Biochemistry - Electron Transport Chain Lecture Notes PDF
Document Details
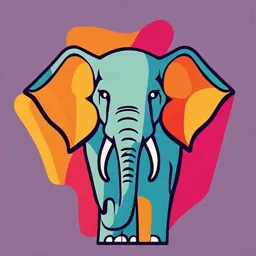
Uploaded by VeritableAzurite
Bluefield University
2023
Jim Mahaney, PhD
Tags
Summary
These lecture notes cover the electron transport chain (ETC) in biochemistry, including its role in respiration, components, and the generation of ATP. The notes include discussions of different tissue needs and energy metabolism.
Full Transcript
The Electron Transport Chain Lecture 28 Reference: Lieberman and Peet, Chapter 24 Bluefield University – VCOM Campus MABS Program Biochemistry Jim Mahaney, PhD https://www.youtube.com/watch?v=rdF3mnyS1p0 (Some small differences in detail from lecture) Lecture Objectives a. Compare the mitochondria...
The Electron Transport Chain Lecture 28 Reference: Lieberman and Peet, Chapter 24 Bluefield University – VCOM Campus MABS Program Biochemistry Jim Mahaney, PhD https://www.youtube.com/watch?v=rdF3mnyS1p0 (Some small differences in detail from lecture) Lecture Objectives a. Compare the mitochondrial content of different tissues and relate this characteristic to the function of the particular tissue (skeletal muscle versus cardiac muscle, parietal cells, etc.). b. Recall the two phases of respiration and relate the purpose of the electron transport chain (ETC) in the second phase of respiration. c. Identify the components of the ETC. Recognize which components are integral versus peripheral proteins and lipidbound molecules versus small metabolites. d. Relate the reaction of NADH (hydride transfer) at Complex I (NADH dehydrogenase) to start the electron transport chain reaction. e. Relate the reaction of FADH2 at Complex II to start the electron transport chain reaction. f. Recognize the physical location and role of Coenzyme Q in the electron transport chain. g. Relate how Coenzyme Q accepts and donates electrons in the electron transport chain and describe the oxidationreduction potential of its different Q ‘forms’ (i.e. ubiquinone, semiquinone, and ubiquinol). h. For each step of the ETC, identify how electrons move from one species to the next, and recognize which species becomes reduced and what becomes oxidized as the electrons move. i. Recognize and identify which ETC integral proteins pump protons across the inner mitochondrial membrane from the matrix to cytosolic side. j. Relate the change in oxidation-reduction potential as electrons flow through the electron transport change and recall how this relates to change in free energy. k. Recognize and interpret the influence of the proton gradient on the activity of the electron transport chain and vice versa. 2 Objective A Tissue Needs and Mitochondria Body tissues differ in how much energy they need and in the relative amount of mitochondria in these tissues: Cardiac and slow twitch muscle: lots of mitochondria, lots of oxygen consumption, lots of aerobic ATP production. steady, constant work. Fast twitch skeletal muscle: less mitochondria, more reliance on anaerobic ATP production by glycolysis, can utilize aerobic ATP production. Quick energy bursts, rapid ATP use then time for tissue recovery. Red Blood Cells: no mitochondria, no aerobic energy production, but energy needs are fairly light compared to other cell types. It all comes down to what is the metabolic need of each tissue. 3 Energy Metabolism: The Global Picture • The average adult human has a total energy fuel reserve of 166,000 kcal. • 1 lb of adipose tissue (15% water) = 3,500 kcal • 1 lb of muscle (85% water) = 365 kcal • This reserve consists of the following components: • Lipids ~85%, 9 kcal / g • Proteins ~15%, 4 kcal / g • Carbohydrates ~0.5%, 4 kcal /g 4 Skeletal Muscle and Energy Expenditure • Energy stores in a 70-kg lean human athlete (10% body fat) can be expressed in walking distance equivalents: ATP in the muscle at any given time: Phosphocreatine: Glycogen: Fat: 40 yds 120 yds (covered later: regenerates ATP from ADP) 1400 yds (anaerobically - Glycolysis…2 net ATP) 10 miles (aerobically - Glycolytic pyruvate à TCA) 630 miles (aerobic ATP production) • Clearly we need to generate energy on a continuous basis! • Can use fuel stores or dietary fuels, but fuel is needed • Consider other tissues: cardiac muscle, brain, peripheral tissues 5 Review: TCA Cycle Acetyl CoA + 3NAD+ + FAD + GDP + Pi + 2H2O 2CO2 + CoASH + 3NADH + 3H+ + FADH2 + GTP 3x 2.5 1x 1.5 9 ATP + 1 GTP 1 How do the electrons carried by FADH2 and NADH lead to ATP production? What do the Numbers 2.5 and 1.5 mean? 6 Objective B Generation of ATP From Fuels in the Blood Phase 1 of Respiration: Oxidation of Fuels • Glycolysis, fatty acid oxidation and the oxidation of amino acids produce acetyl CoA (and other TCA cycle intermediates) and reduced cofactors NADH and FADH2. • The TCA cycle oxidizes the acetate and produces more reduced cofactors (NADH, FADH2). Phase 2 of Respiration: ATP Production • The reduced cofactors give their electrons to the ETC. • The ETC uses the energy of the electrons to create a proton gradient across the inner mitochondrial membrane. H+ H+ H+ H+ • The ATP Synthase uses the energy of the proton gradient (ETC) to drive formation of ATP (oxidative phosphorylation). 7 The Chemiosmotic Theory § Electron transfer from NADH/FADH2 to O2 through the ETC creates a a proton concentration gradient, which serves as an energy source for ATP formation § Peter Mitchell, early 1960s, Nobel prize in 1978 8 The Electron Transport Chain (ETC) and Oxidative Phosphorylation (Ox Phos) • Electron transport chain (ETC) • a series of proteins and compounds that receive electrons from NADH and/or FADH2 generated by the TCA cycle and catabolic pathways • The ETC will transfer these electrons to molecular oxygen to form water. • The passage of electrons through the chain release energy that is used to transport protons across the inner mitochondrial membrane and thereby maintain a large proton gradient. • Oxidative Phosphorylation: • the process of synthesizing ATP from ADP + Pi using the energy of the proton gradient generated by the ETC. • The ETC system and its related ATP synthesis are tightly coupled and are some of the most complicated metabolic pathways encountered in Biochemistry. 9 The Mitochondrion and Oxidative Metabolism The outer mitochondrial membrane is permeable to anions and small molecules. Anything smaller than 10L molecular weight can pass. Contains porins: proteins in outer membrane that allow diffusion* of ions and water soluble metabolites < 10,000 molecular weight The inner mitochondrial membrane is impermeable to almost everything, including protons. Specific transporters are required for metabolites to pass The mitochondrial matrix is the site of: TCA cycle and fatty acid oxidation, both of which generate reduced coenzymes (NADH and FADH2). Electron transport chain (ETC) and the ATP Synthase, located on the inner surface of the inner mitochondrial membrane. • The ETC accepts electrons from NADH in the mitochondrial matrix and from FADH bound within flavin-containing proteins embedded in the inner mitochondrial membrane. • Electrons used to generate a proton gradient across inner mito membrane. • ATP Synthase uses the proton gradient to form ATP. 10 Objective C The Electron Transport Chain • Combination of integral membrane proteins, a peripheral protein and a lipid soluble cofactor. • Electrons enter the chain at Complex I from NADH and at Complex II from FADH2 • Electrons exit the chain at Complex IV by being transferred onto oxygen to form water • Electrons move *sequentially* through the chain in an energetically favorable direction. • Electron movement through the chain stimulates the *active* transport of protons (H+) across the membrane. • Proton gradient used as an energy source for ATP synthesis https://courses.lumenlearning.com/wm-biology1/chapter/reading-electron-transport-chain/ 11 Electron Transport and Oxidative Phosphorylation* Objectives C-I 3H+ ADP ATP H+ Pi H+ ADP ATP ATP/ADP Translocase and phosphate transporter 10 H+ are transported per 1 NADH oxidized, 6 H+ are transported per 1 FADH2 oxidized. 3 H+ are required per ATP synthesized, and 1 H+ is required for import of 1 inorganic phosphate * Complex II not shown here – will be covered on upcoming slides (required for the synthesis of ATP) 12 Pi Electron Transport and Oxidative Phosphorylation: NADH Objectives C-I Complex I: 4 H+ transported 3H+ per 2 electrons that pass through Complex III: 4 H+ transported per 2 electrons that pass through Complex IV: 2 H+ transported per 2 electrons that pass through. 10 H+ are transported out of the mitochondrial matrix per 1 NADH oxidized 3 H+ coming back into the matrix are required to synthesize 1 ATP 1 H+ coming back in is required for import of 1 inorganic phosphate 4 total H+ required for the synthesis of 1 ATP 13 Objectives C-I What About Complex II? Complex II is Succinate Dehydrogenase from the TCA Cycle • 4 4 2 Succinate + FAD à fumarate + FAD(H2) These electrons come from succinate (TCA cycle) and to directly to CoQ. Complex I is *not* involved Notice: • FADH2 electrons do NOT pass through Complex I FAD Succinate fumarate • Complex II does not transport H+ Complex III transports 4 H+ and Complex IV transports 2 H+ : 6 H+ total Recall 4 H+ required to synthesize 1 ATP: https://courses.lumenlearning.com/wm-biology1/chapter/reading-electron-transport-chain/ Therefore, for each FADH2 à 1.5 ATP formed 14 Objectives C-I Other FAD-Containing Proteins also Contribute • Complex II is Succinate Dehydrogenase from the TCA Cycle • Glycerol 3-phosphate dehydrogenase is the shuttle system used by glycolysis and other cytoplasmic NADH sources. • Fe-S (FAD) protein is part of the fatty acid oxidation pathway • In ALL CASES…only 6 H+ are transported per 2e- entering ETC from FADH2 sources… so only 1.5 ATP formed per FADH2 15 Objectives C-I One Pool of Coenzyme Q From Glycolysis lecture Coenzyme Q (CoQ) accepts electrons from Complex I and Complex II and other flavoproteins. Regardless of the source of e-, CoQ transfers electrons to Complex III. There is no proton pumping associated with Complex II or the other flavoprotiens, so * NO * direct contribution to the H+ gradient for ATP synthesis Better slide next slide… 16 Objectives C-I Put it ALL Together • Notice where the H+ transport occurs • This explains the number of ATP molecules produced starting from NADH (2.5) versus FADH2 (1.5) 17 Objective C COMPLEX I • NADH transfers a hydride ion (H: -) to Complex I • Complex I splits the electrons and gives the to FMN • FMN transfers 2 e-, one at a time, to a chain of Fe-S clusters • Final Fe-S transfers 2 e-, one at a time, to oxidized coenzyme Q to form fully reduced CoQ(H2) • Four H+ are transported out of matrix to the inter membrane space By Sunilpai93 - Own work, CC BY-SA 4.0, https://commons.wikimedia.org/w/index.php?curid=47270334 18 Objective C COMPLEX II : Succinate Dehydrogenase • Part of the TCA cycle and ETC: • succinate oxidized to fumarate by removing 2 e• Electrons transferred to FAD+ • FADH2 passes electrons to a chain of three Fe-S clusters • Electrons transferred on to CoQ forming CoQ(H2): electrons • No protons are transported out of the matrix during this process. Succinate Fumarate • Flavins are hydrophobic and must be bound to proteins. Not mobile in the aqueous phase. 19 Objective C and G Coenzyme Q • Lipid soluble cofactor, resides in the inner mitochondrial membrane • Freely diffuses between binding partners • Accepts 2 e- and 2 H+ in two single steps. Transfers electrons to complex III • Errors in this reaction can result in e- transfer to O2, forming superoxide, O2- (more on this in another lecture). 20 Heme A eFe3+ à Fe2+ Cytochromes are proteins containing a heme cofactor. There are a variety of hemes, which vary by structure and reduction potential, etc. 21 Objective C Complex III : Coenzyme Q – Cytochrome C Reductase • CoQ(H2) transfers electrons to Complex III • Very complex mechanism • Electron “bifurcation” • Protons taken up from matrix • CoQ(H2) protons are released into inter membrane space. • Complex III transfers 2e- to cytochrome c • Protons released into intramembrane space – not actually transported. But the net effect is the same. https://www.google.com/search?q=Complex+III&source=lnms&tbm=isch&sa=X&ved=0ahUKEwjvm9Hi69Pd AhWvxVkKHS6HBSIQ_AUIDigB&biw=1745&bih=857#imgdii=r4ytOSUZoliE9M:&imgrc=VjnIuqWCulqAWM: http://www.newworldencyclopedia.org/entry/Cytochrome_c 22 COMPLEX IV : Cytochrome c Oxidase Objective C Mitochondria use the majority of oxygen we breathe, and COMPLEX IV uses the majority of that O2 • Complex IV accepts 2e- from cyt c, one at a time. • e- transferred, one at a time, to O2, forming H2O. • O2 binds, is split into atomic oxygen, 2 H+ and 2 e- added to form H2O • Second oxygen atom held for next 2 e• Complex IV is called a “terminal oxidase” since the electron transfer ends here. • 2 H+ net are transported 23 Objective J ΔEo’ Drives the ETC • electrons flow spontaneously from a molecule with a more negative to a molecule with a more positive Eo’. • This favorable movement of electrons liberates the energy needed to pump H+ against the concentration gradient. • Energetically coupled process. • ΔEo’ = Eo’acceptor - Eo’donor: Recall that electrons spontaneously move from a o o species with a more negative E ’ to a species with a more positive E ’. 24 Objective K What Regulates the Electron Transport Chain? The Proton Gradient • The ETC uses electron passage as an energy source to actively transport protons (H+) out of the matrix. • The ETC will “sense” this growing voltage and slow down as the H+ gradient gets stronger. • As ATP is synthesized, the proton gradient gets smaller and the proton motive force decreases. • This decreases the voltage across the membrane and the ETC “senses” this • The ETC will respond by increasing its activity to re-generate a large proton gradient and proton motive force. • Likewise, as ATP synthesis slows, the proton gradient does not decrease a quickly, so the electron transport chain activity will decrease as well. 25 Objective K The BIG Picture • ETC creates the proton gradient • MUST have O2 • MUST have a supply of electrons (NADH, FADH2) • MUST have an intact inner mito membrane • The ATP Synthase (next lecture) uses the proton gradient as an energy source to synthesize ATP. • More ATP produced? Proton gradient decreases, ETC activated to rebuild gradient • Less ATP used? Proton gradient not used as fast so ETC slows down. H+ H+ • Both are critically sensitive to the magnitude of the proton gradient 26 Question A patient has stopped breathing and the supply of oxygen in his tissues is extremely low. How will this affect the function of his Electron Transport Chain? A. No effect: The ETC will continue as normal. B. The ETC will be activated to compensate for the loss of O2. C. The ETC will be inhibited due to a lack of O2. D. The ETC will be inhibited due to a loss of TCA cycle function. E. There will be additional loss of electrons from the ETC. 27 Question : Straightforward A patient is exposed to carbon monoxide, which destroys the function of Complex IV in his mitochondria. What effect will this have on the function of his TCA cycle ? A. No effect: CO binds to ETC Complex IV B. The TCA cycle will be activated to compensate C. The TCA cycle will be inhibited as the ETC shuts down D. The TCA cycle will no longer produce NADH for the ETC X 28