AP Pulmonary part 2 2023 Rev 15.pdf
Document Details
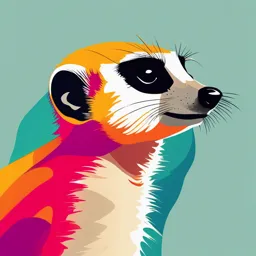
Uploaded by TemptingIndigo
Keiser University
2023
Tags
Full Transcript
Keiser University Dr. Joseph Curione PULMONARY SYSTEM Part 2 22-1 Objectives Control of Ventilation Integrated Responses Pressure & Flow Gas Exchange Mechanisms V/Q Ratio Flow-Volume Loops Lung Volumes & Capacities Elasticity/Compliance/Resistance Affects of Aging and Pathologies 22-2 Neural Control...
Keiser University Dr. Joseph Curione PULMONARY SYSTEM Part 2 22-1 Objectives Control of Ventilation Integrated Responses Pressure & Flow Gas Exchange Mechanisms V/Q Ratio Flow-Volume Loops Lung Volumes & Capacities Elasticity/Compliance/Resistance Affects of Aging and Pathologies 22-2 Neural Control of Breathing The normal automatic process of breathing originates from the brainstem Neurons in medulla oblongata and pons control unconscious breathing – The cortex can override these centers if voluntary control is needed The automatic rhythm is controlled by neurons located in the medullary rhythmicity center 22-3 Respiratory Control Centers Medulla – sets rhythm Pons – fine tunes rate & depth 22-4 Respiratory Control Centers Nuclei in medulla – set rhythm – DRG (dorsal respiratory group) Sends signals to diaphragm/intercostals for inspiration – VRG (ventral respiratory group) Sends signals for both inspiration & expiration Pre-Botzinger complex (pacemaker neurons c/ leak channels) – Sets normal rate (12-16 breaths/min.) Botzinger complex (controls expiration) VRG also involved in forced respiration – Recruitment of accessory muscles of breathing 22-5 Respiratory Control Centers Pons – fine tunes rate & depth of breathing – apneustic center Controls inspiration promotes maximal lung inflation and long, deep breaths – pneumotaxic center Controls transition from inspiration to expiration sends continual inhibitory impulses to inspiratory center, as impulse frequency rises, breaths shorter, faster and shallower 22-6 Central Control The expiratory area is normally quiet during normal breathing, but will become activated with forceful breathing (elastic recoil is passive) Pneumotaxic center in the upper pons – Inhibits inspiration – Limits the burst of action potentials in the phrenic nerve, effectively decrease the tidal volume and regulating the respiratory rate – “Fine tuning” of respiratory rhythm because a normal rhythm can exist in the absence of this center Apneustic center in the lower pons – Impulses have an excitatory effect on the Dorsal Respiratory Group in the medulla – Promotes inspiration – Sends signals to the Dorsal Respiratory Group in the medulla to delay the “switch off” signal provided by the pneumotaxic center 22-7 22-8 (-) (+) Dorsal group Ventral Group 22-9 22-10 Animation - Ventilation Control 22-11 Input to Respiratory Centers From limbic system & hypothalamus – respiratory effects of pain & emotion From airways & lungs – irritant receptors in respiratory mucosa stimulate vagal signals to medulla, result in bronchoconstriction / coughing – stretch receptors in airways - inflation reflex excessive inflation triggers stop of inspiration (Herring-Breur reflex) J-receptors – juxtapulmonary capillary receptors – sense interstitial fluid, Pulmonary Edema, PE, etc. à increase rapid, shallow breathing; sense of dyspnea From chemoreceptors 22-12 – monitor blood pH, CO2 and O2 levels Blood Chemistry and Respiratory Rhythm Rate and depth of breathing adjusted to maintain levels of: – pH – PCO2 – PO2 22-13 CENTRAL CHEMORECEPTORS 22-14 Effects of Hydrogen Ions pH of CSF (primary stimulus for central chemoreceptors) Respiratory acidosis (pH < 7.4) caused by ¯ pulmonary ventilation – hypercapnia: PCO2 > 45 mmHg CO2 easily crosses blood-brain barrier in CSF the CO2 reacts with water and releases H+ central chemoreceptors strongly stimulate inspiratory center – “blowing off ” CO2 reaction is to the left: CO2 (expired) + H2O ¬ H2CO3 ¬ HCO3- + H+ – so hyperventilation reduces H+ Effects of Hydrogen Ions Respiratory alkalosis (pH > 7.4) – hypocapnia: PCO2 < 35 mmHg – Induces Hypoventilation (to CO2), reaction is to the right: CO2 + H2O ® H2CO3 ® HCO3- + H+ – H+ lowers pH to normal pH imbalances can have metabolic causes – Ex: uncontrolled diabetes mellitus ketoacidosis, may be compensated for by Kussmaul respirations 22-16 Chemoreceptors Central – in medulla primarily monitor pH of CSF [H+] stimulates ventilation ¯ [H+] inhibits it Peripheral – found in major blood vessels aortic bodies – signals medulla via C.N. X carotid bodies – signals medulla by C.N. IX 22-17 Ventilatory responses 80% mediated by central chemoreceptors 20% mediated by peripheral chemoreceptors peripheral responses are more rapid (but weaker) 22-18 Peripheral Chemoreceptors – Located in the carotid bodies at the bifurcation of the common carotid arteries, and in the aortic bodies above the aortic arch Contain glomus cells - sites of chemoreception modulate neurotransmitter release by physiological and chemical stimuli – Peripheral respond mainly to ¯ O2 & pH; ( CO2) – Central receptors respond mainly to Δ PCO2 22-19 Central receptors monitor CSF The normal pH of CSF is 7.33 has much less buffering capacity than blood ∴ greater change in PCO2 = greater change in pH Compensatory mechanism is active transport of HCO3- into the CSF – Example: A patient with chronic lung disease will have CO2 retention, but may have a near normal CSF pH and a resulting low ventilation for his or her PCO2 level 22-20 Hypoxic Drive With severe lung disease, the hypoxic drive becomes the main stimulus for ventilation – In these pts., the kidneys have already metabolically compensated for the respiratory acidosis, so the peripheral chemoreceptors now have very little pH stimulus to increase ventilation – Under these conditions, arterial hypoxemia (PaO2) becomes the chief stimulus of ventilation If a high O2 mixture is given to relieve the hypoxemia, the ventilation may be grossly depressed 22-21 Conditions that affect ventilation Receptor afferent signals can be enhanced or suppressed – Enhanced: Metabolic acidosis Low PO2 (< 60 mmHg) Elevated temperature – Suppressed CNS depressants / narcotics Deep Anesthesia Metabolic alkalosis Cold temperatures 22-22 Integrated Responses Anesthetized patient will sometimes stop breathing for a minute or so if hyperventilated by the anesthesia provider – Decreasing arterial PCO2 normally reduces stimulus to ventilation 22-23 Drug Effects on Ventilation Amnestics – desirable for surgery, but – Suppress CNS leading to decreased respiratory rate – Affect muscle tone of upper airway – Apnea at high dosages Opioids – Affect respiratory drive center Decrease respiratory rate Mildly increases tidal volume Decreases minute ventilation over all 22-24 Drug Effects on Ventilation Volatile Anesthetics – Suppresses neuronal activation Relaxes all muscles, including diaphragm, leading to fast and shallow breathing Suppresses response to PCO2 (completely abolishes response to PO2) Nitrous Oxide – Supportive of ventilation Increases respiratory rate 22-25 Pressure and Flow Atmospheric pressure drives ventilation: 1 atm = 760 mmHg Intrapulmonary pressure and lung volume are inversely proportional – Recall Boyle’s Law Physical pressures drive conduction Partial pressures drive gas exchange Flow governed by Poiseuille’s Law Turbulent flow occurs with increased velocity, tube diameter, and gas density 22-26 Transitional Flow Transitional flow is a mixture of laminar and turbulent flow and occurs at branch points in the airways The trachea and larger airways have either turbulent or transitional airflow Laminar flow in smallest airways 22-27 Alveolar Physical Pressures (conduction) 22-28 Intrapleural Pressure Pressures are generated by the movement between the lung and chest wall The lung tends to decrease its volume due to inward elastic recoil The chest wall tends to increase its volume due to outward elastic recoil Pressure in the potential space between the parietal and visceral pleura is normally subatmospheric around -3 to -5 cm H2O (-2.2 to -3.6 mmHg) 22-29 Distribution of Alveolar Ventilation Not all alveoli are ventilated or perfused equally. Alveoli in the lower lungs (base) receive more ventilation per breath than alveoli of the upper regions of the lungs in the awake, spontaneously breathing, upright patient. The influence of gravity on a supported structure, called dependency, accounts for regional differences in alveolar ventilation & Perfusion (dependent vs. nondependent) difference in volume & compliance leads to a difference in ventilation 22-30 Perfusion There is more perfusion in the base relative to the apex (upright) This is why infarcts usually occur in the lower lobes With tissue hypoxia: – Every organ à vasodilation – Lungs à vasoconstriction (why?) to shunt blood to better ventilated areas of lung 22-31 Distribution of Ventilation & Perfusion Effective gas exchange depends on an approximately even distribution of gas (ventilation) and blood (perfusion) in all portions of the lungs (V/Q). Ventilation and perfusion depend on body position. If a standing individual assumes a supine or side-lying position, the areas of the lungs that are then most dependent become the best ventilated and perfused. Distribution of perfusion in the pulmonary circulation also is affected by alveolar pressure (gas pressure in the alveoli). The pulmonary capillary bed differs from the systemic capillary bed in that it is surrounded by gas-containing alveoli. If the gas pressure in the alveoli exceeds the blood pressure in the capillary, the capillary 22-32 West Lung Zones PA = alveolar pressure Pa = arterial pressure Pv = venous pressure Zone I: PA>Pa>Pv Zone II: Pa>PA>Pv Zone III: Pa>Pv>PA Zone I = PA, compressed arterioles = V w/o Q = deadspace (usually only seen in mechanically ventilated pts) 22-33 22-34 22-35 22-36 Lung Volumes and Capacities FRC (functional residual capacity) is the volume remaining in the lungs at the end of a normal tidal expiration. Elasticity Elasticity is the tendency of lung tissue to return to its original (or relaxed) position after an applied force has been removed. – When inflated, there is a tendency to recoil to a smaller unstressed volume – To keep the lungs inflated, an opposing pressure difference is required Provided by the chest wall and the respiratory muscles 22-38 (Compliance) 22-39 Elastic Properties With negative pressure in the thorax the lungs expand – Using spirometry, the pressure volume curves for the lung can be demonstrated during inspiration and expiration – The difference between the two curves is called hysteresis – The lung always retains some residual air, and even in forced expiration, small airway closure and trapping prevents further air loss 22-40 Dynamic Compression with Airway Closure 22-41 The Bernoulli effect accentuates collapse of airways When air enters a constriction, the linear velocity increases and pressure decreases 22-42 Flow Volume Loops ü Asthma ü COPD ü Emphysema ü Pulmonary Fibrosis ü Sarcoidosis ü Interstitial Lung Disease ü Myasthenia gravis ü ALS Compliance Chest wall compliance is the opposite of elasticity, it is a measure of the distensibility of the lung. Mathematically it is the change in lung volume divided by the change in recoil pressure, where change in volume is in liters and change in pressure is in cm H20 For mechanical vent. calculations units of pressure measured in cm H20 1 cm H20 = 98.07 Pa ≈ 0.735559 mm Hg 1 mm Hg = 1.359 cm H20 22-46 Compliance Reduced compliance is caused by: – Increased fibrous tissue (ex. pulmonary fibrosis) – Alveolar edema – Conditions that increase surface tension 22-47 Factors Affecting Airway Resistance Diameter of airway Flow (Laminar vs. Turbulent) Density of gas (viscosity) Governed by Poiseuille’s Law Age or Pathologies that affect recoil (ex. Pulmonary fibrosis) 22-48 Calculations – Minute Ventilation VE = minute ventilation (volume per minute) vT = tidal volume (volume per breath) f = frequency or RR (Respiratory Rate) Example: VE = vT x f = 500 x 10 = 5000 ml/min. 22-49 Calculations – Alveolar Minute Ventilation VA = (VT – vDS) x RR VA = alveolar ventilation VT = tidal volume VDS = physiologic dead space ~ 1ml per pound ideal body wgt. Example: 150 pound pt. VA = (VT – vDS ) x RR = (500 – 150) x 12 = 4200 ml/min. Note: anatomic ds = conducting airway mechanical ds = ventilator machine circuit, ET tube, etc alveolar ds = nonperfused alveoli (usually nominal) physiologic ds = the sum of anatomic & alveolar ds V/Q ratio Ventilation ~ 4200ml Perfusion = SV x HR ex. 70ml x 75bpm = 5250ml V/Q ratio = 4200/5250 = 0.8 = NORMAL V/Q ratio If V , then V/Q > 0.8 Q must increase to keep a normal V/Q ratio of 0.8, so perfusion increases via vasodilation. (Vasodilation) Perfusion Adjustments 22-52 Alveolar Gas Exchange Time required for gases to equilibrate = 0.25 sec RBC transit time at rest = 0.75 sec to pass through alveolar capillary RBC transit time with vigorous exercise = 0.3 sec 22-53 V/Q ratio Ventilation ~ 4200ml Perfusion = SV x HR ex. 70ml x 75bpm = 5250ml V/Q ratio = 4200/5250 = 0.8 = NORMAL V/Q ratio If Q , then V/Q < 0.8 V must increase to keep a normal V/Q ratio of 0.8, so ventilation increases via bronchodilation. Ventilation Adjustments 22-55 Increase Rate or Volume? If you want to increase alveolar ventilation, should you increase respiratory rate or tidal volume? § Increasing frequency while maintaining a constant volume results in proportional increase of both alveolar ventilation and dead space § Increasing tidal volume while maintaining constant frequency results in no change to dead space but an increase in alveolar ventilation § Answer: increasing tidal volume is more effective to increase VA than increasing frequency 22-56 22-57 Age Effects Decreased alveolar elasticity and lung compliance, respiratory muscles weaken Higher Residual Volume and decreased maximal expiratory flow rates Loss of alveolar surface area Decreased pulmonary perfusion 22-58 Pathologic Conditions Restrictive disorders (can’t get air in) – ¯ compliance and vital capacity Pulmonary Fibrosis Sarcoidosis Interstitial Lung Disease (Myasthenia gravis) (ALS) Obstructive disorders (can’t get air out) – interfere with airflow, expiration requires more effort or is less complete Asthma COPD Emphysema 22-59 Gas Exchange – Partial Pressures Alveolus Pulmonary Artery 22-60 Animation - Gas Exchange 22-61 Alveolar Partial Pressures (gas exchange) Partial pressures (as well as solubility of gas) determine rate of diffusion of each gas and gas exchange between blood and alveolus 22-63 Composition of Air Mixture of gases; each contributes its partial pressure – at sea level 1 atm. of pressure = 760 mmHg – nitrogen constitutes 78.6% of the atmosphere so PN2 = 78.6% x 760 mmHg = 597 mmHg PO2 = 159 PH2O = 3.7 PCO2 = + 0.3 PN2 + PO2 + PH2O + PCO2 = 760 mmHg 22-64 Composition of Air Partial pressures (as well as solubility of gas) – determine rate of diffusion of each gas and gas exchange between blood and alveolus Alveolar air – humidified, exchanges gases with blood, mixes with residual air – contains: PN2 = 569 PO2 = 104 PH2O = 47 PCO2 = 40 mmHg 22-65 Air-Water Interface Important for gas exchange between air in lungs and blood in capillaries Gases diffuse down their concentration gradients Henry’s law – amount of gas that dissolves in water is determined by its solubility in water and its partial pressure in air 22-66 Alveolar Gas Exchange O2 has conc. gradient CO2 has solubility 22-67 Factors Affecting Gas Exchange Membrane thickness - only 0.5 µm thick Membrane surface area - 100 ml blood in alveolar capillaries, spread over 70 m2 Ventilation-perfusion coupling - areas of good ventilation need good perfusion 22-68 Oxygen Transport 98.5% bound to hemoglobin; 1.5% dissolved Binding to hemoglobin – each of 4 heme groups bind O2 – oxyhemoglobin (HbO2 ) – deoxyhemoglobin (HHb) 22-69 Oxygen Transport Oxyhemoglobin dissociation curve – relationship between hemoglobin saturation and PO2 is not a simple linear one – after binding with O2, hemoglobin changes shape to facilitate further uptake (positive feedback cycle) 22-70 Note: 2,3-BPG = compound made by RBCs to adapt to conditions of hypoxemia, facilitating O2 release to tissues Video - Oxygen-Hgb Dissociation 22-72 Fetal Hgb Adult Hgb – α and β chains – β chain has pos. (+) charged histidine à readily binds 2,3-BPG (-) – Shifts dissociation curve to the Right Fetal Hgb Ɣ chains replace β chains - Ɣ chains neutral (0 charge) à doesn’t bind 2,3-BPG Shifts dissociation curve to the Left 22-73 Carbon Dioxide Transport – carbonic acid - 70% – carbaminohemoglobin - 23% – dissolved gas - 7% 22-74 Gas Exchange at tissues (systemic) CO2 loading – carbonic anhydrase in RBC catalyzes CO2 + H2O ® H2CO3 ® HCO3- + H+ – chloride shift keeps reaction proceeding, exchanges HCO3- for Cl- (H+ binds to hemoglobin) O2 unloading – H+ binding to HbO2 ¯ its affinity for O2 Hb arrives 97% saturated, leaves 75% saturated - venous reserve – utilization coefficient amount of oxygen Hb has released 22% 22-75 At tissues: CO2 loading O2 unloading At lung: CO2 unloading O2 loading Cl- ion shift via HCo3-/Cl- Anion exchanger 22-76 Gas Exchange at lung (alveolar) Reactions are reverse of systemic gas exchange CO2 unloading – as Hb loads O2 its affinity for H+ decreases, H+ dissociates from Hb and bind with HCO3 CO2 + H2O ¬ H2CO3 ¬ HCO3- + H+ – reverse chloride shift HCO3- diffuses back into RBC in exchange for Cl-, free CO2 generated diffuses into alveolus to be exhaled 22-77 Alveolar Gas Exchange 22-78 Non-Respiratory Functions Extraction/Metabolism of Hormones & Drugs: Amines (5-HT, NE) Peptides (Bradykinin, ANP, Endothelin) Arachadonic Acid derivatives (Prostaglandins, Leukotrienes) Purine derivatives (ATP to Adenosine) Some Local Anesthetics Diazepam Propofol Thiopental Some Opioids 22-79