Electron Transport Chain & Oxidative Phosphorylation PDF
Document Details
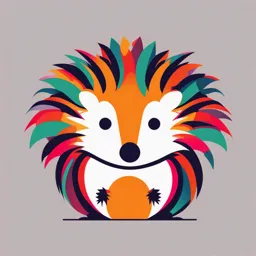
Uploaded by BullishPeridot
Midwestern University
Dr. Thomas Bodenstine
Tags
Summary
This document covers the electron transport chain and oxidative phosphorylation, explaining the process, the role of oxygen, and related clinical aspects. It provides enabling objectives about electron transport, oxidative phosphorylation, and clinical relevance. The summary highlights the critical role of mitochondria in energy production.
Full Transcript
Biochemistry 1501 Lecture 19 Dr. Thomas Bodenstine Electron Transport Chain and Oxidative Phosphorylation Introduction Electrons carried in NADH and FADH2 are removed (forming NAD+ and FAD) in a process known as the electron transport chain in the mitochondria. This process generates a proton [H+]...
Biochemistry 1501 Lecture 19 Dr. Thomas Bodenstine Electron Transport Chain and Oxidative Phosphorylation Introduction Electrons carried in NADH and FADH2 are removed (forming NAD+ and FAD) in a process known as the electron transport chain in the mitochondria. This process generates a proton [H+] gradient across the inner mitochondrial membrane by allowing for the flow of electrons. The proton gradient is used to power the synthesis of ATP from ADP and inorganic phosphate (Pi) through a complex known as ATP Synthase. This process is collectively referred to as oxidative phosphorylation. All of these pathways occur in association with the mitochondria, underscoring the importance of this organelle in aerobic respiration. Each round of the citric acid cycle generates mitochondrial NADH and FADH2 which are oxidized via the electron transport chain to NAD+ and FAD, respectively. This process, called electron transport, generates a proton gradient across the inner mitochondrial membrane. The final acceptor of reducing equivalents in the electron transport chain is oxygen. If oxygen is lacking then cells cannot carry out this process. The proton gradient is used to power the synthesis of ATP from ADP and inorganic phosphate, a process called oxidative phosphorylation. Cyanide and carbon monoxide, structurally similar to oxygen, are deadly in part because they disrupt oxidative metabolism. Uncoupling of electron transport from oxidative phosphorylation generates heat rather than ATP, and for humans this occurs primarily in infants. All of these pathways occur in association with some part of the mitochondria, underscoring the role of the mitochondria as “the powerhouse of the cell.” Terminal Objective: To understand and describe the electron transport chain and oxidative phosphorylation, and how these processes mediate energy production in the human body. Enabling Objectives: 1. Describe the process of electron transport, including the sequence and function of each electron carrier. 2. Understand the role of oxygen and how it functions as the final electron acceptor. 3. Describe the process of oxidative phosphorylation, proton motive force, and explain how oxidative metabolism has clinical relevance. 4. Explain how uncoupling proteins function to dissipate the proton gradient generated by the electron transport chain, and how this affects heat production and ATP yield. 5. Describe clinical aspects of mitochondria including how mitochondria are inherited and how this relates to mitochondrial disease. Recommended Reading: Lippincott’s Illustrated Reviews: Biochemistry, 8th Edition, Chapter 6, pages 80-88 Overview Reminder: ATP is the major energy currency of the cell. How have we made ATP so far? • • Substrate level phosphorylation through glycolysis. Direct production of ATP. Indirectly by substrate level phosphorylation through the citric acid cycle: production of GTP and then conversion to ATP. (Substrate level phosphorylation: production of ATP through ADP and a phosphorylated molecule; Lecture 4) At this point in our discussion of aerobic metabolism, the citric acid cycle has led to the production of NADH and FADH2. We will now discuss how the electrons from these reduced coenzymes lead to the production of ATP. A process known as oxidative phosphorylation in mitochondria by which our cells produce the majority of their ATP. 1. Electron Transport Chain and Oxidative Phosphorylation 1A) Mitochondrial NADH and FADH2 At this point, acetyl-CoA has been oxidized in a series of reactions that have indirectly generated ATP (from conversion of GTP), and also produced energy rich molecules NADH and FADH2. However, the ATP from NADH and FADH2 has not yet been produced. When NAD+ and FAD are reduced to NADH and FADH2, they carry additional electrons. These electrons are donated to a specialized set of electron carriers in the mitochondria, collectively known as the electron transport chain. These carriers participate in the exchange of electrons through donor/acceptor reactions. The flow of these electrons creates an energy “current”. 1B) Cytosolic NADH Recall that the process of glycolysis produces NADH in the cytosol. Under anaerobic conditions, the electrons being carried by this cytosolic NADH are utilized in the LDH reaction to produce lactate and NAD+ is regenerated in the process. What happens to cytosolic NADH under aerobic conditions? Two different shuttle systems can be used to transfer the electrons from cytosolic NADH into the mitochondria using a series of intermediates. The result is that the electrons are transferred and used to create either mitochondrial NADH or FADH2, depending on which shuttle is used. • Malate-aspartate shuttle: transfers cytosolic NADH electrons to produce mitochondrial NADH. • Glycerol-3-phosphate shuttle: transfers cytosolic NADH electrons to produce mitochondrial FADH2. In either case, cytosolic NADH is re-oxidized to NAD+ and electrons are transferred into the mitochondria. 2 1C) Organization and reactions of the electron transport chain The components of the electron transport chain are embedded or attached to the inner mitochondrial membrane. Four separate protein complexes (Complex I, II, III and IV) and two mobile electron carriers (Coenzyme Q and Cytochrome C) make up the electron transport chain. Members of the electron transport chain have increasing reduction potentials (strength of attracting electrons) that allows electrons to flow in a specific order from lowest to highest reduction potentials. Oxygen has the greatest reduction potential and is utilized at the end of the electron transport chain. Without oxygen as an anchor, this process becomes inhibited. Alternative names of each component are listed in parentheses for your reference: 1. Complex I (NADH dehydrogenase) • Complex I accepts electrons from NADH, causing NADH to be oxidized to NAD+ in the process. • Flow of electrons through Complex I causes it to pump 4 protons [H+] into the inter-membrane space. 2. Complex II (Succinate dehydrogenase [also used in the citric acid cycle]) • Accepts electrons from FADH2 (FADH2 is oxidized to FAD). • Complex I is bypassed. • NOTE: no protons are pumped by Complex II. 3. Coenzyme Q (Ubiquinone) • Mobile electron carrier that accepts electrons from Complex I and Complex II and transfers them to Complex III. The next 3 components contain iron in heme-like structures which is involved in the transfer of electrons. Thus, sufficient dietary iron is important for this part of the electron transport chain. 4. Complex III • Accepts electrons from Coenzyme Q • 4 protons [H+] are pumped into the inter-membrane space as electrons flow through Complex III • Complex III passes electrons to Cytochrome C 5. Cytochrome C • Accepts electrons from Complex III • Cytochrome C then transports the electrons to Complex IV 6. Complex IV (cytochrome C oxidase) • Accepts electrons from cytochrome C • 2 H+ are pumped into the inter-membrane space as electrons pass through Complex IV • Complex IV combines the electrons with oxygen (O2) to form water. NOTE: Cyanide (CN-) and carbon monoxide (CO) both inhibit Complex IV. 3 7. Oxygen is the final electron acceptor. After electrons flow through Complex IV, they combine with oxygen and protons to form water. This accounts for the majority of oxygen use by the human body. Without oxygen the electron transport chain backs up and energy cannot be produced, quickly creating an energy crisis for the body. Summary of electron flow from NADH Flow of electrons powers complexes I, III and IV to translocate protons to the intermembrane space + H + + +H H H + H + + +H + H Outer Mitochondrial Membrane H + H H Inter-membrane space C - e III Q I IV II - e (Complex II bypassed) NADH O2 + NAD Complex I accepts electrons (e ) from NADH Inner Mitochondrial Membrane H2O Electrons combine with O2 (the final electron acceptor) forming water Summary of electron flow from FADH2 Complex I not utilized, less protons pumped compared to NADH Outer Mitochondrial Membrane + H Complex I bypassed + + H + + H H + H H Inter-membrane space C Q I II III IV - e - e FADH2 O2 FAD Complex II accepts electrons (e ) from FADH 4 Inner Mitochondrial Membrane H2O 2. Oxidative Phosphorylation 2A) Proton motive force The transport of electrons down the electron transport chain is energetically favorable because of increasing reduction potentials. However, this flow of electrons does not directly result in ATP synthesis. Proton motive force As protons are pumped out of the mitochondrial matrix and into the intermembrane space (by complexes I, III and IV), this results in a pH gradient and a transmembrane electric potential. Higher [H+] concentration in the intermembrane space can be used to generate energy if the [H+] are allowed to flow back into the matrix down their concentration gradient. The energy from this flow is known as proton motive force and can be used to generate ATP from ADP and inorganic phosphate (P). A complex known as ATP-synthase (complex V) allows [H+] to flow from the intermembrane space back into the mitochondrial matrix. ATP is synthesized by ATPsynthase when protons flow back into the matrix by harnessing the energy from proton motive force. 2B) ATP-Synthase + H + H + H H + H + H + Two-part ATP-synthesizing complex with FO and F1 domains. + H + + +H H +H H + H FO Domain 1. FO Domain: when protons accumulate in the intermembrane space, ATPsynthase allows the protons to flow across the inner membrane back into the matrix. This causes rotation of the FO domain, which in turn causes rotation of the upper portion of the F1 domain. channel that allows flow of protons + H Stabilizer arm F1 Domain binds ADP and P, forms ATP ATP ADP + P 2. F1 Domain: binds ADP and phosphate (P). Rotation of the FO domain causes F1 to chemically convert bound ADP + P to ATP. 5 The proton gradient created by the electron transport chain powers ATP-synthase. A total of 3032 ATP can be generated through aerobic metabolism of glucose compared to anaerobic (net 2 ATP). Summarized below. Outer Mitochondrial Membrane + + H + + H H + H H+ + H H + H + +H + H H+ + H + H H + + + H H + H H C Q I III + + H +H + + H + HH +H + H +H H H + Proton gradient Proton motive force: protons flow through ATP+ H Synthase FO IV II ATPSynthase ATP ADP + P 2C) ATP/ADP and phosphate (P) transport ATP is transported to the cytosol through an ADP/ATP translocase in the inner mitochondrial membrane that pumps out ATP and imports ADP. ATP and ADP can diffuse through the outer mitochondrial membrane. A separate phosphate transporter in the inner mitochondrial membrane imports phosphate (P). P can diffuse through the outer mitochondrial membrane. This allows for the continual delivery of ATP to the cytosol while ADP and P are returned to the mitochondrial matrix to be reconverted to ATP. Summarized on next page 6 Cellular reactions Cytosol ATP, ADP and P diffuse through outer mitochondrial membrane Specific transporters mediate transfer of these molecules through the inner membrane ATP ADP + P Porin channels + ATP P ADP + H + H H H+ + H + H H + + + H + +H H + H+ +H+ + + +H H H H H H H H + +H + H + + H ATP P ADP ADP + P Mitochondrial Matrix ATPSynthase ATP 2D) Uncoupling Proteins When expressed, uncoupling proteins (UCPs) are located in the inner mitochondrial membrane and allow protons from the proton gradient to “leak” back into the mitochondrial matrix before they can be used by ATP-synthase to generate ATP. In this case, the energy from proton motive force is instead dissipated as heat, and therefore “uncouples” it from ATP production. This occurs largely in brown adipose tissue through the actions of UCP1. Thus, the purpose of brown adipose tissue (brown fat) is to produce heat. This is known as non-shivering thermogenesis. (NOTE: the role of the more abundant white adipose tissue in the human body is to function as a fuel reserve for fatty acids which will be discussed in upcoming lectures). Brown adipose tissue uses the majority of its proton gradient for thermogenesis and contains a high number of mitochondria to accomplish this. The high mitochondrial content is what gives this tissue its brown appearance. This thermogenesis is mediated in mammals by UCP1 and plays a major role in heat production, especially during hibernation. The activity of UCP1 is fueled by fatty acids stored in the brown adipose tissue which facilitate the transfer of [H+] back into the matrix. 7 Humans have very little brown adipose tissue except during the newborn phase where brown adipose tissue exists in neck, upper chest and upper back which allows the neonate to respond to fluctuations in temperature. Other UCP family members are present in humans but their biologic roles remain unclear. 2E) Life with oxygen The use of oxygen in human metabolism allows us to produce maximum ATP yields from metabolic fuels. Approximately 90% of oxygen is devoted to the electron transport chain/oxidative phosphorylation. However, when electrons are combined with oxygen, this can also produce reactive oxygen species (ROS) such as superoxide and hydrogen peroxide. Normal functioning of the electron transport chain and oxidative phosphorylation results in low level ROS production. Our cells have pathways to neutralize ROS but in some conditions these oxygen species can contribute to disease (e.g. Alzheimer’s disease) and promote neurocognitive decline. Pollution and toxins can increase ROS in our cells. Some evidence has shown that diets high in antioxidants can help to combat these negative effects. 3. Defects in Mitochondrial ATP Production As we have seen above, mitochondria serve as the location for the citric acid cycle, electron transport chain and oxidative phosphorylation. Thus, as discussed, aerobic respiration is responsible for the majority of ATP production in a cell, and since this occurs in mitochondria, these organelles are the primary site of ATP production. 3A) Mitochondria function and inheritance The number of mitochondria present within a cell will vary based on the cell type and its function. Unique organelles Mitochondria divide and contain their own DNA which closely resembles bacterial genomes. Evidence suggest that mitochondria developed from a symbiotic relationship between a eukaryotic cell and an intracellular photosynthetic bacterium. The eukaryotic cell provided protection to the bacteria and the bacteria produced ATP for the eukaryotic cell. Genome The mitochondrial genome contains 37 genes • 13 of these genes encode for proteins (mostly components of the electron transport chain and ATP-synthase) • The remainder code for tRNAs and rRNAs. • Other proteins utilized in mitochondria are encoded by the nuclear genome and imported into mitochondria. IMPORTANT: unlike nuclear DNA, mitochondria do not contain DNA repair pathways. This makes mitochondrial DNA more susceptible to mutations. 8 Inheritance Mitochondria are inherited in a maternal pattern since mitochondria from the sperm do not enter the egg during fertilization. Mutations in nuclear encoded mitochondrial proteins will exhibit a more classical Mendelian inheritance pattern (e.g. recessive, dominant). However, if mutations exist within mitochondrial DNA, the inheritance pattern can be variable because mitochondria are randomly distributed within eggs. Example: Mother with 50% mutated mitochondria (mild/no disease) may have a child with 80% mutated mitochondria (severe disease) or 20% mutated mitochondria (no symptoms) due to random assortment of mitochondria during egg development. 3B) Mitochondrial disease When mutations occur within mitochondrial pathways that affect processes such as oxidative phosphorylation, this may lead to significant functional consequences due to the lack of ATP. ATP ATP ATP ATP ATP ATP ATP ATP Reduced energy output ATP ATP ATP ATP ATP ATP ATP Mitochondrial disease Healthy mitochondria Defects that reduce the activity of mitochondrial aerobic metabolism will significantly decrease ATP production. Tissues and organs which need high levels of ATP are the most affected. These include neurons, especially during early stages of development, cardiac cells and muscle tissue. Thus, mitochondrial disease often presents with neurologic and/or neuromuscular complications due to dysfunction of muscle as a result of reduced ATP production and a lack of neurostimulation which can lead to muscular atrophy. 3C) Clinical features • Exertional fatigue: the lack of proper ATP production causes difficulty regarding physical exertion in patients with mitochondrial myopathies. ATP stores are depleted quickly and cannot be maintained during exercise. • Lactic acidosis: because aerobic metabolism is impaired, a higher dependence on anaerobic metabolism (conversion of pyruvate to lactate instead of acetyl-CoA) becomes necessary. Since anaerobic metabolism of glucose produces significantly less net ATP per molecule of glucose (2 ATP compared to 30-32 ATP), higher activity of this pathway is needed to compensate for the loss of ATP production and leads to high levels of lactate from anaerobic metabolism. High activity of anaerobic metabolism causes acidification of blood and is associated with elevated lactate levels in the blood. As the pH of the blood decreases, this has effects on the function of organs and must be quickly corrected. 9 • Visual complications: A common feature of these abnormalities involves a weakening of the muscles which control eye movements, an effect known as ophthalmoplegia causing patients to turn their head to look at objects rather than their eyes. A gradual drooping of the eyelids known as ptosis is common in these patients. The muscles which control the eyelids contain a high density of mitochondria. This allows for large-scale production of ATP making them resistant to fatigue and allowing the eyelids to stay open. When ATP production is altered in mitochondrial disease, the eyelids remain partially closed due to the lack of ATP needed to keep them open. Examples of mitochondrial disorders: Brain 129 (Pt 9):2363-74 • • • • • • Kearns-Sayre syndrome Leigh disease Leber hereditary optic neuropathy Mitochondrial encephalomyopathy, lactic acidosis and stroke-like episodes (MELAS) Myoclonic epilepsy with ragged-red fibers (MERRF) Neuropathy, ataxia and retinitis pigmentosa (NARP) Patients are taught “energy management”, helping them to understand their individual energy capabilities and avoid muscle/tissue damage as a result of activity or exertion. Maximizing activity for each patient helps to prevent deconditioning and maintenance of existing muscular function. 10 Study Questions 1. Which of the following directly accepts electrons from FADH2 in the electron transport chain? A) Complex I B) Complex II C) Complex IV D) Cytochrome C 2. The energy provided by proton motive force in the mitochondria is used by which of the following? A) Glycerol-3-phosphate shuttle B) Citrate synthase C) ADP/ATP translocase D) ATP-synthase 2. A 19-year-old woman is diagnosed with a mitochondrial disorder. During therapy for her condition, the patient exhibits exertional fatigue and muscle pain with exercise. At the biochemical level, what is the reason for these symptoms? A) Increased electron transport chain activity. B) Inhibition of oxidative phosphorylation. C) High levels of ATP production by ATP-synthase. D) Reduced anaerobic metabolism through LDH. Answers: 1B, 2D, 3B 11