Auditory and Vestibular Physiology Primer (PDF)
Document Details
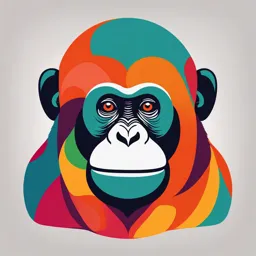
Uploaded by AccomplishedMagic
Stony Brook University
Kelly A. Warren
Tags
Summary
This document provides an introduction and summary of auditory and vestibular physiology. It explains the function, structure, and mechanism of hearing and equilibrium in the human ear. The document is geared toward an undergraduate audience and is likely part of a university course or class.
Full Transcript
Kelly A. Warren, Ph.D. Department of Physiology and Biophysics BST-T5, Rm. 180 [email protected] HEARING, EQUILIBRIUM, AND VISION The lecture material is provided in from Chapter 10 of Silverthorn’s Human Physiology Textbook. It is recommended that you refer to this text when studying. T...
Kelly A. Warren, Ph.D. Department of Physiology and Biophysics BST-T5, Rm. 180 [email protected] HEARING, EQUILIBRIUM, AND VISION The lecture material is provided in from Chapter 10 of Silverthorn’s Human Physiology Textbook. It is recommended that you refer to this text when studying. THE EAR: HEARING Because the ear has a purpose in both hearing and equilibrium function, there are components of this structure that are dedicated to these specific purposes. Overall, however, the ear consists of an external, middle, and inner portion. The external ear contains both the pinna and the ear canal and is segregated from the middle ear by the eardrum (or tympanic membrane). The middle ear is an air-filled cavity that contains connections to our pharynx (via a eustachian tube) and also holds structures (malleus, incus, and stapes) that are capable of transferring soundwave energy from the external to internal ears. The inner ear consists of apparatus that benefits both auditory and vestibular functions. Vestibular functions are governed by the vestibular apparatus concerning the semicircular canals, while the auditory functions are a result of the cochlea. The cochlea is a fluid filled and coiled structure containing hair cells necessary for the transduction of an auditory (sound) stimulus. 1 Sound: Hearing is the ability to turn sound (or pressure) energy into action potentials within the auditory system. Sound, on the other hand, is our interpretation of the frequency, duration, and amplitude of these waves. Sound is characterized by frequency (which gives us pitch; measured in waves/s) and amplitude (which gives us an index of intensity or loudness; measured in decibels). Hearing Transduction: A multi-step transduction process means that the energy of sound waves is not simply converted into action potentials directly. Instead, there is an initial conversion of energy to sound waves and mechanical vibrations within the external and middle ear, which is further converted to fluid waves within the inner cochlear ducts. These vibrations ultimately act to deform hair cells within the cochlea to produce neurotransmitter release, and subsequently result in action potential generation. 2 Cochlear structure: The cochlea is divided into three distinct ducts including, (1) the vestibular, (2) the tympanic, and (3) the cochlear. The vestibular and tympanic ducts are continuous with one another through a small opening called the helicotrema and contain fluid that is similar in composition to plasma (low potassium and high sodium). This fluid is referred to as perilymph. The cochlear duct does not have any openings to the vestibular and tympanic ducts, but does have a small opening to the saccule of the vestibular apparatus which reflects its role in equilibrium. The fluid of the cochlear duct resembles that of intracellular fluid, where the potassium concentrations are generally elevated and sodium concentrations are reduced. The fluid of the cochlear duct is called endolymph. The endolymph composition generates a potassium electrical and chemical potential that drives potassium into hair cells when channels are open. 3 The cochlear duct contains a structure called the organ of Corti, which is critical when considering the transition of turning pressure waves in electrical signals. The organ of Corti contains hair cell receptors and support cells and is attached to two distinct membranes, the basilar and tectorial membranes. The basilar membrane and tectorial membrane move in response to waves passing through the vestibular duct and result in an increase or decrease in the oscillations of hair cells within the organ of Corti. Hair cells are non-neural receptor cells that exist in the organ of Corti. They have an apical surface that is defined by stereocilium (finger-like projections) of successively increasing heights. The longest of these stereocilia is referred to as kinocilium. The kinocilium of hair cells attaches directly (and is embedded into) the tectorial membrane. So, as the tectorial membrane moves it produces movement of the kinocilium and stereocilium as well. The kinocilium and stereocilia are bridged together by proteins that act to keep ion channels either opened or closed, depending on which direction these projections are pushed. In a neutral position, about 10% of the channels bound by these proteins are open and there is relatively low incidence of neurotransmitter release and action potential propagation down primary sensory neurons. However, when cilia bend towards the kinocilium there is an excitation, which opens the plugs to these ion channels and allows for the depolarization of the hair cell membrane. Subsequently, depolarization results in the opening of voltage gated calcium channels that allow for the release of neurotransmitters from the basal side of hair receptor cells onto primary sensory neurons, leading to action potential propagation. If the stereocilia bend in the opposite direction (away from the tallest projection; kinocilium) there is an inhibition and a result of that is the closing of ion channels, ultimately leading to a hyperpolarization due to a reduction in cation influx. This reduces neurotransmitter release and sensory action potential frequency. 4 Signal processing within the cochlea: The initial processing for both pitch and loudness (intensity) occurs within the cochlear structure. Pitch, for example, is a feature of where the sound waves act on the basilar membrane. The basilar membrane differs in thickness and flexibility as it progresses from the oval window to helicotrema. Towards the round window, the basilar membrane is both narrow and stiff, while at the helicotrema the membrane is wide and flexible. Sound waves of different frequencies make it different distances down this membrane. High frequency sound waves (leading to high pitch sounds) act predominantly on the stiff and inflexible region, while low frequency sound waves (leading to low pitch sounds) reach the further, wider, and more flexible region. In this way pitch is also temporally and spatially coded, meaning that the auditory system will be able to sense the time it took to reach the membrane giving a context of location along the basilar membrane. This spatial coding is retained as information is processed along the sensory nerves and into higher auditory cortex centers. 5 Neural auditory pathways: Although the cochlea is responsible for the conversion of vibration waves into electrical signals, it is the responsibility of the auditory cortex to interpret the information being gathered by each ear simultaneously. The way this is done is through neurons (of the cochlear nerve, VIII) being activated by cochlear neurotransmitters and then projecting to the medullary region (cochlear nuclei) of the brainstem. From this region, projections extend for each cochlea (left and right) both ipsilaterally and contralaterally to the midbrain (superior olive) and thalamus (medial geniculate nucleus). The thalamus sends projections from both the left and right cochlea input to the left and right auditory cortex, so that each cortex is receiving information regarding the input of auditory information into the system as a whole (or, in simpler terms, both ears). This is quite important, since the auditory system is receiving simultaneous input from both ears, and in more complicated manners utilizing time differentials to detect the directions of where sounds are coming from. 6 THE EAR: EQUILIBRIUM Equilibrium is a result of the functioning of both vestibular apparatus within the inner ear and also the response to propioceptors dictating the placement of the body in space. For the purpose of this lecture, equilibrium is sensed in the inner ear through the vestibular apparatus which ultimately modify the position of hair cells (similar to that observed within the cochlea) and activate primary sensory neurons. In contrast to the cochlea, however, the forces required to move these hair cells are provided by gravity and acceleration, not sound waves. Rotational acceleration: The semicircular canals sense rotational movements. They are composed of interconnected tubes positioned at right angles to one another. The ending of each tube (canal) has an enlarged chamber referred to as the ampulla where cristae (sensory receptors) are present. Cristae consist of a gelatinous matrix where the cupula, a structure composed of hair cells, resides. The hair cells bend in response to the movement of endolymph against the cupulla structure and generate action potentials in sensory neurons. As the endolymph moves through the semicircular apparatus, it results in the bending of hair cells embedded within the cupula. The bending of the hair cells is in the opposite direction to that of the rotation of the head. 7 Linear acceleration: The otolith organs are composed of both a utricle and a saccule and their sensory receptors reside in the maculae. Each maculae consists of a gelatinous otolith membrane in which otolith crystals are embedded. Underneath these crystals lie hair cells that can be mechanically disturbed to release neurotransmitter onto primary sensory neurons. The maculae of the utricle is oriented horizontally when an individual is in the upright position, therefore it can sense movement of the head that is forward and backward. This is a result of gravity having the otolith crystals slide across the hair cells, opening up channels similar to that observed within the cochlea. The maculae of the saccule is oriented vertically when an individual is in the upright position and can therefore sense changes in movement upwards and downwards; this is in a similar manner to both the utricle and cochlear hair cell transduction mechanisms. 8 Transmission of vestibular information: Hair cells of the vestibular apparatus stimulate neurons of the vestibular nerve (CN VIII). The information carried by this cranial nerve participates in three major reflexes including (1) helping to maintain equilibrium and gaze during movement, (2) maintaining posture, and (3) maintaining muscle movement. Therefore, the targets of this pathway include the vestibular nuclei of the medulla, somatic motor neurons controlling eye movements, the cerebellum, and higher cortical centers. 9