Biochemistry 9 - Nucleic Acids PDF
Document Details
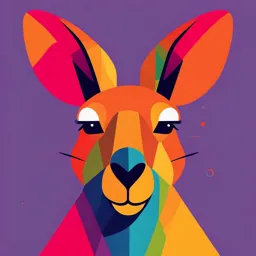
Uploaded by PopularRhodochrosite2288
Charles University
Tags
Related
- Analysis Of Nucleic Acids - PDF
- 2024 Chapter 2 Structure and Function of Nucleic Acids PDF
- Principles and Techniques of Biochemistry and Molecular Biology PDF
- Nucleic Acids - 2024/2025 Biochemistry I - University of Babylon PDF
- CHEM 43: Biochemistry I: Nucleic Acids PDF
- Nucleic Acids CHEM 43 Biochemistry I PDF
Summary
This document provides an overview of nucleic acids, including their structure, function, and different types like DNA and RNA. It also examines the key principles of nucleic acids, their role in cellular metabolism, and the various types of nucleotides.
Full Transcript
BIOMOLECULES Proteins Carbohydrates Lipids Nucleic acids Most of them are polymers (nucleic acids – DNA and RNA) – comprised of repeating units called Monomers (nucleotides) Such polymeric macromolecules in living systems are highly ordered chemical entities, with specific...
BIOMOLECULES Proteins Carbohydrates Lipids Nucleic acids Most of them are polymers (nucleic acids – DNA and RNA) – comprised of repeating units called Monomers (nucleotides) Such polymeric macromolecules in living systems are highly ordered chemical entities, with specific sequences of monomeric subunits giving rise to discrete structures and functions Three interrelated principles: (1) the unique structure of each macromolecule determines its function (2) noncovalent interactions play a critical role in the structure and thus the function of macromolecules (3) the monomeric subunits in polymeric macromolecules occur in specific sequences, representing a form of information on which the ordered living state depends Key principles Nucleic acids are both repositories and functional expression of biological information The transmission of biological information relies on molecular complementarity Biological information is subject to natural damage and change Nucleoside triphosphates occupy a central role in cellular metabolism, serving as an energy currency and as important regulatory signals Nucleic acids and nucleotides Nucleic acids are required for the storage and expression of genetic information There are two chemically distinct types of nucleic acids: deoxyribonucleic acid (DNA) and ribonucleic acid (RNA) Consist of chemically linked sequences of nucleotides Nucleic acids are biopolymers made of monomers – nucleotides The functions of DNA and RNA Only 2 known functions of DNA: Storage of biological information Transmission of that information to the next generation Gene – a segment of a DNA molecule that contains the information required for the synthesis of a functional biological product, whether protein or RNA Several classes of RNA Ribosomal RNAs (rRNAs) – components of ribosomes Messenger RNAs (mRNAs) – intermediates in protein synthesis Transfer RNAs (tRNAs) – adapter molecules that translate the information in mRNA into a specific amino acid sequence Noncoding RNAs (ncRNAs) – a wide variety of functions Small nuclear RNA, micro RNA.. Nucleotides Have a variety of roles in cellular metabolism Energy currency in metabolic transactions Essential chemical links in the response of cells to hormones and other extracellular stimuli Structural components of an array of enzyme cofactors and metabolic intermediates Constituents of nucleic acids deoxyribonucleic acid (DNA and ribonucleic acid (RNA) Nucleotides – basic structure Each nucleotide consists of a sugar bound on one side to a phosphate group and bound on the other side to a nitrogenous base Nucleotides – chemical structure 6-C sugars (hexoses): Nucleotides – (1) sugar base Glucose etc. There are two different kinds of sugars in a nucleotide, deoxyribose and ribose If the polynucleotide chain forms DNA then the sugars in its nucleotides are deoxyribose while nucleotides containing ribose as its sugar form RNA Pentose- 5-carbon sugar (for both ribose and deoxyribose) Numbering of sugars is “primed” to distinguish them from the numbered atoms of the nitrogenous bases Ribose and deoxyribose More stable, in DNA in RNA Conformation of ribose (a) In solution, the straight-chain (aldehyde) and ring (β-furanose) forms of free ribose are in equilibrium. RNA contains only the ring form, β-D-ribofuranose. Deoxyribose undergoes a similar interconversion in solution, but in DNA exists solely as β-2′-deoxy-D-ribofuranose. (b) Ribofuranose rings in nucleotides can exist in four different puckered conformations. In all cases, four of the five atoms are nearly in a single plane. The fifth atom (C-2′ or C-3′) is on either the same (endo) or the opposite (exo) side of the plane relative to the C-5′ atom Nucleotides – (2) phosphate group Polar molecule (highly ionized oxygen atoms) Same as in ATP (but in ATP, there are 3 phosphate groups) Carbon Hydrogen Nucleotides – (3) nitrogenous bases Oxygen Nitrogen A nitrogenous base is an organic molecule that contains the element nitrogen and acts as a base in chemical reactions The basic property derives from the lone electron pair on the nitrogen atom The nitrogen bases are also called nucleobases because they play a major role as building blocks of the nucleic acids deoxyribonucleic acid (DNA) and ribonucleic acid (RNA) Planar, aromatic, and heterocyclic Derived from purine or pyrimidine The numbering of bases is “unprimed” Two classes of nitrogen bases Called purines (double-ringed structures) and pyrimidines (single- ringed structures) The four bases in DNA's alphabet are: adenine (A) - a purine cytosine(C) - a pyrimidine guanine (G) - a purine thymine (T) - a pyrimidine In RNA, there is a uracil (U) – pyrimidine (instead of Thymine) Purines and pyrimidines 1. Purines are fused five- and six-membered rings Adenine A DNA RNA Guanine G DNA RNA 2. Pyrimidines are six-membered rings Cytosine C DNA RNA Thymine T DNA Uracil U RNA Structure - review Nucleoside – without the phosphate group! Bonding of the groups to the sugar – (1) Nitrogenous base Result from linking one of the sugars with a purine or pyrimidine base through an N-glycosidic linkage (covalent bonding!) Purines bond to the C1’ carbon of the sugar at their N9 atoms Pyrimidines bond to the C1’ carbon of the sugar at their N1 atoms glycosidic bond or link - between the anomeric carbon of a carbohydrate and another group or molecule! Bonding of the groups to the sugar – (2) phosphate group the phosphate group is esterified to the 5′ carbon of the sugar To review at home if interested in The base of a nucleotide is joined covalently (at N-1 of pyrimidines and N-9 of purines) in an N-β-glycosyl bond to the 1′ carbon of the pentose, and the phosphate is esterified to the 5′ carbon. The N-β-glycosyl bond is formed by removal of the elements of water (a hydroxyl group from the pentose and hydrogen from the base), as in O-glycosidic bond formation 5: N-glycosidic Bonds - Chemistry LibreTexts Esterification - Chemistry LibreTexts Nucleotides Nucleoside – without the phosphate group! Monomers for nucleic acid polymers Nucelotides result from linking one or more phosphates with a nucleoside onto the 5’ end of the sugar molecule through esterification Mono-, di- or triphosphates [!Phosphates can be bonded to both C3 (DNA and RNA sugar phosphate backbone) or C5 atoms of the sugar!] There are two main cellular functions for purines and pyrimidines: 1. the purines adenine and guanine and the pyrimidines cytosine, thymine and uracil are all utilized for the production of DNA and RNA 2. function of pyrimidines and purines is short-term energy storage Naming Conventions 1) Nucleosides: Purine nucleosides end in “-sine” - Adenosine, Guanosine Pyrimidine nucleosides end in “-dine” - Thymidine, Cytidine, Uridine 2) Nucleotides: Start with the nucleoside name from above and add “mono-”, “di-”, or “triphosphate” Adenosine Monophosphate, Cytidine Triphosphate, Deoxythymidine Diphosphate Nucleotides examples Nucleotides – Adenosine and guanosine derivates These compounds are a very powerful second messenger involved in passing signal transduction events from the cell surface to internal proteins The most common adenosine derivative is the cyclic form, 3'-5'-cyclic adenosine monophosphate, cAMP Regulate glycogen breakdown, lipids breakdown, stop cholesterol synthesis Regulate transcription and translation Regulate permeability of cell membrane Regulate insulin secretion Catecholamines biosynthesis Also, guanosine cyclic monophosphate (cGMP) Vasodilation Calcium homeostasis neurotrasmission Nucleotides - Adenosine derivates The most common form of energy in all cells is adenosine triphosphate, or ATP Release of the third phosphate to produce adenosine diphosphate, or ADP, is an extremely favourable reaction and can drive reactions requiring energy input Nucleotides – Other Derivatives Guanine triphosphate (GTP) and guanine diphosphate (GDP) are utilized by certain enzymes and receptors as an on/off switch while cytosine triphosphate and uridine triphosphate are both used in the production of biomolecules GTP- Protein synthesis, Purine Synthesis, ATP formation, gluconeogenesis A cyclic form of GMP (cGMP) is found in cells involved as a second messenger molecule. In many cases its' role is to antagonize the effects of c-AMP Important components of coenzymes such as FAD, NAD+ and Coenzyme A (flavin adenine dinucleotide is a redox -active coenzyme associated with various proteins, which is involved with several enzymatic reactions in metabolism; Nicotinamide adenine dinucleotide is a cofactor central to metabolism; CoA - an important molecule in controlling the interconversion of fats, proteins, and carbohydrates and their conversion into metabolic energy) Solubility of Nucleotides Hydrophobic and relatively insoluble in pH 7.0 water Leads to stacking interactions (van der Waals and dipole-dipole) Charged and more soluble at acidic or alkaline pH values Base-pairing – fulfil the requirement of keeping all hydrophobic stuff together – hydrophilic outside Hydrogen bonding pattern Hierarchical Levels of Nucleic Acid Structure Primary structure – covalent structure and nucleotide sequence Secondary structure – regular, stable structure taken up by some or all the nucleotides Tertiary structure – complex folding of large chromosomes or the elaborate folding of tRNA or rRNA structures DNA DNA, the repository of genetic information, is present in chromosomes in the nucleus of eukaryotic organisms, in mitochondria and the chloroplasts of plants Prokaryotic cells, which lack nuclei, have a single chromosome, but may also contain nonchromosomal DNA in the form of plasmids In eukaryotic cells, DNA is found associated with various types of proteins (known collectively as nucleoprotein) present in the nucleus whereas in prokaryotes, the protein–DNA complex is present in a non-membrane-bound region known as the nucleoid Structure of DNA DNA is a polymer of deoxyribonucleoside monophosphates covalently linked by 3′→5′– phosphodiester bonds With the exception of a few viruses that contain single-stranded (ss) DNA, DNA exists as a double-stranded (ds) molecule, in which the two strands wind around each other, forming a double helix Phosphodiester bonds join the 3′- hydroxyl group of the deoxypentose of one nucleotide to the 5′-hydroxyl group of the deoxypentose of an adjacent nucleotide through a phosphate group The resulting long, unbranched chain has polarity, with both a 5′- end (the end with the free phosphate) and a 3′-end (the end with the free hydroxyl) that are not attached to other nucleotides Formation of the phosphodiester bonds Phosphodiester linkages between nucleotides (in DNA or RNA) can be cleaved hydrolytically by chemicals, or hydrolyzed enzymatically by a family of nucleases: deoxyribonucleases for DNA and ribonucleases for RNA The bases located along the resulting deoxyribose–phosphate backbone are, by convention, always written in sequence from the 5′-end of the chain to the 3′-end In the DNA helix, the hydrophilic deoxyribose–phosphate backbone of each chain is on the outside of the molecule, whereas the hydrophobic bases are stacked inside The spatial relationship between the two strands in the helix creates a major (wide) groove and a minor (narrow) groove Step ladder model Watson-Crick Model for the Structure of DNA Offset pairing of the two strands creates a major groove and a minor groove 3 hydrogen bonds form between G and C 2 hydrogen bonds form between A and T The double helix is stabilised by: Metal cations (Mg2+) that shield the negative charges of backbone phosphates Base stacking interactions between successive base pairs Van der Waals Double helix In the double helix, the two chains are coiled around a common axis called the axis of symmetry The chains are paired in an antiparallel manner, that is, the 5′-end of one strand is paired with the 3′-end of the other strand The grooves provide access for the binding of regulatory proteins to their specific recognition sequences along the DNA chain Certain anticancer drugs, such as dactinomycin (actinomycin D), exert their cytotoxic effect by intercalating into the narrow groove of the DNA double helix, thus interfering with DNA and RNA synthesis Base pairing The bases of one strand of DNA are paired with the bases of the second strand, so that an adenine is always paired with a thymine and a cytosine is always paired with a guanine The base pairs are perpendicular to the axis of the helix The specific base pairing in DNA leads to the Chargaff Rule: In any sample of dsDNA, the amount of adenine equals the amount of thymine, the amount of guanine equals the amount of cytosine, and the total amount of purines equals the total amount of pyrimidines Bonding of base pairs The base pairs are held together by hydrogen bonds: two between A and T and three between G and C These hydrogen bonds, plus the hydrophobic interactions between the stacked bases, stabilize the structure of the double helix Separation of the two DNA strands in the double helix Helicase Enzyme unzips the DNA for replication The two strands of the double helix separate when hydrogen bonds between the paired bases are disrupted Disruption can occur in the laboratory if the pH of the DNA solution is altered so that the nucleotide bases ionize, or if the solution is heated Phosphodiester bonds are not broken by such treatment When DNA is heated, the temperature at which one-half of the helical structure is lost is defined as the melting temperature (Tm) Under appropriate conditions, complementary DNA strands can reform the double helix by the process called renaturation (or reannealing) Replication of DNA Step 1: pre-existing or parent strands become separated Step 2: each parent strand serves as a template for the biosynthesis of a complementary daughter strand DNA interaction with proteins DNA interacts with a large number of proteins, each of which performs a specific function in the ordered packaging of these long molecules of DNA Eukaryotic DNA is associated with tightly bound basic proteins, called histones These serve to order the DNA into basic structural units, called nucleosomes, that resemble beads on a string Histones and the formation of nucleosomes There are five classes of histones, designated H1, H2A, H2B, H3, and H4 These small proteins are positively charged at physiologic pH as a result of their high content of lysine and arginine. Because of their positive charge, they form ionic bonds with negatively charged DNA Histones, along with positively charged ions such as Mg+2, help neutralize the negatively charged DNA phosphate groups Two molecules each of H2A, H2B, H3, and H4 form the structural core of the individual nucleosome “beads.” Around this core, a segment of the DNA double helix is wound nearly twice, forming a negatively supertwisted helix Histones The N-terminal ends of these histones can be acetylated, methylated, or phosphorylated. These reversible modifications can influence how tightly the histones bind to the DNA, thereby affecting the expression of specific genes Neighbouring nucleosomes are joined by “linker” DNA approximately fifty base pairs long Histone H1, of which there are several related species, is not found in the nucleosome core, but instead binds to the linker DNA chain between the nucleosome beads Structure of RNA The genetic master plan of an organism is contained in the sequence of deoxyribonucleotides in its deoxyribonucleic acid (DNA) However, it is through the ribonucleic acid (RNA)—the “working copies” of the DNA—that the master plan is expressed The copying process, during which a DNA strand serves as a template for the synthesis of RNA, is called transcription Transcription produces messenger RNAs that are translated into sequences of amino acids (polypeptide chains or proteins), ribosomal RNAs, transfer RNAs, and additional small RNA molecules that perform specialized structural, catalytic, and regulatory functions and are not translated The final product of gene expression, therefore, can be RNA or protein, depending upon the gene Structure of RNA There are three major types of RNA that participate in the process of protein synthesis: ribosomal RNA (rRNA) transfer RNA (tRNA) messenger RNA (mRNA) Like DNA, these three types of RNA are unbranched polymeric molecules composed of nucleoside monophosphates joined together by phosphodiester bonds The three major types of RNA also differ from each other in size, function, and special structural modifications Nucleic acids – sugar phosphate backbone RNAs differ as a group from DNA in several ways: They are smaller than DNA They contain ribose instead of deoxyribose ribose They contain Uracil instead of thymine deoxyribose Most RNAs exist as single strands that are capable of folding into complex structures Ribosomal RNA rRNA make up about eighty percent of the total RNA in the cell rRNAs are found in association with several proteins as components of the ribosomes There are three distinct size species of rRNA (23S, 16S, and 5S) in prokaryotic cells In the eukaryotic cytosol, there are four rRNA species (28S, 18S, 5.8S, and 5S) Some rRNA function as catalysts in protein synthesis RNA with catalytic activity is termed a “ribozyme” Transfer RNA tRNAs are the smallest of the three major species of RNA molecules tRNA make up about fifteen percent of the total RNA in the cell There is at least one specific type of tRNA molecule for each of the twenty amino acids commonly found in proteins The tRNA molecules contain unusual bases (for example, dihydrouracil) and have extensive intrachain base- pairing that leads to characteristic secondary and tertiary structure Each tRNA serves as an “adaptor” molecule that carries its specific amino acid—covalently attached to its 3′-end— to the site of protein synthesis. There it recognizes the genetic code word on an mRNA, which specifies the addition of its amino acid to the growing peptide chain Messenger RNA mRNA comprises only about five percent of the RNA in the cell The most heterogeneous type of RNA in size and base sequence The mRNA carries genetic information from the nuclear DNA to the cytosol, where it is used as the template for protein synthesis If the mRNA carries information from more than one gene, it is said to be polycistronic (prokaryotes) If the mRNA carries information from just one gene, it is said to be monocistronic and is characteristic of eukaryotes In addition to the protein coding regions that can be translated, mRNA contains untranslated regions at its 5′- and 3′-ends Structural characteristics of mRNA Special structural characteristics of eukaryotic (but not prokaryotic) mRNA include: A long sequence of adenine nucleotides (a “poly-A tail”) on the 3′-end of the RNA chain, plus A“cap” on the 5′-end consisting of a molecule of 7-methylguanosine attached “backward” (5′→5′) through a triphosphate linkage The addition of this 7-methylguanosine “cap” permits the initiation of translation and helps stabilize the mRNA. Eukaryotic mRNA lacking the cap are not efficiently translated A chain of 40–200 adenine nucleotides attached to the 3′-end.This poly-A tail is not transcribed from the DNA, but is added after transcription. These tails help stabilize the mRNA and facilitate their exit from the nucleus. After the mRNA enters the cytosol, the poly-A tail is gradually shortened mRNA