Oxidative Phosphorylation Biochemistry PDF
Document Details
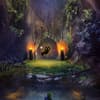
Uploaded by iiScholar
Arizona State University
Tags
Summary
This document details the process of oxidative phosphorylation, focusing on the proton motive force and the ATP synthase mechanism. It explains how the electron transport chain creates a proton gradient across the mitochondrial membrane, driving ATP production. The document also discusses different components and mechanisms related to the process.
Full Transcript
# Oxidative Phosphorylation ## Introduction The electron transport chain produces a higher proton concentration (and a lower pH) outside the mitochondrial matrix than within it, or in other words, a pH gradient across the membrane. The separation of charge and the difference in concentration serve...
# Oxidative Phosphorylation ## Introduction The electron transport chain produces a higher proton concentration (and a lower pH) outside the mitochondrial matrix than within it, or in other words, a pH gradient across the membrane. The separation of charge and the difference in concentration serve as a source of stored energy referred to as the proton motive force. The stored energy is released when the excess protons in the intermembrane space are allowed to flow down their concentration gradient back into the mitochondrial matrix. The enzyme complex called ATP synthase couples this flow of protons with the synthesis of ATP through condensation of ADP with inorganic phosphate (Pi). Because this mode of ATP synthesis is powered by oxidation of NADH and FADH2, it is called oxidative phosphorylation. This lesson explores the proton motive force and how it facilitates oxidative phosphorylation. ## 12.3.01 The Proton Motive Force Lesson 12.2 discusses the four complexes of the electron transport chain that together result in the pumping of protons from the mitochondrial matrix into the intermembrane space. Proton pumping results in a pH difference across the inner mitochondrial membrane, with the intermembrane space having a lower pH (ie, a higher H+ concentration) than the mitochondrial matrix. Specifically, in a typical healthy mammalian cell, the intermembrane space pH is approximately 7.4 while the pH in the mitochondrial matrix is close to 7.8. Because protons have a positive charge, the difference in proton concentration across the membrane also yields a voltage across the membrane, with the intermembrane space being more positively charged than the mitochondrial matrix. The resulting electrochemical gradient stores potential energy, called the proton motive force (pmf). Note that the term "force" in this case is a misnomer because the pmf does not refer to the acceleration of mass directly, but rather to the potential energy in the gradient and the work done as protons move along it. The energy stored by the pmf is released by allowing the protons to cross the membrane back into the mitochondrial matrix (ie, by allowing protons to flow down their electrochemical gradient). However, as discussed in Lesson 3.3, ions cannot readily cross the hydrophobic environment of a phospholipid bilayer such as the inner mitochondrial membrane. To facilitate reentry into the matrix, a channel or carrier protein is needed. The enzyme ATP synthase facilitates diffusion (passive transport) of protons into the matrix. Active transport of protons out of the matrix through the ETC, and passive transport back into the matrix, are shown in Figure 12.29. ## 12.3.02 ATP Synthase ### ATP Synthase Mechanism ATP synthase, sometimes called Complex V of the electron transport chain, is a translocase enzyme (Concept 4.2.08). Many translocases couple the energy released by ATP hydrolysis with the transport of solutes against their electrochemical gradient (eg, the Na+/K+ pump). ATP synthase, however, facilitates the reverse process: it couples transport of solutes (H+) down their gradient with synthesis of ATP. Proteins that couple solute transport to chemical reactions are said to be chemiosmotic, and therefore ATP synthase facilitates ATP production by chemiosmosis. Figure 12.30 highlights several components of ATP synthase. These components do not need to be memorized, but an understanding of their interactions greatly facilitates understanding of the underlying mechanisms of ATP synthase. ### ATP Synthase Components ATP synthase consists of two major domains called the F<sub>o</sub> and F<sub>1</sub> domains. Each domain contains multiple subunits. The F<sub>o</sub> domain is a transmembrane complex through which protons can flow. The protons bind a set of subunits called the c subunits. Each c subunit can bind one proton, and every time this occurs, the set of subunits rotates. Once a subunit makes a complete rotation, it releases its proton into the mitochondrial matrix and is then ready to bind another proton. The F<sub>o</sub> domain also contains a homodimeric arm consisting of two identical subunits called the b subunits. This arm extends from the membrane into the mitochondrial matrix, where it interacts with the F<sub>1</sub> domain. The F<sub>1</sub> domain contains three sets of heterodimers, each consisting of an a subunit and a ß subunit. The aß dimers surround the y subunit, which is connected to the c subunits of F<sub>o</sub>. As the c subunits rotate, so does the y subunit. This links proton movement to y subunit rotation. Rotation of the y subunit causes the αβ complexes to change conformations. In other words, at any given time, each aß complex is in a conformation different from the other two aß complexes. The rotation of the y subunit is shown in Figure 12.31. ### ATP Synthase Rotation and ATP Production One aß complex is in a conformation that readily binds ADP and P; (Dimer 1 in the first panel of Figure 12.31), another is in a conformation that binds ATP (Dimer 2), and a third is in a low-affinity conformation that binds neither (Dimer 3). As the y unit rotates, the aß complex that was bound to ADP and P; (Dimer 1) changes to the ATP-binding conformation. In so doing, the ADP and P₁ molecules are brought closely together, which catalyzes their conversion to ATP. Simultaneously, the complex that was originally bound to ATP (Dimer 2) changes to the low-affinity conformation that binds neither substrate, and the ATP that was bound to it is released. Finally, the complex that was unbound (Dimer 3) changes to the conformation that binds ADP and P₁ and binds them. This cycle continues as long as protons continue to flow through the F<sub>o</sub> domain. ### ATP Yield Per Glucose The most common model of human ATP synthase proposes that three protons flowing through F<sub>o</sub>cause rotation of the y subunit from one aß complex to the next; therefore, nine protons are required for one complete rotation of the y unit, which produces three ATP molecules. Once ATP is produced in the mitochondrial matrix, it must be transported to the cytosol. This is accomplished through an antiport system in which ATP exits the matrix while ADP enters. ATP can then freely diffuse from the intermembrane space into the cytosol for use as needed. To phosphorylate the ADP that has entered the matrix, inorganic phosphate must also enter. This occurs through a symport system in which a proton moves from the intermembrane space into the matrix through the phosphate transporter. Therefore, although this proton does not move through ATP synthase, a fourth proton (in addition to the three needed to cause rotation) must enter the matrix to synthesize cytosolic ATP. Consequently, according to this model, producing ATP for use in the cytosol requires a total of four protons moving into the matrix per ATP synthesized (see Figure 12.32). ## 12.3.03 Uncoupling of ATP Synthase Because ATP synthase relies on the proton gradient generated by the electron transport chain, ATP synthase function is said to be coupled to the ETC. However, it is possible to uncouple these processes. In isolated mitochondria, for example, addition of the molecule 2,4-dinitrophenol (2,4-DNP) or of the molecule FCCP allows protons to cross the membrane without passing through ATP synthase. Figure 12.33 shows a simplified mechanism of FCCP-mediated uncoupling. 2,4-DNP uncoupling follows a similar mechanism. When these uncouplers are present, the electron transport chain continues to function as long as it has fuel (eg, pyruvate, acetyl-CoA, succinate). Therefore, oxygen consumption continues and may even increase because the smaller pH gradient makes it easier for protons to be pumped. However, ATP production slows because many of the pumped protons return to the matrix without providing energy to ATP synthase. Many endothermic (ie, warm-blooded) organisms deliberately uncouple proton flow from ATP synthesis, at least partially, to help maintain body temperature. Certain cells (eg, brown adipose tissue cells) express an H+ channel protein called thermogenin or uncoupling protein 1 (UCP1) in their inner mitochondrial membranes. In cold conditions, these channels are induced to open and allow proton flow into the matrix as shown in Figure 12.34. When protons flow through ATP synthase, the released energy is coupled to ATP synthesis. In other words, the energy of the proton gradient is used to do work. In contrast, when protons flow through uncouplers such as thermogenin, the energy is not used to do work and therefore is converted to heat instead. This heat may then be used to help maintain a high body temperature in cold external conditions.