic diseases Evolutionary relationships Cloning DNA profiling Disease testing A healthy person, a carrier and a diseased person will have different lengths of DNA sequences. This can be indicated based on how far the DNA moves in electrophoresis. This individual is most likely a carrier, since the bands match with the known carrier sample. Forensics The DNA in crime suspects is compared with DNA from a crime scene. If it is a 100% match, the suspect is guilty. The bands of suspect 2 completely match with that from the crime scene, therefore they are probably guilty. Paternity testing 50% of a child’s DNA comes from the mother and the father. If one parent is known, the other parent is the one which completes the child’s DNA sequence. The bands from male 1 cover the bands not covered by the known mother for the child. Therefore, he is most likely the father. [5.5.2] Uses of Population Genetics CONSERVATION MANAGEMENT Trends and patterns in population data allow us to: ➔ Develop strategies for management of endangered species ➔ Observe extinct species as a comparative model for similar endangered animals The Tasmanian Devil (endangered) Issues: - Virus-induced cheek cancer - Competition from introduced species - Habitat loss Management strategies: - Vaccines to treat virus - Raised in fenced habitats with low competition - Storage of complete genome to recreate species if they go extinct The Wooly Mammoth (extinct) Observation of DNA from fossils of 2 groups of wooly mammoths: 1. Mainland group 2. Island group When compared to the mainland group, the island group had mutations impacting - Their sense of smell - Their urine composition Which made it difficult for them to mark territory and mate. This was because there was less gene flow in the island population → less genetic variation. Thus, the island group lasted much shorter (4300 yrs) than the mainland group (45,000 yrs). Koalas (endangered) Tissue samples collected from koalas to identify genetic variation. Haplotypes studies to identify disease susceptibility. INHERITANCE OF DISEASE/ DISORDER Newborns are screened ↓ DNA sequenced ↓ SNPs or haplotype identified ↓ Large-scale database created for whole population ↓ Genomic studies ↓ Develops: - Preventative strategies - Treatments HUMAN EVOLUTION MULTIREGIONAL HYPOTHESIS REPLACEMENT (OUT-OF-AFRICA) HYPOTHESIS Process Process Homoerectus migrated out of Africa 1st group of ancient humans left Africa ↓ ↓ 2nd group evolved into ancestors of the modern Groups interbred but became reproductively human IN Africa isolated ↓ ↓ Group 2 outcompeted group 1 Different races emerged ↓ ↓ Group 2 spread into different areas and evolved Travel prevented complete speciation into the modern human ↓ Reproductive isolation led to the races Evidence Evidence Fossils of homosapiens show similarities Carbon dating of fossils suggests ancient to homoerectus and modern humans existed at the same time Suggests evolutionary relationship Common genes in mt-DNA suggest that they interbred Using mitochondrial DNA sequencing, we can: ➔ Establish evolutionary history of a species ➔ Establish evolutionary relatedness between species Evolutionary relatedness of two species can be determined by: DNA hybridisation % similarity of DNA sequences