Biotechnology: Past, Present, & Future PDF
Document Details
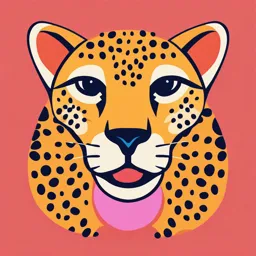
Uploaded by OrderlySphinx
Tags
Summary
This document provides an overview of biotechnology, covering its historical context, various applications, impact on food production and medicine, and the different types of biotechnology (first, second, and third generations). It discusses topics like genetically modified organisms (GMOs), traditional breeding, and ethical considerations around using biotechnology to improve humans. It also touches on specific examples within the field, such as the use of biotechnology to produce drugs.
Full Transcript
Biotechnology : What we already have done using biotechnology : Purple tomatoes contain high levels of anthocyanins which are healthy. Have been shown to decrease cancer levels in mice. Sterile mosquitoes can help reduce populations and spread of malaria and dengue fever Floo...
Biotechnology : What we already have done using biotechnology : Purple tomatoes contain high levels of anthocyanins which are healthy. Have been shown to decrease cancer levels in mice. Sterile mosquitoes can help reduce populations and spread of malaria and dengue fever Flood an area with sterile mosquitoes Salmon containing increased growth hormone allow more efficient farming Genetically modified salmon Animals expressing a gene encoding a fluorescent protein are used in medical research Expressing a green florescent protein There are still things that cannot be done using GM technology. Ex. Can’t make dinosaurs, can’t make dragons What we might be able to do (but should we?) Although research can be used to improve humans there are enormous ethical issues around doing so. Would it be beneficial to engineer people to run as fast as pro athletes ? We can do it with biotech What is biotechnology ? Terms : Bios – means “life" Technikos – means “tools" Logos – means “the study of" Using tools to study and manipulate living organisms Biotechnology therefore is… The characterization, use and/or change of organisms, using some technical process, to the advantage of mankind and the environment. (NB: it does not only refer to molecular biology and genetic manipulation) A field that tries to bring together biology, chemistry and engineering According to the European Federation of Biotechnologists (EFB): "Biotechnology is the integration of natural and engineering sciences in order to achieve the application of organisms, cells, parts thereof and molecular analogues for products and services." THE HISTORY OF BIOTECHNOLOGY Biotech is nothing new Started with dog domestication 1970s : microbes and animals were manipulated to create drugs like insulin (from dead animals) 1980s : true insulin human gene for insulin was taken and put into E coli that basically makes insulin Tomatoes : first genetically Modified plants Animals are used to produce Drugs ( ex. Put drugs into an animal and milk the animal) WHY DO WE NEED BIOTECHNOLOGY? - The graph shows how the population will increase over the next 30 years - Increases in population : we need more food and better medicine - Biotech will increase food production and the types of medicines we have - As the population increases Arable land per capita (ha in use per person). Data from FAO - The increase in world population is accompanied by the decrease in land we can grow on - This graph shows the amount of arable land per capita that is used to produce food - Since the population has been growing wd have increased food in the past Why do we need biotechnology : - This graph show that we have managed to increase yield - This graph : The amount of land that is used to produce a fixed quantity of crop - We are able to feed everybody because we can produce more and more food on the available land - Most of this has been accomplished using traditional breeding - How humans have altered crops - Over the past 9000 years we have managed to change corn from the plant on the left to the plant on the right - This has been done by manipulating 6 Genes to alter this plant - Trough natural selection - This is caused by a natural mutation somewhere in the genome - Over time this has led to artificial corn - There are big breeding companies that utilize DNA in order to identify specific plants that have things like high yield ect. Why we need biotechnology It has been estimated that conventional plant breeding will not be enough to increase yields for this growing population - We have a problem : we have consistently be able to increase plant yield - The rate of increase has started to decrease over the past decade - The reason is when plant breeding started in the industrial scale - Plant breeders started with a wide variety of different plants that they have isolated a=from all over the world - They started teasing out different genes and introducing them to what is known as elite varieties - Elite varieties are grown to produce the food we eat - We are running out in finding genes that can be used to increase yield - The dotted line is how much we need to increase for the expected world population - There is a gap into what we need and what we are doing - It can be done by using gmos Impact of biotech : Since the late 1970s when gmo plants has been grown Reduction in pesticide use Increase in yield Caused by decreases in insect attack Big increase in farmer income Why we need biotechnology : - Not only will we have more people, they will also live longer, so there is a need for better medicines etc - We have an increasing population which is getting older - The graph shows life expectancy to increase - Because of our aging population we need better medicine to cure disease that come with age - Biotech is fundamental into developing drugs that help cure these different diseases RELEVANCE OF BIOTECHNOLOGY - Amish Farmers Grow Nicotine Free Biotech Tobacco and Blight Resistant Potatoes - Amish farmers in Pennsylvania say they can earn twice as much with biotech tobacco. - Genetically modified plants Fuel for thought - The biofuels revolution is on its way – and South Africa is well placed to harvest its bounty. - Just one problem: will it eat up all our food? - Or increase food prices - We need to produce less fossil fuels thus use of biofuels - Sugar cane, canola Developing new drugs : - HIV Vaccine Research is "Best Hope" for Controlling AIDS Pandemic - Production of vaccines always use biotech RELEVANCE OF BIOTECHNOLOGY : - Biotech used to produce stem cells - These are cells which can be used to produce any organ in the body - DNA tech is used to identify people - Evidence for criminals - Identifying plants - Legal context : patent law Types of biotechnology can be defined as 1. Different generations 2. Different colors 1. BIOTECHNOLOGY GENERATIONS A. Conventional use, cultivation and improvement of organisms - Natural plant products - Breeding / improvement - Aspects of modern agriculture (e.g. hydroponics) grow in liquid - Fermentation (traditional brewing, bread baking etc.) - Nothing to do with genetic modification B. The use of in vitro technology - Plant tissue culture - Embryo rescue (plants) and in vitro fertilization (animals) like plants that don’t produce seeds - Growing things in Bioreactors (bakers yeast, bits of a plant or animal) - Growing an organism in a sterile culture - Plants in sterile cultures C. Molecular plant biotechnology - Molecular markers - Cloning and stem cell research (alter DNA) - Genetic engineering( making a genetically modified organism) - DNA technology FIRST GENERATION: BREEDING - Breeding/selection is an age-old practice through which genetic variation is selectively utilized. - Breeding is one of the most important aspects - Humans have been doing it for 1000s of years Example : Forms of Brassica oleracea developed from wild cabbage. (a) Kales - leaf and stem (b) Kohlrabi - enlarged stem (c) Broccoli - floral buds (d) Cauliflower - floral meristem (e) Brussel sprouts - lateral buds (f) Cabbage - apical buds. - bred to produce different things - Caused by small changes within the genome First Generation Biotechnology Green revolution Refers to the exponential increases in the yields of crops due to dedicated breeding and selection programs and improved agricultural practices, e.g. maize production increased from 1.5 tons/ha in 1930 to 6.2 tons/ha in 1980. - first generation biotech is incredible in Increasing crop yield - Green revolution led to a huge increase in the production of plants - Caused by industrial breeding - Introduction of DNA markers - Norman Borlaug – Father of the green revolution - Awarded Nobel peace prize 1970 in recognition of his contributions to world peace through increasing food supply FIRST GENERATION: NATURAL PLANT PRODUCTS - D - First generation biotech takes many forms - Farmer grows ginger corns (1st photo) - Extract ginger compounds like (3d photo) - You make more and more money - The farmer grows more ginger - The supermarket extracts more ginger FIRST GENERATION: FERMENTATION - Beer brewing - Wine making - Bread making - Utilization of bakers yeast - Alcohol and bread - Important for industries around the world SECOND GENERATION: IN VITRO TECHNOLOGY What is it? In vitro = in glass. - Includes all techniques in which plants/tissue goes through a sterile, in vitro culturing phase. - Forestry industry and potato industry (they put them in cultures) - Helps to maintain seedless fruit cultures How is it used? - How are trees propagated in the forestry industry? How do you make new crosses with seedless grape cultivars? - Protoplasts are plant cells where all the cell walls are away - Right side : there is a little plant growing in media that contains all the nutrients that the plant needs - If you cut the plant and leave the piece to grow it will grow into another plant THIRD GENERATION: MOLECULAR MARKERS What is it? - A molecular marker is a molecule which can be linked directly to a specific genetic trait. - Biotech that utilizes DNA - Identifying individuals - Using it to identify individuals for forensics How are they used? - The eventual phenotype of a plant can be predicted by analyzing it on DNA-level. THIRD GENERATION: GENETIC MANIPULATION What is it? - The direct (non-sexual) addition of genetic material to an organism. - Production of genetic modified organisms - Adding a new piece of DNA into an organism How is it used? - New genetic traits can be added to an organism or unwanted traits can be removed. - Removing genes that lead to a genetic disease 2. The Colors of Biotechnology - Red: Health, Medical, Diagnostics - Yellow: Food biotechnology, Nutrition Science - Blue: Aquaculture, Coastal and Marine Biotechnology - Green: Agriculture, Environmental Biotechnology - Brown: Arid zone and Desert Biotechnology - Dark: Bioterrorism, Biowarfare, Bio crimes - Purple: Patents, Publications, Inventions, IPR’s - White: Gene based bioindustries - Gold: Bioinformatics, Nanobiotechnology - Grey: Classical fermentation and Bioprocess Technology GMO’s occur naturally - Sweet potatoes contain genes inserted using the same bacteria that humans use to make GMOS’s - Some butterflies contain genes injected into them by parasitic wasps. - Looking at sequence in different genes - No deaths from GMOs - GMO plants are safe for consumption Third generation biotechnology is a very new discipline (Avery transformed bacteria for the first time in 1944, the first plants were only transformed in 1987, but already it has had a huge influence worldwide. The first GM crops were released in 1996, by 2005 (10 years) there were already nearly 100m ha planted in more than 21 countries worldwide Four main GM crops (soybean, maize, cotton and canola), but others emerging (e.g. potato). Two main transgenes, roundup resistance and Bt (Bacillus thuringiensis) toxin. RECOMBINANT DNA-TECHNOLOGY The central dogma : Central dogma definition : is the process by which the instructions in DNA are converted into a functional product. - The flow of info within a biological cell - DNA contains all of the information which will lead to the production of protein - DNA sits in the nucleus - It can be duplicated - And transcribed into mRNA - mRNA will leave the nucleus and go out into the cytosol and will be translated into the production of proteins - proteins are the enzymes etc that actually do things in cells - if we want to alter a cell we need to alter the protein, because the protein does stuff - what we do : - we alter the protein - we take the DNA that codes for the protein and put that into the nucleus of a living organism - and that’s why we want to make transgenic organisms - so we can add a new piece of DNA - which will make new RNA - which will end up making protein in the cell THE CENTRAL DOGMA AND THE GENETIC CODE Definitions : Transcription = process where RNA is made using DNA as a template RNA polymerase Replication = process where identical DNA molecules are made DNA polymerase - DNA is a double stranded nucleic acid that contains four bases a) Adenine – A b) Cytosine – C c) Guanine – G d) Thymine – T - Remember : A with T and G with C - These 4 bases will go trough a specific sequence - Where each 3 bases will code for one amino acid – called a codon - Top strand : amino acids and proteins - Bottom strand : mirror image of top strand - These 3 different bases called codons code for different amino acids - At the RNA you get transcription of the top strand of DNA into RNA - Differences between RNA and DNA - 1) RNA is one strand - 2) instead of thymine RNA contains uracil -U - RNA goes out into cytosol - Used by ribosomes to synthesize proteins - Methylamine(met) – encoded by AUG - This is an nm codon because it is the start codon - The first amino acid present in protein - Then at the end you get a stop codon that tell the ribosomes to stop making protein at this point - This means that DNA will contain several dozen codons - The enzyme used in this process Different types of enzymes : - DNA Polymerase : this allow DNA to replicate during cell division - RNA polymerase : The enzyme that takes the DNA and manufacturers RNA Codons in DNA - Multiple codons encode - Different amino acids So, how can we manipulate DNA? - One way to do it is to use restriction enzymes - Each of these enzymes cuts a specific sequence of DNA - These sequences are different for each enzyme DNA is cut like this by a specific restriction enzyme : USING RESTRICTION ENZYMES, DNA LIGASE AND PLASMIDS : ❖ Bacterial restriction enzymes cut DNA molecules at specific DNA sequences called restriction sites ❖ A restriction enzyme usually makes many cuts, yielding restriction fragments ❖ The most useful restriction enzymes cut DNA in a staggered way, producing fragments with “sticky ends” that bond with complementary sticky ends of other fragments ❖ Bacterial restriction enzymes cut DNA molecules at specific DNA sequences called restriction sites ❖ A restriction enzyme usually makes many cuts, yielding restriction fragments ❖ The most useful restriction enzymes cut DNA in a staggered way, producing fragments with “sticky ends” that bond with complementary sticky ends of other fragments ❖ DNA ligase is an enzyme that seals the bonds between restriction fragments ❖ Each restriction site is between 4 to 8 bases ❖ If you have a large amount of DNA a restriction enzyme will cut at multiple points in that DNA ❖ Each restriction enzyme will yield a really specific sequence of what is called restriction cuts ❖ However we want to get hold of a piece of DNA and manipulate it ❖ We can cut DNA with restriction enzymes ❖ But we can ligate it back together by using an enzyme called DNA ligase ❖ In this case we have an example where we digest with 2 different enzymes ❖ They cut different sequences ❖ The difference between these is the kinds of DNA ends they produce ❖ Because EcoRI cut apart from each other it produces sticky ends ❖ This is useful because we can easily pair these together ❖ They will naturally line up EcoRV cuts in the middle and produces a blunt end ❖ This has the disadvantage that they don’t naturally line up ❖ You can glue together any piece of DNA from any enzyme that produces a blunt end What is a plasmid? - a Small, circular piece of DNA that can replicate independently of the chromosome - Expression of the inserted gene will be regulated by the promoter - Generally we want to make copies of a strand of DNA we made - The way we do this is to grow the DNA in a host : E coli - So the first thing we have to put it in a piece of DNA that will replicate in E coli and allow the production of lots of bits of DNA - We do this using plasmids - A plasmid is a circular bit of DNA that can go into a host and it can then grow and make multiple copies of itself - Selectable maker : identification - Antibiotic resistance marker : identify e coli which contain the plasmid - Origin of replication : allows plasmid to be replicated in bacteria - Promoter : allow dry transcription - Inserted gene : both sides are restriction sites – thus we can cut the plasmid without the inserted gene and cut it with the restriction enzyme we could take a piece of DNA combine them together and add some DNA ligase which will add a little piece of DNA into the plasmid DIFFERENT COMPONENTS OF PLASMIDS : Plasmid Component Description DNA sequence where plasmid replication Origin of Replication (ORI) starts Allows selection of bacteria that carry the Antibiotic Resistance Gene plasmid Short DNA sequence containing many Multiple Cloning Site (MCS) unique restriction enzyme cutting sites to allow. where we insert pieces of DNA Piece of DNA that has been ligated into Insert the plasmid, normally in the MCS Drives transcription of the insert DNA, leading to the production of recombinant protein. Promoters can lead to Promoter Region constitutive expression, expression at certain growth phases, or be induced by chemicals Allows selection of an organism that contains that price of DNA. In bacteria it Selectable Marker would normally be and antibiotic resistance gene (as above). In plants it could also be a herbicide resistance gene. Short piece of DNA where synthesised DNA primers can bind, either to allow Primer Bindings Sites sequencing of the DNA or to amplify by the polymerase chain reaction. More realistic view of plasmid Left antibiotic resistance lacZ important gene - We would take a plasmid - We would cut it with ecol1 - Which would be joined tog- ether in a circle which is joined by a sticky end Restriction enzyme cuts sugar-phosphate backbones. - We do this in a plasmid - We can insert a piece of DNA into a site using the enzyme DNA ligase DNA CLONING: - - Represents i.o.w. the multiplication of a DNA fragment (in a plasmid) by using bacteria. - Plasmids are used to increase pieces of dna we want to get multiple copies of - Top left : we have a plasmid that’s growing in the bacteria E coli - We could isolate this plasmid - Cut it with a restriction enzyme - We could then take a chromosome of any organism - Cut that chromosome up – this will cut the chromosome up into millions of pieces of dna each with a eco1 site - We can then ligate pieces of dna from that chromosome into the restriction site of the plasmid we cut with eco1 and then we can take that plasmid which has a new piece of dna - Then we take that plasmid with a new piece of dna and put it into a bacteria - The bacteria will start off with o e cell containing that plasmid - But we can take E coli. That contain that plasmid and inoculate it into liquid media and allow it to grow overnight - Range from litres to millilitres - Overnight : you find a thick culture with lots of cells containing the new dna - We can spin down that liquid culture we can use chemicals to isolate the plasmid dna - Which means we end up with a larger amount of new dna than what we started with WHERE DOES THE DNA COME FROM? A number of ways, mostly from: 1. Polymerase Chain Reaction (PCR) - Only works if you know what the sequence of the DNA is. - If you work with an organism where you know the genome sequence 2. Libraries - Can be used to identify genes based on DNA similarity to other genes - You don’t know the genome sequence IN VITRO AMPLIFICATION OF DNA: The Components and Process.... Hybridization is very specific between complementary sequences: 5’-ATTGCGTACTAGCATGA-3’ 3’-TAACGCATGATCGTACT-5’ So, specific sequences can be identified using primers. EXPLANATION OF THE RIGHTHAND SIDE PHOTO AND POLYMERASE CHAIN REACTIONS : The polymerase chain reaction is a very important molecular biotechnology technique. It allows the extremely rapid amplification of very specific pieces of DNA, but only if you know the sequence of that DNA. So you need to know what the sequence is – either through genome sequence or through somebody else, identifying a particular gene. Now, it takes normally a few hours to start from a very small amount of DNA and use the PCR reaction to increase it to produce a large amount of DNA of a particular sequence that you're interested in. Now, the PCR reaction, if you look on the left, you'll see a tube and it contains lots of different things. It contains DNA, and it's the DNA that contains the gene that you're interested in. It contains an enzyme called tac polymerase. Now this is an enzyme that will produce lots of copies of DNA. It contains nucleotides because DNA is made up of nucleotides, so we need to have substrate in order to make the DNA and it contains primers. And these prime with a little short sequence of DNA nucleotides normally about 18 to 25 base pairs long and they lie either side as a piece of DNA that you want to have by. Now if you haven't been on the right hand side on that little box, you'll see the steps of the polymerase chain reaction. You start off with the DNA that you happen to double stranded PCR work on double stranded DNA, so you have to split it into two strands. So you heat it up initially to 95 degrees or 94 degrees in order to split the two strands of DNA. And then you have primers which you have designed and they come along and they will bind specifically, either side of the piece of DNA that you're interested in. angels match up with T's and G's always match up with CS. So if you know the sequence of a DNA strand, you can design a primer that will bind specifically to that DNA strand at a very specific point. But could you have the eight always lining up with the teeth and the DNA and the genes will be lining up with the seed? Okay, so we then see in the middle that we have primers lining up, and then you have the enzyme tackle DNA polymerase, which will then start elongating the DNA from where the primers come up until the reaction stops. And this normally is based on the amount of time that you leave the reaction going for the normally most Tac DNA polymerase will amplify kilobase of every minute that you leave the reaction going from. So there are two things to note from it. Firstly, you have big changes in temperature. You have denaturation at 95 degrees. You don't have a kneeling in the middle where the private buying is normally occurred between about 50 and 60 degrees and then you have the tacking a preliminary action which will occur at 72 degrees. And one of the cool things here is attack DNA polymerase comes from an organism that lives at very high temperatures. So it can withstand the denaturation step going up to 95 degrees. Without itself reading activities will become very important. In a second thing you can see that in this one step we are doubling the amount of DNA that we are going from one sequence or one piece of DNA to two pieces of DNA the doubling it's very important here because you don't just do one cycle of amplification in the PCR reaction, you normally do somewhere between 20 and 35 cycles. So that means that every time you do one cycle, you double the amount of DNA you start off with one strand after the first like you'll have two after the second four after the third eight after the fourth 16, etc, etc. And if you got to 35 cycles you end up with many strands of DNA. And it's also why the Tac DNA polymerase is important. It can withstand these high temperatures because you have to go through multiple denaturation cycles when you go through one of these new cycles so it needs to be able to then going through auditing denaturation the steps and still have activity at the end. And what you can see on the right hand side is actually a gel containing DNA that's been amplified by the PCR reaction. So after we've done the amplification, we can put it onto a Argos gel, give it a electric current, the DNA will go towards the positive current from me right. And then we can visualize it by using various chemicals and ultraviolet light. And you can see that there are bands of DNA that have been amplified in line late two and four but the PCR reaction in lane 3 haven't worked particularly well. And we can see on the far left hand side we have a DNA ladder, which has specific sizes of DNA that we can identify what size of PCR reaction is PCR IS LIKE COPYING THE PAGE OF A BOOK For PCR to work you need to know the DNA sequence. If you don’t, then the best way is to make a library. PCR The Whole Genome The piece of DNA you want to amplify and the primers you will use The 4 bases (ATCG) that make up DNA PCR Machine PCR MACHINE IS LIKE A PHOTOCOPY MACHINE : so you can think of PCR reactions as being something a little bit like a photocopier where we have a book, each page could be a gene. And we want to try to which would encode the entire genome. And we want to get hold of one gene. So one page of that, of that book. So the page in the bookmarks have sentences in we could look for primate binding sites within those sentences just to get hold of one page, we can then bind just upstream and downstream of the sequence that we want to amplify. We then have to have lots of substrates. So A T G's and C's nucleotide, primers, enzymes and things like that, where we in the case of the photocopy paper and ink, and then we have a machine where we put the samples to cycle through the denaturation in needling and synthesis step. Now, the important thing to note here is that as I said before, we need to know the sequence of the gene in order to do PCR reaction, because we need to be able to design primers to sit either side of the gene or to amplify it. And if we don't know that, then we can't use the PCR reaction. So if we can't do that, we do the next best thing, which is to make a library and then screen that library in order to try to identify genes that we're interested in. DIFFERENT TYPES OF LIBRARY Type of Library Description Contains randomly cut pieces of DNA isolated from Genomic the nucleus. If it is from a eukaryote it contains both exons and introns Contains randomly cut pieces of DNA isolated from Metagenomic an environmental sample Contains DNA synthesised from RNA using the cDNA enzyme reverse transcriptase. Contains no introns. Detailed discussion : - libraries are essentially plasmids, which contain bits of DNA from various sources. And there a number of different types of libraries. - We have genomic libraries, - a genomic library, where we take genomic DNA this could be kind of chromosomal DNA from any source from humans and plants, from bacteria from yeast - And we cut that up randomly with restriction enzymes, and then ligate this randomly into different plasmids. - meta genomic libraries and meta genomic libraries where we're sampling DNA from the environment. - So we know that in laboratory we can only grow about 1% of all micro organisms that live in a particular environment. - So what do you do if you want to get hold of a gene from a organism that we can't grow? - Well, we just isolate DNA from that environment. - It could be from soil, from milk from seawater, whatever environment you're interested in. We isolate the genomic DNA from these micro organisms that live there. - We cut up the DNA and we put those into libraries. - And finally, we have what are called cDNA libraries. - And cDNA is something that you can make from RNA and you can convert it to DNA, using the enzyme reverse transcriptase - and this DNA, when you make it from RNA is called complementary DNA or cDNA, because the reverse transcriptase is making the complementary strand to the RNA. - So you can take RNA and you can then make cDNA and you can take the cDNA and put those into plasmids to make a cDNA library. PRODUCTION OF A GENOMIC DNA LIBRARY WHAT IS IT? - A library from the gDNA of an organism‘ (ALL the DNA in the nucleus) DNA fragment unknown? Isolate DNA and fragment using restriction enzymes Ligate fragments into plasmids Same concept as R-DNA technology Many (1000000000’s) of individual DNA fragments will be ligated into plasmids Restriction Bacteria take up enzyme plasmids with new DNA digestion Restriction insert eg. EcoRI enzyme digestion eg. EcoRI Detailed explanation : what we want to do with genomic DNA library is get hold of the DNA from the genome of an organism can be any organism that you're interested in. You just need to be able to grow up. Once you get a hold of a sample extract genomic DNA. You then take that genomic DNA and you cut it into lots of little pieces using restriction enzyme. The example in the diagram at the top says use econ one doesn't have to be current one it could be any restriction enzyme, as long as the plasmid that you're going to end up putting it into also contains that restriction site. But you econ one you'll end up with probably hundreds of millions of different pieces of DNA of different sizes from that genome. You then take vector DNA, again, you're going to need hundreds of millions of plasmid don't I should also said plasmids are often called vectors. You cut those plasmid was the same enzyme, in this case, the current one, and then you'd like gate the little piece of DNA into those cosmic using DNA ligase. And in the end, you'll end up with hundreds of millions of plasmids, each containing a different piece of genomic DNA. And you can grow all of these up and you're going to end up with a tube normally with about a milliliter of liquid in containing probably hundreds of millions of plasmids, each with a different little piece of genomic DNA. GENE STRUCTURE & EXPRESSION Gene structure: A Gene consists of regulatory domains (promotor, activator-, silencer- and terminator sequences) and an open reading frame (ORF) that contain exons and introns. Activator Silencer Promotor ORF Terminator ATG - This is a eukaryotic gene - Prokaryotic genes are simpler with no introns. DETAILED EXPLANATION OF HOW A GENE WORKS AND WHAT IT LOOKS LIKE : Genomic DNA contains lots of different things. So in this case, we have a piece of genomic DNA and this comes from eukaryotes. So from something which has a nucleus doesn't come from bacteria. And I know that because if you look at the, the open reading frame or the off, it contains both exons and introns. So in eukaryotes eukaryotic genes contain exons, which is a bits of code for proteins. And these are interrupted by introns, which are which basically don't code for proteins They space the exons together and genes can contain one exon or they can contain up to hundreds of exon depends very much on the gene. So this eukaryotic piece of DNA contains an open reading frame. The other thing to note here is that the Open Reading Frame had an ATG at the beginning at start codon ATG codes for methylamine It also upstream of the ATG had a few different things which basically describe where the DNA are going to be transcribed the activators, silencers and promoters so these things are used by transcriptional machinery to work out where the DNA needs to be transcribed. So this could be transcribed in a particular cell type. So for example, there are some genes that are only transcribed in liver. There are others that are only transcribed in grain it could be thing it needs to be transcribed everywhere, all the time. And that would be what's called a can get to Gene a constitutive gene, the one that's transcribed everywhere the entire time. It could be that it wants to be transcribed with different life stages of an organism. So humans express some genes when they're babies which they will never expressed again in their lives and other genes which are only expressed in old age. And this is all driven by the upstream region from the open reading frame. So we have activated silence and promoters and things like that. Now that the Open Reading Frame starts with ATG, Coco protein has got exons and introns. And this is then what gets transcribed for what protein gets made. And then finally, we have a terminator region. And this is saying when the RNA needs to stop being produced so in this case, when a gene has been transcribed, there's an enzyme called RNA polymerase that comes from I talked about this earlier in terms of central dogma. It looks or at least it can get information from the promoter, the silent in the activator, about whether it should be making RNA find the ATG, it transcribed, both the exons and introns of his open reading frame and these will go out and then there are enzymes that come along and cut out the intron regions and glue together the exon regions to make different one piece of RNA without any intron regions. And then the RNA polymerase stops making RNA when it finds the Terminator. So this is essentially what a gene from a eukaryotic organism would look like A PROBLEM THOUGH : - One problem with cloning is the identification of bacteria containing plasmids with an insert. - An antibiotic can select for a bacteria that contains a plasmid. BUT… - How can you tell if a bacterium contains a plasmid with an insert or not? Detailed explanation of the problem : - If you remember when I was showing the picture of how to make a genomic library, we said we can cut a genome with a restriction enzyme like eco one. - And we can cut a plasmid also with the restriction enzyme like Eco One. - But you might be asking yourself, What stops the ligated gluing the cosmic back together again? - Well, actually nothing. - And what you will find if you set up an experiment like this is that some of the plasmids will contain a piece of genomic DNA and other plasmids will not and they will just be like aged back together again. - And we want to be able to try to get rid of the plasmids that don't have any extra DNA glued in between the restriction site. - And we can do this and there's several technique for doing this. - So we have an antibiotic resistant gene in the E coli. so we can then take our library, transform it into e coli, plate these out onto media and we'll get individual colonies growing. Each one of which will contain a different plasmid. - But this doesn't tell us which of those colonies contained with a insert in SOLUTION TO THE PROBLEM : - complementation Special cloning vectors containing bits of the lac operon. These vectors contain part of the gene for β-galactosidase (also known as lacZ). β-gal catalyses the degradation of the sugar lactose. It can also use an artificial substrate, e.g. X-gal. When X-gal is degraded it produces a BLUE dye. DETAILED EXPLANATION TO THE SOLUTION : There is a little piece of DNA here called lacZ, where the multiple cloning site is. And it's this piece of DNA that allows us to identify the plasmid which contain an insert. And the reason for this is the lacZ gene encodes an enzyme called beta galactosidase. So beta galactosidase, also called beta gal for short. It's rolling in bacteria is to degrade the sugar called lactose, and lactose, the sugar, found in milk. So it's quite useful for the bacteria to be able to degrade this in order to get a hold of the monomers which you can then use in metabolism. But beta galactosidase can also take a substrate called X gal that had a very long name, but I'm just going to call it x gal. And when it takes x gal, instead of lactose, it converts it to something which then colored blue. So x gal is colorless. But when it's acted on by the beta galactosidase, it turns blue. So when you have an active whole LacZ gene, then and this has grown up in a tap in bacteria, you put this onto a media containing X gal the whole bacterial colony will stain Blue If on the other hand, if you cut the lacZ in the muscle cloning site, which occurs in the middle of the gene, and you insert a piece of DNA into that, then the lacZ gene will no longer be active. And it will no longer be able to take the x gal which means that the colony will stay white thus they will not contain an insert - complementation A fragment (the first 146 amino acids) from the lacZ gene (the α-fragment) is in the vector (plasmid) The other half of the lacZ gene is present in the chromosome. Beta galactose enzyme is actually made up by two parts. One part is imposed by a gene that's present in the, the E.coli genome. And the other part is encoded by the gene that I just showed you in the plasmid. And we need both of those genes in order for the enzyme to be active. So what does this system then actually look like in practice? In practice solving the problem looks like this DETAILED EXPLANATION : ✓ In this case, we have a plasmid which contains a lacZ gene on the left with a multiple cloning site or poly linker is another name for the multiple cloning site, interrupting it in the middle of the lacZ gene. ✓ We can then cut that poly linker with a restriction enzyme like in d3. ✓ We can cut the genomic DNA we've isolated with the same restriction enzyme, put them together. ligase and we end up with two possibilities. ✓ We have two possibility that the plasmid in the middle or felt like a to go back to being like the cabinet on the left. ✓ This will then be able to produce an active lacks that protein mean that it will stay in blue if it's grown with X gal ✓ If on the other hand, we have a gene that gets inserted into the poly linker it will be unable to produce an active lacZ of protein. And this colony will stay white if you've grown up with Excal. ✓ So what does this look like in practice? Well, in this case, we will have taken a mixture of plasmids which don't contain the insect with passwords, which have reached do and transformed it into E. Coli. And then plated the they call out the plate containing X gal we have a mixture of white colonies and we have a mixture of blue colonies. ✓ The white ones contain an insert and the blue ones don't. ✓ So we want to get rid of the blue colonies and there's a very easy way to do that you can sit there with a toothpick and just be called a white colony. ✓ Of course in practice that that is very time consuming. ✓ So what people actually do is that they use Image Analysis software to pick all the different white colonies and then leave all the blue colleagues on the plate in order to make a genome, the genomic library, which contains plasmids only containing inserts. The technique : making a genomic library : So to go back to our example of making a genomic DNA library, in this case, we're making it from hummingbirds. So we have plasmid growing up in bacteria, we isolate them. We have a hummingbird which we can isolate genomic DNA from. We cut both the genomic DNA from the hummingbird and the plasmid with the same restriction enzyme. In this case, they got sticky ends of that could be cut with the current one We add the genomic DNA together with the plasmid and the DNA ligase You’ll get plasmids which don’t have pieces of DNA in it but many which do have DNA in it Nonrecombinant plasmid Recombinant plasmids Bacteria carrying plasmids You then transform these into bacteria You then put these on to a plate containing X gal and pick out the white colonies This will end up making your genomic library DNA can be used for recombinant protein production of: 1 Protein hormones 2 Enzymes for washing powders 3 Vaccine epitopes 4 Molecular biology reagents ❖ Once you have a library, you want to be able to do something with it. ❖ And normally what you want to do is you want to try to identify some genes which are important either for an industrial process or for trying to understand the biology of an organism. ❖ And once we get hold of those genes, we definitely want to do something with it. ❖ And there are many industries that are based on identifying gene often for micro organisms, in order to make enzymes for example for washing powders ❖ There are other things you can do with Gene and you can make protein hormones like insulin or human growth hormone. ❖ You can make epitope for vaccines to help fight things like COVID-19 you can make molecular biology reagent. ❖ So the restriction enzymes I talked about earlier, will generally all come from identifying a gene which encodes a restriction enzyme, and then using that to produce that restriction enzyme protein DNA REPLICATION OCCURS NATURALLY - DNA is synthesized in the cell naturally by using a number of different enzymes But we can also synthesise DNA Step 1 : Oligo synthesis - The first step in making DNA is making oligonucleotides - part of the PCA PCR reaction utilizes oligonucleotide, which bind either side of the bit of DNA that you want to amplify. - In this case, we're making the same thing. - So oligonucleotides which contain anywhere between 20 to 50 base pairs not for them to bind to the DNA but so that we can actually then start synthesizing DNA. - We can synthesize short bits of DNA called oligonucleotides in the laboratory Step 2 : DNA synthesis - Primers are normally about 50bp long, with 20bp overlap - Extension PCR fills in the gaps between primers - Larger DNA molecules can then be assembled together using a similar extension PCR process - Eventually the entire gene can be amplified by PCR using primers specific to each end Detailed explanation of DNA synthesis : - So in order to synthesize DNA, - if you look in the top left of the diagram , you see a couple of different things labeled f1 to f5 (forward primers) r one to R five(reverse primers), and essentially, these are primers that are making up a forward strand of DNA and a reverse strand of DNA. - Now, normally, when you're making these primers are longer than we would normally use for the polymerase chain reaction. - I said in the last lecture that they were normally for the PCR reaction, normally about 18 to 25 base pairs. - In this case, they're about 50 base pairs and they have overlap to the prime is between the forward and reverse primers. - And then there are gaps between the forward primers and gaps between the reverse primers - and these are filled in by the sequences in between f one and F two in the primer r1. - So if we just make up the prime and we put them into a tube, they will naturally align because they have these 20 base pair overlaps and - as I said to you before, nucleic acid will bind A's and T's and G's and C so if you design this correctly, the ends of the primers will line up in a particular sequence. And then what we do is we just fill in the gaps using the polymerase chain reaction. - So we would take this primer or these sets of primers, add Tac DNA polymerase and all the ingredients that you need for the PCR reaction and attack DNA polymerase will just fill in the gaps between f one and F two and F two and F three and F three and F four etc - and then the same with the reverse strand between R two and R one. - We will end up with two strands of DNA which are essentially mirror images of each other look near to each other. Now these might be a few 100 base pairs long. - But we might very well want to make a much larger piece of DNA so many 1000s of base pairs. - So the next step for that is basically to do just exactly the same thing. But using the bits of DNA that we have synthesized. - So we take these larger bits of DNA, we design them for that they have overlaps and the overlaps will kneel at the end to each other. - And then we just do PCR we make larger bits we can put those together with even larger bit sand by doing that we can just build up a new sequence or at least a new strand of DNA. ADVANTAGES OF CREATING DNA THIS WAY 1. Does not need template DNA (advantage over with PCR) 2. Can optimise DNA sequence for a particular organism to help enhance expression - There are a couple of advantages of using the synthesis technology over amplifying genes using PCR. - For example, you don't need to get hold of template DNA. - And it's possible you might be interested in trying to get hold of a gene that's present in some organism in a faraway country, and you're unable to get hold of that organism in order to get DNA tagged as template in the PCR reaction. - As long as you know the sequence, you can just synthesize a DNA by sending sequence off to a company and a few weeks later, they will send you back the gene that you are interested in. - The second reason is that you might want to optimize the DNA for expression in a particular organism. - In most laboratories we use mainly the bacteria E.coli or the yeast Saccharomyces cerevisiae is workhorses, in order to get gene expression and protein production of gene from other organisms. - Now, sometimes, the organisms that we are taking the gene from don't express particularly well in these micro organisms. - The reason for this is that E.coli uses optimally certain codons. - So certain of the three letter codes for particular amino acids differently to some other codon. - So if you remember back there are some amino acids that are encoded by up to six different codons. - And E.coli will use one or two of those codons in preference over the other four - other organisms will use as a codon so may might use some of the other ones that Ecoli doesn't use very often. - So if you know that you can synthesize the gene and you can optimize it, so it uses the codons that are used most frequently in these micro organisms, - you get better expression and better protein production that way WHAT HAS THIS BEEN USED TO DO? No Induction Native construct Codon Native construct optimised As you can see the gel picture here. Now, this picture is showing the proteins which have been extracted from the bacteria E.coli and separated out based on their molecular size in an SDS page gel. So, basically the top you've got big proteins and if you go down to the bottom you get some smaller proteins which have been stained using the dichromatic blue. Now, what the people have tried to do in this experiment is to express a protein or to make a protein called protein Beta. On the far left, you will see extract from cells where there's been no induction, so they're using a promoter here which is induced by adding a specific chemical and you will see that there's no protein B to be made. Then we have two native contracts which are being taken from an animal where they've amplified the gene using PCR. And you can see that the little bit of protein beta are being made. Within the in between the two native constructs. You have this codon optimized construct, where people have optimized it to use codons, or to contain codons, which E.coli produces optimally and here you get much more protein being made because E.coli can make more RNA. This is an advantage of synthesizing your DNA is that you can optimize it for the particular microorganism that you might want to express the gene ARTIFICIAL LIFE 1000 000 bp genome from Mycoplasma mycoides was synthesised and transplanted into Mycoplasma capricolom - With the knowledge of DNA synthesis I talked about in the last couple of slides, people have started using that technology in order to try to create artificial life. Now, this was the this slide is the first attempt to - people doing and what they were trying to do with it. - They were trying to recreate the bacteria microplasm myocodies - This has a particularly small genome around about a - million base pairs and they knew the sequence of it. - So the first thing they did was they designed all the nucleotides I explained, and they used this to produce large bits of DNA that make up different sections of the genome. - They then use various tricks and you don't - really need to understand this new thing ecoli and - the Saccharomyces cerevisiae AI to take these - large bits of DNA and assemble them together - into a complete genome. - And then they took another highly related bacteria - mycoplasma, Capri colon, and they removed - the DNA from those bacteria. - So they had cells which had no DNA in and they replaced that DNA with the microplasm mycodies - Then they faded it out and they had a look and they found that they were actually producing mycroplasm mycodeis and my quantities instead of the mycoplasma Capri covered on and they could - show this by sequencing the DNA was in the genome. - And they also went a little bit further and they were able to demonstrate that the DNA that we've used that was within with one they had synthesized and not some contaminant coming from Mycoplasma mycoides which may have been present within their their samples and they could show this because they wrote in code, various sentences of using the four base pairs of DNA in the genome that they synthesized. - So they could actually then demonstrate that the genome was they had, were definitely want that they're transplanted MINIMAL GENOME PROJECT A group are examining Mycoplasma genitalium which, when they started the project, had the lowest number of genes (475) known in a microbe. (Since then some Candidatus species have been identified that contain fewer that 200 genes.) - This type of technology has fed through to what's called the minimal Genome Project. - And there's a project where people are trying to identify the smallest number of genes necessary for bacteria to survive. - And people working on this project started off using a bacteria called Mycoplasma genitalium, because at the time it had the smallest number of genes ever identified in a bacterial genome only 475. - Since then a couple of other species of candidatures have been identified, who actually only have 200 gene, but there are advantages to using mycoplasma in an labaroty. - So this is a species that people have been working on They have found that 372 genes are necessary for this bacterium to survive - Researchers have been doing : being trying to identify which of those 475 genes is essential for growth. - And the way they do this is to knock out each gene individually and see if it's lethal, or if the bacteria can still survive without that particular gene. - And by doing that, they've identified 372 genes from the mycoplasma which are essential for it to grow and by using that they can then start to synthesize the minimal genome when they synthesize a piece of DNA, which contains only the necessary genes and remove all the genes that the bacteria don't actually need. MINIMAL GENOME PROJECT The minimal genome has been synthesised and transplanted into cells containing no DNA leading to the first artificial organism Mycoplasma laboratorium - Researches have synthesized a synthethic genome only containing those 372 genes - They’ve then taken a mycroplasm and removed DNA and then added in this synthetic bacterial DNA HOW CAN WE ALTER ALREADY EXISTING GENOMES? 1. Transgenesis (putting an artificial construct into a genome at a random place) 2. CRISPR/CAS9. Technology that alters a genome at a a precisely defined sequence Detailed explanation : Okay, now what you have to think talking about allows us to create a new genome. But generally, that's not what we want to do, what we want to do is we want to affect organisms in order to make them do something slightly different. That's advantageous to humans. And the way we do this is to a couple of different techniques. We can use something called jab transgenesis where we add a new piece of DNA into the genome. And this will go randomly into the genome, but it will then affect gene expression, either by reducing the amount of expression of a particular gene or producing more RNA of particular gene. The second type of technology is called Genome editing using the enzymes CRISPR CAS9. This is the main modern technology it's only been around for about a decade now. And instead of randomly putting DNA into genome like this transgenesis what CRISPR cast nine does is it alter the genome at a very specific defined place. That where we know that the genome editing is going to occur TRANSGENESIS I'll start off talking about the previous slide. This is where we add a new bit of DNA into the genome of a plant or an animal in order to effect the expression of a gene. So tried to get it to make more RNA, or less RNA.. Today, I just want to show you the kind of DNA constructs that we put into an organism in order to affect gene expression. Overexpression – Adds a new activity, or increases the amount of one already present ATG Stop Promoter Gene Terminator - Now the first kind of construct I'm going to talk about are ones which lead to either new new activity being present in an organism, or where you increase the amount of enzyme activity that's already present. - And there are probably different reasons for doing both these kinds of experiments. - If you're introducing a new activity you're normally wanting to make something novel in the organism. - You could be wanting to make, say a protein that could be used for a vaccine or a hormone. - Or you could be wanting to try to get the organism to make a new chemical that can be used at the drug. - If you're trying to over express an enzyme is already present in an organism, and therefore to increase that enzyme activity, you might be wanting to just to increase the amounts, flux particularly the biochemical pathway, so you end up with more something that the organism would normally make naturally. - And these kinds of constructs look like the diagram at the top of this slide where you have a promoter, a gene, and a terminator. - And we make these constructs in in the plasmids, I was talking about in an earlier lecture for the circular bits of DNA. - And we could amplify up each of these elements, the promoter, the gene or the Terminator, we could synthesize them and we could then use ligase, the restriction enzymes to cut them and to glue them together in the order that you see on the image - The promoter will tell the organism where and when to make this gene and how much so often you would want to have a constitutive promoter to tweak the gene everywhere to very high level the gene is what the protein that you want to make, and then the Terminator stops the production of RNA from that protein. - Now, these kinds of constructs are often called sense constructs. - And that is because the gene is sense orientation. And I'll compare that to antisense in a second. And it's incense orientation, because if you have the start codon, just after the promoter and the stop codon just before the Terminator - so that means that all the codons within the gene can be read from the start and stop in order to produce a protein. This is insense orientation OVEREXPRESSION - These are just some examples where people have used these kinds of constructs. - On the left hand side, we have a mouse on the left, which has grown obese because it's overexpressing, a gene called Pep- carboxylase. - So this tells us something about the role of this gene, in fact deposition and it tells us something that it's important in this process. - On the other hand, we could also take a gene from another organism. - So say the gene that encodes a protein that spiders used to make silk and we can express that in an animal in order for it to start making spider silk. - And finally, silk has some very interesting properties it can be used in bulletproof vests, for example, TRANSGENESIS - Antisense and RNAi– Represses expression of a gene present in an organism Antisense Stop ATG Promoter Gene Terminator RNAi ATG ATG Promoter Gene Gene Terminator - The sense construct to show to you excuse to either introducing new enzyme activity, or to increase the activity which is already present in an organism. - The two constructs above are the antisense and RNAi constructs, are designed to do the opposite. - They're designed to remove the activity of an enzyme that is normally made from RNA from a gene that's present within that particular organism. - I'll start off with the antisense construct. - Here we have a promoter, a gene and a terminator looks very similar to the awnaw conaruct that I showed you a couple of slides ago. - But in this case, the gene is in what is known as antisense orientation. - So if you go back a couple of slides, you'll see the gene has the ATG just after the promoter, which at the start codon and then the stop codon just before the Terminator. In this case, it's in the opposite orientation, which is the antisense orientation. - So in this case, if you make this construct in a plasmid, transform it into an organism, the organism will make the antisense RNA starting from the stop codon, and ending at the ATG. - This antisense RNA will go out into the cytosol. - And it will because it's a mirror image of the sense RNA that's been produced by the native gene. - It will just naturally bind to it. - And this will then form double stranded RNA and when it does, this the plant starts to degrade the double stranded RNA, because it thinks is coming under attack from an RNA virus. - Because RNA viruses often make double stranded RNA. - So the organism just naturally started degrading the double stranded RNA and remove both the sense and the antisense strand. - The effect of this is to remove the sentence RNA from the organism, which means that the native gene which is making the sense RNA the RNA will get degraded and therefore will not be able to make protein. - Now the second construct the RNA I construct is kind of quite similar. - Got a promoter and a terminator. - And it's got two little bits of the gene which identical in sequence, but they're an opposite orientation. So one is in sence orientation, going from the promoter up to somewhere in the middle of the construct and the other one is an antisense orientation running from the ATT by the Terminator, also up to the middle of the constuct. - And they are separated by a little bit of what's called spacer DNA. - Now the effect of this is that when the organism starts transcribing, it will start transcribing the sense RNA from the ATG after the promoter all the way through to the ATT just before the Terminator this RNA will go out into the cytosol and because two bits of plant DNA or organism DNA the two bits labeled gene in the construct identical in sequence but mirror images of each other. - They will just naturally line up and form double stranded RNA separated by a spacer. - Again, the organism will look at that double stranded RNA and it will think it's being invaded by an RNA virus and it will start degrading that double stranded RNA. - Now, interestingly, the way It does this it cuts up the dna to little 23 base pair nucleotides, and it will then go and bind to the to the native sense RNA of the the gene that you want to remove. And when it bind to this, again, the organism thinks that this is an RNA and it will start degrading then the sense of RNA - for producing this kind of natural double stranded RNAi vector, - we can then start removing the sense RNA coming from the gene is present in the organism. - Now, the main difference behind these in terms of their effects is that the RNAia is actually a much more powerful way to repress gene activity. - You normally see a much better effect with RNA than you do with an antisense construct. ANTISENSE AND RNAI EXAMPLE Repression of polyphenol oxidase (PPO) stops apples browning - So an example of where people have used this for an industrial product is this apple here the Arctic, which is currently on sale in the United States. - And what this does is that they've removed an enzyme that causes apples to go brown. - When you cut the apple and you can see that arctic apple on the right hand side has been cut. - The conventional apple on the left hand side has also been cut but the one on the left hand side is going brown and the one on the right is staying relatively yellow. - but, you might be asking yourself, well, what pointers if you don't mind eating about Apple? - The reason for this is actually something consumers generally don't like. - And a lot of apples gets thrown away in the food industry, because they turn brown. - So using this kind of technology, you can reduce food waste by giving customers the preference of having an unstained Apple even though it's been cut CISGENESIS Cisgenic plants only use DNA from that plant. e.g. promoters, genes and terminator sequences from apple to make an antisense construct is transformed back into apple. The apple bafore I just showed you has a new piece of DNA inserted into its genome. But it's technically not a transgenic organism. Although it's had a new piece of DNA. It's technically what's known as a cyst genic organism. And because all of the DNA that was put into that Apple had originally come from an apple. So the company that was making this it difficult, they took the gene from the Apple they took the promoter from the apple, they took terminated from the termination from an apple. They made a construct in in a Catholic vector. And then they use that to transform the promoter, the RNA contract and the Terminator back into the into the epigenome piece of DNA that have gone in there, but it all come previously from apples, and therefore classified as the cis genic organism. Now, this is somewhat important because there's quite a lot of debate at the moment about whether cis genic organisms should be classified in the same category of transgenic organism. I s it a genetically modified organism if all the DNA comes from that organism? Different regulatory authorities have different ideas about this though, for example, the United States cis genic organisms are seen as being different to be to transgenic organism and more similar to a natural variety of plant. While, in Europe, sis genic organisms are seen as being transgenic CRISPR/CAS9 – A NEW WAY TO MANIPULATE GENOMES CRISPR and Cas9 are bacterial genes that help protect the bacteria against infection by viruses by recognising and cleaving viral DNA. Detailed explanation : ❖ We will talk about genome editing using CRISPR cas9 ❖ this kind of genome editing allows us to edit the genomes of organisms at very specific places. ❖ Now, the way that the reason that people have identified CRISPR cas9 genome editing is from research that being performed in bacteria and the people working on bacteria looked into the genomes of bacteria, they found little areas, which contained viral genes. And they were a bit confused as to why you bacterial DNA will contain the viral sequences. ❖ And then they worked out that these are actually defense mechanism of the bacteria since the bacteria is invaded by a virus, it tries to drive these CRISPR loci with the viral genes. ❖ They will go into identify viral and they will bind to that DNA at the strands which are analogous to the DNA from the CRISPR loci. ❖ And they will guide enzymes that the bacteria produce called cat nine, which will then cut up the DNA at those specific points. ❖ And by doing that they can inactivate the viral DNA and stop the virus from invading the bacteria. ❖ When people thought that they thought well maybe this will work in other organisms, we can, instead of using bacterial DNA at CRISPR loci, ❖ we can then use plant or animal DNA in order to target specific loci or specific points in the genome where we where we can then use the cas9 enzyme in order to cut the DNA This has been manipulated to help modify eukaryotic genomes Detailed explanation : ❖ Researchers have been using technology to manipulate genomes of both plants and animals. In fact, pretty much every organism now. ❖ And what they do is that instead of making viral DNA, they make DNA from a gene and organism that they want to manipulate. ❖ They put this RNA into the organism, and then they also put the cas9 enzyme into the organism. ❖ The RNA will go to a very specific point of the genome that the researchers are interested in. ❖ And the caste nine will come along and it will cut the chromosome at that point. ❖ Now, you might be asking yourself, what's the point of doing this? ❖ Why would you want to cut a chromosome? ❖ Well, what happened at this point is that the repair mechanisms in the organism and the repair mechanisms will try to glue the chromosome back together again. ❖ But these repair mechanisms are often not particularly good. ❖ And they often add or remove few base pairs when they glue the DNA back together again. ❖ And this will essentially lead to a mutation interested in from very similar effect to the RNA or the the antisense construct I was talking about, but in this case, ❖ you will mutate a gene, which will mean that it will be unable to produce a protein that it would normally choose and you can remove that protein from the from the organism WHAT HAVE PEOPLE USED THIS FOR? - So what is CRISPR cast nine being used for? - Well people have made musqeutoes don't get affected by the malaria parasite. - And the idea here would be that you could then release these into the environment and outcompete all of the all of the the mosquitoes that would present in the environment that would normally get infected by the malaria parasite to try to reduce the amount of malaria - people have made plant which are resistant to disease. - We have a example here of grapes which are resistant to mildew by deleting or removing a protein that normally gets made. - And using a different form of CRISPR cas9 - people have also started to try to cure HIV in this case, and they've cured muscular dystrophy in mice, - not by mutating a gene but by introducing a healthy gene back into the genome of mice. - This is just another kind of form of CRISPR cast nine Microbial biotechnology : Micro-organisms…..”animalcules” MICROBES – GENERAL Microbes grow fast 600 500 Total biomass 400 300 200 100 0 0 2 4 6 8 10 12 Generations 100 µm 20 µm 1 µm Bacteria on a needle’s tip - Micro organisms grow, kind of the faster things can grow incredibly fast. - The main microorganism that we use in the laboratory is the bacteria e.coli and this will double about every 15 to 30 minutes in the kind of laboratory conditions that we grow them in - and people to ask what the general amount of microorganisms on the tentative and they live everywhere. They live on us. They live enough they live in all the ocean they live in all the land areas on the planet. - People have worked out, that actually more than half of the biomass on the planet come from microorganisms. - You can see on this, this sort of data here that people have estimated that life on Earth is around about four and a half times 10 to the 11 times and micros make up about 2.8 times 10 to the eleven tons, which is 60% of the entire life on the planet. - This is some electron micrograph picture of what I think probably Ecoli looks like on the tip of the needle. - And you can see going with increasing magnification from left to right there on the left -. On the far left, you can see just about some kind of colonies. - On the middle picture. You can see the actual colonies of kind of individual cells on and then on the far right you can see the individual cells with around about one micro meter long. - Now, as I said, microorganisms grow incredibly fast, some of them will double every 15 minutes or so. - And if you look at how they grow, you can see that they have this kind of exponential growth rate, where they start off relatively slowly, but because they double every generation, after a small number of generations they to start producing a huge amount of biomass. WHY DON’T MICROBES TAKE OVER THE WORLD ? - So, despite their numbers, exponential growth and generation time, bacteria have not taken over the world. - Why? Because, like any other organism, their growth is limited by the availability of nutrients and by the build up of their own toxic by-products. - Bacterial growth curve We can thus see that if we wish to use a bacterial culture for biotechnological purposes, the growth conditions need to be very carefully controlled. Microbes do not take over the world because … Their growth is limited by the availability of nutrients. They produce toxic compounds. The reason for this is because micro organisms are limited in their growth by a number of different things. They're limited by the amount of nutrients to available in the laboratory we grow them with the optimal amount of nutrients which allows them to grow incredibly fast. But out in the in the wild, they're often in areas where there are little nutrient and they can only grow quite slowly. Secondly, they also produce toxic compounds in order to remove competition from the environment. Where they're living. Look at this diagram here, this shows what the rough growth curve would be of an organism, especially in a kind of a contained culture in the laboratory. They started off in what's called lag phase, where they grow very slowly with a few cells. And then eventually, after a few generations, they start increasing very quickly and hit what's known as either log phase or exponential phase. This is where there's lots of nutrients, so they don't have any limitations. But eventually, they produce so many bacteria, that it becomes very crowded. And this is when the nutrient start becoming limiting. So they've taken the nutrient out of the solution, and they basically then can't increase anymore. And they enter what's known as stationary phase. After quite a long time in stationary phases can be actually many months or many years, they will start dying off. And this is often caused by toxins that they start putting into the environment in order to kill off competing bacteria and eventually they hit what's called long term stationary phase where they can produce it. In some bacteria, they can produce some kind of cyst, which can survive for many decades, or actually many hundreds of years, without dying until it becomes erected. MICROBES ALSO CAUSE DISEASE 1. Pandemics are epidemics that spread over a wide geographical area a) Plague b) Smallpox c) HIV d) Ebola e) Zika f) Covid-19 2. H1N1 virus responsible for Spanish Flu BIOLOGICAL WARFARE - First recorded case of biological warfare was in 1348 - A Mongol army invading Europe catapulted the bodies of soldiers who had died of plague into a city in Crimea. It is thought that this led to the black death pandemic that killed 75 million people worldwide, and 1/3 of the population in Europe. So clearly, biological warfare in bad. But we can combat it using modern technology Smallpox killed between 300-500 000 000 people in the 20th century alone. 30% of individuals infected with the virus died Vaccines. To combat smallpox, people have infected themselves with the closely related cowpox for centuries. Smallpox is one of the great success stories of science in the 20th century. You can see here figures that in the 20th century between 300 and 500 million people died of smallpox, with about a third of everybody who got infected dying. Now, we managed to wipe this virus off the planet or at least within human population by the mid 1970s. So all those deaths occurred in the 20th century between 1900 and around about 1975. And we did this by mass vaccination programs. Now people know simply at least the late 1700s that infection by other kinds of diseases like cow pox, which is very closely related to smallpox leads to people becoming immune from being infected by smallpox. So it's actually many hundreds of years people purposely injected themselves with cow pox in order to protect them against smallpox. But it was only in the 20th century that managed to be able to produce backing in the lead to mass vaccination program. VACCINES : Vaccines can also be made using genes from the disease causing agent, which are expressed in a non-pathogenic organism. Means that there is no possibility of infection when the vaccine is used. - How do you Make a vaccine? - Well, as I said, the smallpox vaccine is a it's another virus, it's quite closely related to small box iand cowpox - And this will infect humans will give them a mild defeat, but it will lead to immunity from smallpox. - the polio vaccine, on the other hand is actually the polio virus which is heated up and disarmed. - This means that it can't infect people, but it can be recognized by the body as being the polio virus that leads to the production of antibodies. - A more modern way of producing antibodies is to make what's called recombinant protein. - So here what you do is you take a virus like in this case, a hepatitis B virus, and we know that one of the proteins made by Hepatitis B which is on the upside of the virus, is recognized by the human body and is then used to make antibodies. - So instead of taking the Hepatitis B virus and disarming it by heating it somehow because there's still the potential that this can lead to disease, we take the gene that codes for the protein, the one protein from hepatitis B virus, that causes the antibody response. - We then do what I talked about a couple of lectures ago, we take that gene and we insert it into a plasmid. - And this plasmid then transmit then goes into yeast cell and the cell starts making the Hepatitis B virus protein. We can then drop that use so we can isolate the protein and we can use that to make a VAC vaccine. - So you take that purified protein, you inject it to people, and they the body recognizes that protein and starts making antibodies against it. - So when if you get infected by Hepatitis B, you have become immune to it. - But you don't have to make these proteins in yeast. - You could also make the bacteria, people make them in plants, for example. - But nevertheless, this kind of technology where you're taking a gene from an organism that causes the disease, make the protein from that and then use that to the vaccine is useful for quickly making a lot of vaccine. It is really important to become vaccinated because of herd immunity. Helps people who cannot be vaccinated e.g. if they are immunocompromised you may have heard about in terms of COVID-19 is herd immunity. And with COVID-19 people are saying what potentially we just let the vaccine run through a population. And once about 60, or 70 or 80% of the population have had the vaccine and recovered from it. They're likely to be immune, but we don't know how long that immunity is going to last at the moment. And if we get enough people infected and immune, it can stop the spread of the disease. herd immunity kind of became a concept when people started vaccination programs. And the concept is illustrated on this slide here. If nobody is vaccinated, then the one person gets the disease, it will spread without the population. But if you increase the number of people who have become vaccinated, then you can decrease the spread of the disease. If one person gets the disease, and is surrounded by people who are immune to the disease and it cannot spread, because they don't come into contact with anybody else. Who is not immune to the disease. This is why it's really important to get vaccinated, so that we can introduce this herd immunity into population in order to stop diseases spreading if they do actually come into a population. And this is especially important for people who are immunocompromised who potentially cannot become vaccinated. Some vaccines still use attenuated viruses, which can infect people especially if they're immunocompromised BIOLOGICAL WARFARE Can we make disease causing agents more dangerous? 1. More infectious 2. Resistant to antibiotics 3. More deadly 4. Overcome immunity from current vaccines…… - We could, for example, overcome antibiotics by taking known antibiotic resistance genes and make them better at resisting antibiotics e.g. they lead to resistance against more than one antibiotic or can resist higher amounts. DETAILED EXPLANATION : - And if after your degree you want to go and work for somebody like Dr. Evil and make organisms which are actually more dangerous. - How could one do that you might think you could make an organism that becomes more infectious. - You can make a bacteria that becomes more resistant to antibiotics. - You can make something that's more deadly or something that can overcome immunity from current vaccines. - I want I want to talk about potentially how one could think about making organisms which are more resistant to antibiotic. - already some bacteria contain genes that make them resistant to certain types of antibiotic. - And often one way to overcome this is to increase the amount of antibiotic that you give to a patient because often the antibiotic resistance gene will only lead to bacteria being resistant to low levels of antibiotic. - But can we make these kinds of genes code encode proteins? - Which can overcomes increased amount of antibiotics that you might give to something - We can do this using gene shuffling, also known as directed evolution GENE SHUFFLING : And the answer is it is possible to manipulate genes that encode antibiotic resistant proteins in order to make them more resistant to antibiotics. So, what this slide is showing you is something known as either gene shuffling or directed evolution. And this kind of technique is a way of improving proteins for a particular process. So in this case, we have 4 genes from different bacteria, each of them encoding the same antibiotic resistant protein. And scientists have tried to improve them in order to make them more resistant to antibiotics. Now, there are two different techniques here on the left hand side you have what's called single gene shuffling. And here what they're doing is they're taking the individual genes, and they're altering the genes using chemicals or some way of just altering individual base pairs. This will then change amino acids, one at a time within the protein. And by taking the mutated gene putting them in plasmids and forming them into bacteria, and then placing them out onto plates containing increased amount of antibiotic. They could show that spike is altering the odd base pair and the amino acid they get up to about an eight fold increase in resistance to antibiotics. A more powerful way of doing this is shown on the right hand side. In this case, scientists took the four genes and instead of doing or changing just the base pairing on amino acid they cut up all the genes using restriction enzymes. And they ligated them back together again in a random way. So each of the different bits of DNA shown in the middle on the right hand side contains a little bit of resistance genes between the different bacteria in a random order. Now, again, they can take these constructs these bits of DNA, they can like get them into plasmids, transform them into bacteria, plate those bacteria out and what they could show with using this technique on the right hand side multi gene shuffling they got a much higher increase in antibiotic resistance to up to about 270 to 540 fold increase in resistance. Now, you might be asking yourself, what's the point of this? Why would we want to alter antibiotic resistance and make bacteria more resistant to antibiotics? And of course we don't that's the opposite of what we want to do. This is just to illustrate the process. But this technique is used hugely in order to alter the properties of enzymes used in industry. So there are industries for example, the washing powder industry, which contains a large amount of of enzymes which will degrade state of your clothes. But often the enzymes that are isolated from bacteria living in the environment are not optimal for that industry. So for example with washing powder, what we do is to try to improve the enzyme so we could watch them close at room temperature rather than at 40 degrees. This would reduce the amount of energy that we use in order to wash clothes. And you can think about trying to change the activity of the enzyme to make them more active at lower temperatures. By using the kind of technology and then tried to select for enzymes that have this higher activity at room temperature compared to the activity they demonstrated the moment and by using these techniques, people may commonly try to alter the properties of protein for these kinds of industrial proseses. THE SMALLPOX VIRUS GENOME HAS BEEN SEQUENCED. CAN IT BE RECREATED? In 2002 Polio virus was created in a lab by synthesising its genome and allowing it to assemble Microbes can also be used for producing useful things So I talked in the last lecture about DNA synthesis and I talked about the minimal genome project in bacteria. But you might be asking yourself, Could we use that kind of technology to recreate viruses like the smallpox virus? And short answer is yes, we could definitely in principle I hope nobody out there trying to recreate the smallpox virus. But we know the secret to the smallpox virus genome has been published. People have synthesized the polio virus under the lab. And in fact, a number of other viruses nowadays, or since then have also been reproduced in the laboratory. I guess I should also say I would be really surprised if the entire smallpox virus had actually been genome sequence to be published. I would highly suspect that little bits of it have been left out and bits that are really important for the infection process because I don't think people would want the entire genome sequence to be present in the public environment. But we can do things we can use microorganisms for stuff that's not particularly useful like recreating the polio virus or the smallpox virus. But we can also use micro for producing lots of useful things and I talked in a couple of slides ago about using them to produce vaccines. The first protein produced by the pharmaceutical industry using recombinant DNA technology was insulin One of the first drugs that was made in a genetically modified organism was the protein hormone insulin. I think I mentioned this in a previous lecture when I said that before this this recombinant protein insulin was made people used to isolated from, from pigs who had been slaughtered. But what people did with it they identified the gene for insulin from humans. Now, in a previous lecture, I talked about genes containing exons and introns. Now, if you want to express a gene in bacteria and make a protein, you have to have a gene that doesn't contain any introns. So the exons if you remember the bits that code for proteins and the introns are the non coding bits that intersperse the exons within a gene in eukaryotes, prokaryotes don't have exons and introns. So they are unable to remove the the intron from in between the axon so they're unable to produce protein from a eukaryotic gene like, like the ones insulin, which contains both exons and introns. The way to get around this is to isolate cDNA. And I talked about cDNA libraries in the second lecture and if you remember I said cDNA libraries are libraries where people have isolated RNA and use an RNA to produce cDNA using the enzyme reverse transcriptase. So RNA is produced by cells from DNA. And in its very initial form, it contains both exons and introns. But very quickly, the introns get removed from the RNA. So you can amplify out the gene for insulin by taking getting hold of the RNA converting it to cDNA. And then using the PCR reaction to amplify out this, this gene not containing any insurance. What people did so they isolated the insulin cDNA from human pancreatic cells, they ligated it into a plasmid. They put this plasmid into a bacteria and they grow this up in big tanks. And all insulin in the world is now produced using this process. Before 1982 insulin was produced from pigs - About 2000kg of pig pancreases produced roughly 250g of insulin. - Some people develop resistance to non-human insulin. - Expensive - Producing insulin involves a very large scale industrial plant involving fermenters, centrifuges, purification columns etc ARTEMESIN AND MALARIA - In 2010 there were 200 000 000 cases of malaria and 650 000 deaths - 1 child a minute dies of malaria (50% decrease since 2000; Figures from the World Health Organization) - Treatable, but there is no vaccine available - Another thing that's major in microorganisms that I want to talk about at the end is something called artemisinin. - Now, artemisinin is a really interesting compound. - It was developed as a process after the Vietnam War. - And the reason for this was the Vietnamese soldiers were actually at one point losing the war, not because they were losing militarily, but because they were coming down with malaria. - And they didn't have enough kind of drugs in order to support the soldiers with malaria. So they asked the allies of China to try to supply them with anti malarial drugs, and potentially try to develop new drugs to treat malaria. - So what the Chinese did was, they went out into the countryside, and they started testing extracts from plants. - In order to see if these extracts demonstrated anti malarial activity. And they found one plant called Artemisia Annua, which contained a compound that that helped us with active against malaria. - At the time, we didn't know what the compound was but they spent a lot of time researching it. - And eventually they identified what this compound was. - I mean, I think that it is great to have a completely treatable disease. But we don't have any vaccine available currently and people keep trying with a vaccine, but we never seem to work particularly well. Artemesin is an anti-malarial - You might be asking yourself, Why am I talking about this and electron microbial biotechnology? - And the reason is that in the last five years or so, people have been developing systems in order to make artemisinin in microorganism rather than having to grow the plant up and isolate it from the plant. - And they did this because there they saw that there would be price fluctuations in artemisinin and this came because when prices were high, farmers would plant grow the plants up and of course, lots of farmers would harvest at the same time, which would lead to a glut in the market and decrease prices. - So then the farmers would plant something else to decrease in the amount and you get a big increase in price. - So people wanting to try to make a system where they could kind of even out these price fluctuations IN 2013 A NEW PRODUCTION METHOD CAME ONLINE USING SYNTHETIC BIOLOGY (THE RATIONAL DESIGN OF BIOLOGICAL SYSTEMS FOR USEFUL PURPOSES): This involved a two stage process 1. Production of artemesinic acid in Saccharomyces cerevisiae 2. Chemical conversion of artemesinic acid to artemesinin - The system that scientists came up with, uses the baker Saccharomyces cerevisiae as the host for producing something called awesome music acid. - Now this is not artemisinin, it's something very closely related. - And then they can harvest the artemesinic acid from the Saccharomyces cerevisiae AI and use chemicals to convert it through to our tummies CHEMICAL CONVERSION OF ARTEMISINIC ACID TO ARTEMISININ. established cultures of yeast that could actually make artemixic acid, they found that they had a problem. And this was the yeast actually weren't making enough automation ik acid to make the whole process commercially viable. To overcome this, they added a few different chemicals to the to the yeast, and that's shown in the graph on the left hand side of this graph. You can see at the bottom you have the amount of Artemide. On the bottom line you have for the mixed feed, which they have the lowest amount of atomistic acid being produced. They then added a chemical called IPM. And this is something that's solubilizes, the atomistic acid, and they found that they could produce more Automedia Nick acid and then finally the top line you'll ha