Cancer and Cancer Therapies Lecture Notes PDF
Document Details
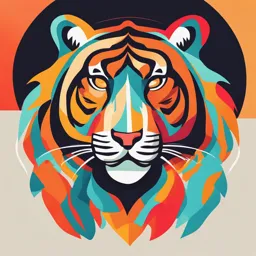
Uploaded by TriumphantOklahomaCity
Tags
Summary
This document presents lecture notes on cancer and cancer therapies. It discusses the genetic origins of cancer, focusing on somatic mutations and the role of oncogenes and tumor suppressor genes in driving uncontrolled cell growth. The material explains signal transduction pathways, highlighting the mechanisms involved in cell division regulation and how cancer can hijack these pathways. The lecture further details the hallmarks of cancer transformation, the functions of proteins like Ras, and mechanisms for cancer progression.
Full Transcript
BIOT-5260 Core Methods in Biotechnology Lecture #18 Cancer and Cancer Therapies 1 Cancer is Genetic in Origin Tumors and cancer are the result of uncontrolled cell division (balance between formation of new cells and cell destruction need to be maintained) Cancers are primarily the result of genetic...
BIOT-5260 Core Methods in Biotechnology Lecture #18 Cancer and Cancer Therapies 1 Cancer is Genetic in Origin Tumors and cancer are the result of uncontrolled cell division (balance between formation of new cells and cell destruction need to be maintained) Cancers are primarily the result of genetic defects that damage the regulatory system controlling cell growth and division. When regulatory proteins are defective, a clone of cells divides repeatedly and without regulation (tumor) until their presence interferes with the function of normal tissues– cancer In some cases, a defective gene is inherited. In other cases, the mutation occurs when an environmental exposure (a mutagen or carcinogen) or highenergy radiation interacts with the DNA of a single cell to damage it and induce a mutation. Some mutations can occur during DNA replication process. Cancer-causing agents are often called carcinogens, genetic mutation-causing agents are called mutagens (almost all carcinogens are also mutagens). Cell death Cell division 2 Cancer Is a Progressive Disease - Caused Mainly by Somatic Mutations Timing of the mutation is important! Early embryos have germline cells, which become eggs and sperm Somatic cells that make up the rest of the organism. Depending on the timing, a somatic mutation usually affects only a small portion of the entire organism (ex. spleen; shown right) Cancer occurs usually when a somatic cell mutates because of errors during DNA replication or exposure to a carcinogen. The mutation allows division in a cell that should no longer divide. The mutant cells keep dividing to form a micro-tumor. Changes in the epigenome of the tumor cell also can drive unregulated cell division 3 Cancer Progression benign tumor Malignant tumor Angiogenesis Un-controlled cell growth i. ii. Normal cell division is lost, the abnormal (mutant) cell divides to form a microtumor Mutant cancer cells emit signaling molecules to surrounding tissues, attracting vascular endothelial cells to organize into blood vessels, a process called angiogenesis. Once the microtumor contains its own blood supply, it can continue to grow into a large mass. iii. If the microtumor remains ‘local’, it is known as a benign tumor and can often be excised by a surgeon. iv. If further mutations occur, a cancer may be able to invade other tissues and form secondary tumors = malignancy. v. Malignant cancers that spread to other organs (tissues) = metastasis. These are much more difficult to treat. 4 Ref: Quail, D. F., and Joyce, J. A. (2013). Microenvironmental regulation of tumor progression and metastasis. Nature Medicine, 19, 1423–1437. doi: 10.1038/nm.3394 Normal Cell Division: The Cell Cycle The eukaryotic cell cycle has four stages: CDK1 G1 (Gap1) phase —cell growth. (G: gap between divisions) S (synthesis) phase —The DNA is replicated to produce copies for daughter cells G2 (Gap2) phase —The cell approximately doubles in size and prepares for division. M (mitosis) phase —The cell and nucleus divide. In addition, cells may exit from the growth and division cycle into a quiescent phase, the G0 phase. Most non-dividing cells are in G0. Many of CDK2 these will differentiate and rarely divide again. Cyclins/CDK Signals from cyclin D and cyclin E promote S phase entry. Signals from cyclin A triggers G2 entry, and cyclin B induces entry into mitosis. CDK4/6 CDK2 5 Cyclins and Cyclin-dependent kinases (CDK) Transitioning from one stage of cell cycle to another the cell requires permission from proteins called cyclins. The cyclins act as security checkpoints. They monitor the environment and also check to make sure that the previous stage of the cell cycle finished properly before moving on. The cyclins work in conjunction with the cyclin dependent kinases (CDKs). When the cyclin for a particular step in the cell cycle senses that conditions are correct, it binds to the appropriate CDK Addition of a phosphate, from ATP, activates the cyclin/CDK complex, which then adds phosphate groups to a series of other proteins. These are the enzymes and structural proteins that actually carry out the process of cell division. These proteins are on standby until the added phosphate group activates them. Several tumor suppressor genes (anti-oncogenes) block the action of the cyclins 6 Yang V, The Cell Cycle. 2018 7 Control of G1 to S Transition by pRB and E2F Arguably, the most important checkpoint is G1 and S transition (commitment to cell cycle). G1 Toward the end of G1, cyclin D must be activated to initiate transition into S phase. This requires binding to its partner, CDK4. Once together, ATP is hydrolyzed and a phosphate is transferred to the cyclin D/CDK4 complex. pRB is then phosphorylated, which releases E2F to transcribe genes needed to initiate DNA replication (e.g., DNA polymerase α). E2F transcription factor promotes expression of genes involved in DNA replication and also increases synthesis of the cyclins E and A. 8 G1/S checkpoint: DNA must be intact and prepared for replication p53 major DNA damage checkpoint and is related to the cell cycle If DNA damage is present p53 > p21 ≠ cyclin E/CDK2 activation 9 Growth factors How do normal cells know when to start (and stop) replicating/dividing? Cancer can hijack this pathway (and many others) MAPK cascade Phosphorylation of Jun and Fos in nucleus Jun Fos E2F (and others e.g., cyclins, CDKs) E2F Cyclins, CDKs, enzymes for DNA synthesis S phase entry 10 Receptors and Signal Transduction A wide variety of molecules are used in signaling, but in nearly all cases the recipient cell needs a receptor. Usually, the receptor is a protein found at the cytoplasmic membrane with the site for binding the messenger facing outward. When a signaling molecule (ligand) appears, it binds to the receptor, causing a conformational change. The receptor then passes the signal on to other proteins, known as signal transduction proteins. Both receptors and signal transduction proteins can associate (or disassociate) during the process of signal transmission. 11 There are 3 Main Types of Signal Transduction mechanisms 1) Phospho-transfer system 2) Second Messenger 3) Ion Channel 12 1. Phospho-transfer systems. Receptor activation causes the phosphorylation of signal transduction proteins. How does this work? First messenger (hormone, growth factor, or neurotransmitter) activates a receptor from outside the cell. Protein kinases transfer phosphate groups from ATP to other proteins. The activated receptor may act as a protein kinase itself (intrinsic protein kinase activity), or it may bind to and activate a separate protein kinase. Often several proteins take part in a phosphotransfer cascade that allows the signal to be amplified or modulated in a variety of ways *can be self phosphorylation or in separate step 13 2. Second Messengers Intracellular signaling molecules called second messengers are produced in response to an activated receptor (G protein-coupled receptors, GPCRs). First messenger (e.g., hormone, growth factor, or neurotransmitter) activates a receptor from outside the cell. The active receptor activates a guanosine nucleotide-binding protein (G protein), which activates a membrane-bound effector enzyme, which in turn synthesizes the second messenger. 14 GPCRs 15 3. Ion Channel Activation The receptor itself acts as an ion channel Binding of a signal molecule to an ion channel induces a conformational change that opens the channel. Ions may now pass through the open channel freely 16 Ultimately, ‘outside-in’ signaling triggers transcription 17 Anatomy of a Transmembrane Receptor (RTK) Growth factors are bound by specific cell surface receptors Growth factor receptors have 3 domains: the extracellular binding site, the transmembrane region, and the intracellular domain (often a tyrosine kinase domain). Phosphorylate Tyr residue 18 Activation of the GPCR Typically, the protein kinase activates a G protein of the Ras family, and this in turn stimulates a phosphortransfer cascade. This cascade consists of 3 mitogen-activated protein (MAP) kinases. MAP kinase kinase kinase (MAPKKK) activates MAP kinase kinase (MAPKK), which activates MAP kinase (MAPK). Finally MAPK phosphorylates transcription factors that activate genes needed early in cell division (e.g., cyclin D). Ras (G protein) Raf (MAPKKK) MEK (MAPKK) ERK (MAPK) Phosphorylation of nuclear transcription factor (such as Elk-1) = transcriptional activation 19 https://www.youtube.com/watch?v=oDjDUUhGVsI 20 Mutations in two main types of genes can cause cancer: oncogenes and tumor suppressor genes. Oncogenes: cancer-causing genes (e.g. mutated gene encoding signaling proteins involved in cell cycle regulation). Mutations in oncogenes are dominant. A mutation in one allele is sufficient to give cancer-causing effect; wt copy cannot suppress the mutant. Proto-oncogenes: turn into oncogenes after a mutation makes the protein more active, the gene is duplicated, or a mutation in the promoter increases the expression of the protein. Oncogenes are mutant alleles of proto-oncogenes. Tumor-suppressor genes: restrain cell division. Both copies must be inactivated. If one copy is mutated (recessive) the cell becomes vulnerable to transformation. In some cases, mutations in tumor suppressor genes can function as “dominant negative”, e.g., mutant p53 protein can prevent normal function of un-mutated p53. 21 Hallmarks of Oncogenic Transformation (in vitro) In culture, normal cells grow until they touch each other cells on all sides; then they stop dividing (contact inhibition). If normal cells are transfected with an oncogene, they can be transformed into cancer cells. These cells continue to divide and aggregate and no longer respond to contact inhibition. 22 How can we determine if an activation of an oncogene has resulted in transformation? When transformed cells are injected into an experimental animal (in vivo; i.e. mouse), a tumor forms 23 Ren T, et al. Biochemical and Biophysical Research Communications Volume 364, Issue 4, 28 To Reiterate: Steps Necessary to Promote Cell Growth and Division The execution of a cell growth signal involves a variety of steps. First, the signal to ‘grow’ involves the production of growth factors (GF). This initiates the signal transduction pathway GFs (or hormones) bind and activate a cell surface receptor, which in turn activates a variety of intracellular proteins that transmit the signal from the cell membrane to the nucleus. Inside the nucleus, a transcription factor activates genes necessary for cell division and growth 24 Function of Ras Protein When a cell surface receptor receives a signal to grow, it transmits the signal to Ras (G protein). Activated Ras binds GTP and sends the growth signal to the nucleus. After the signal has been sent, GTP is hydrolyzed to GDP, and Ras becomes inactive once again ras mutations found in about 25% of cancers Mutations Creating the ras Oncogene Are Highly Specific The transition from the normal proto-oncogene to the ras oncogene involves a single amino acid substitution at amino acids 12, 13, or 61. This amino acid change prevents the hydrolysis of GTP by Ras; therefore, the protein is always in its signal-emitting 25 ON mode. myc Oncogene transcription factor that switches on several other genes needed for cell division. Mutations in myc are among the most common in mammalian cancers. Normally, the gene for Myc is present in two copies. Aberrant chromosomal replication can result in myc duplication Once this occurs, the number of myc oncogenes grows exponentially (50-100 copies possible). Since all these copies are expressed, the amount of Myc protein increases and cancer results. Alternatively, Overproduction of Myc by Changing Promoters Chromosomal translocation (8 & 14)of the c-myc oncogene may enhance the amount of protein by fusing the structural portion of the gene to a highly 26 active promoter. This is seen in Burkitt’s Lymphoma The diversity of myc’s role Llombart V, Mansour MR. EBioMedicine. 2022 PMID: 34942444; PMCID: PMC8713111. 27 Myc’s 3D structure Llombart V, Mansour MR. EBioMedicine. 2022 PMID: 34942444; PMCID: PMC8713111. 28 Indirectly targeting myc Llombart V, Mansour MR. EBioMedicine. 2022 PMID: 34942444; PMCID: PMC8713111. 29 2 ways for this to occur Mutations in Tumor-Suppressor Genes Are Recessive Tumor suppressors block cell division. Examples of tumor-suppressor genes: pRb, p53 and p21 Normally, 2 wt copies are present. In most cases, mutation in both alleles must occur to completely inactivate the antioncogene (p53 is an exception). Tumor suppressor proteins should bind to signal transmission proteins and prevent them from activating transcription factors. 30 Mixed Tetramers of p53 Protein Explains why p53 is Dominant Negative Mutations in the p53 gene are detrimental whether one or both copies are mutated. p53 protein works as a tetramer, so even if only one of the alleles has a mutation, most of the tetramers are defective. p53 functions correctly only if all four subunits of the tetramer are normal. 31 Central Role of p53 Tumor Suppressor Gene The role of p53 is to halt cell division or trigger cell death during circumstances like lack of oxygen, DNA damage, and low nucleotide pools. Activated p53 affects many different targets. If there is severe damage, p53 activates Bax protein and initiates programmed cell death. Otherwise, p53 activates cell cycle arrest by turning on p21, which in turn blocks the cyclins. Active p53 can also block synthesis by DNA polymerase through the action of GADD45. Active p53 also binds to growth factor receptors to block any further growth signals p53 mutations are dominant negatives. Well over half of all human cancers are defective in p53 32 p53 and p21 Block the Cell Cycle When a cell senses DNA damage, the p53 protein forms active tetramers that bind to the control region of the p21 gene. p53 stimulates the transcription and translation of p21 protein. The p21 protein then binds to and inactivates multiple cyclins, preventing progression of the cell cycle. p16 is also a tumor suppressor but functions differently from p21 and can only bind cyclin E (S-phase). 33 34 Formation of a Tumor Requires Several Somatic Mutations The generation of a malignant tumor requires several steps. In practice, multiple somatic mutations are necessary for the production of most cancers. For example, many colon cancers carry the following defects: 1. Inactivation of the APC anti-oncogene (both copies). APC: adenomatous polyposis coli 2. Activation of the Ras oncogene 3. Inactivation of the DCC tumor suppressor gene (both copies). 4. Mutation of a single copy of the p53 gene Formation of Metastases Requires Additional Mutations: 1. Loss of adhesion to neighboring cells in the original tumor (via APC) 2. Ability to digest the membranes surrounding other tissues 3. Vascularization of the tumor, which not only provides nutrients but also allows mobile cancer cells access to the circulatory system 35 Inherited Defective Tumor Suppressors & Susceptibility to Cancer In this case, every somatic cell starts its life with one faulty copy, and only a single somatic mutation is needed to completely inactivate the pair of anti-oncogenes. Mutations in mutator genes (genes involved in DNA synthesis and repair) affect the rate at which further mutations occur during cell division. For example, inheriting a single defective copy of either the BRCA1 or BRCA2 genes (breast cancer A genes) predisposes women to breast cancer. BRCA1 and BRCA2 proteins are involved in DNA repair. The presence of a double stranded DNA break triggers the phosphorylation of BRCA1 via the ATM protein. BRCA2 plus RAD51 then bind to phospho-BRCA1 and take part in the DNA repair process. A potential therapeutic strategy is to inhibit other DNA repair enzymes in the breast cancer cells and they would rapidly accumulate DNA damage, leading to cell death and apoptosis. ATM (ataxia telangiectasia mutated serine-protein kinase) 36 DNA Repair Needs PARP or BRCA1/2 Poly (ADP-ribose) Polymerase (PARP) is needed to mend single-strand DNA breaks. Inhibition of PARP results in increased damage and the emergence of double stranded DNA breaks. These could be mended if BRCA1/BRCA2 were functional. But if BRCA1 or BRCA2 is defective, the cell is doomed. 37 Anti-Cancer Treatment Chemotherapy Designed to kill rapidly dividing cells in the body Designed to target cells overexpressing protein kinases Immunotherapy: use body’s own immune system to flight cancer, which involves activation of immune cells and gets them to recognize cancer cells Monoclonal antibody Immune-checkpoint blockade (e.g., PD-1, PDL-1) Adoptive cell transfer (e.g., CAR T cells) 38 Ref: Wali, Sunila. (2017). Exploring the Alternate Approach for Cancer Treatment in Delhi. Retrieved from https://eurasiantimes.com/cancer-treatment/ 5-Fluorouracil (Adrucil): Thymidylate Synthase Inhibitor Commonly used chemotherapeutic agent for cancer treatment Uracil analogue Inhibition of Thymidylate Synthase (TS) TS catalyzes the methylation reaction of deoxyuridine monophosphate (dUMP) to make deoxythymidine monophosphate (dTMP). This reaction provides thymidylate necessary for DNA replication and repair. The 5-FU metabolite FdUMP binds to nucleotidebinding site of TS and blocks binding of normal substrate dUMP Fluorine at C5 5-FU Thymine Uracil 39 Targeted Cancer Treatment – ‘out of the dark ages’ Chemotherapeutic drugs can kill rapidly dividing cells in the body. Not specific to cancer cells, so other dividing cells also killed. Ex. decarbazine, 5-azacitidine Targeted therapies attack cells that have specific markers unique to cancer cells or cells that support the cancer cells. Targeted therapies work by: -Blocking or stopping cellular signals -Modifying proteins in cancer cells -Preventing vascularization of tumors – bevacizumab (Folkman) -Activate or direct immune cells to target cancer cells -Deliver toxins to the cancer cells Some drugs are more “targeted” than others and some function using more than one method Often used in combination with other types of therapy – surgery, chemo or radiation. What do Targeted Therapies do? Hormone – Slow or stop tumors that require specific hormones to grow by preventing expression or blocking activity. ex. estrogen receptor Signal transduction inhibitors – Blocks cells from sending or receiving biochemical signals to grow or divide. Gene expression modulators – Modify proteins that regulate cellular functions. Apoptosis inducers – Cause cancer cells to enter programmed cell death. Angiogenesis inhibitors – Block the growth of blood vessels that supply tumors with oxygen and nutrition. Immunotherapies – Trigger the immune system to kill the cancer cells (CAR–T; Adoptive cell therapy). Antibodies that deliver toxins – The antibodies recognize a specific cell surface protein on the cancer cell and deliver a toxic molecule only to the cancer cell (silver bullet). Cancer vaccines – Deliver cancer cell specific antigens to produce an immune response. Gene Therapy – Replace tumor suppressor gene, increase sensitivity to treatment, introduce “suicide” genes. Protein Kinase Inhibitors as Cancer Therapeutics Many oncogenes and tumor suppressor genes encode protein kinases or proteins that act in pathways upstream from protein kinases (e.g., CDKIs, VEGFR) The simplest protein kinase inhibitors are ATP analogs that occupy the ATP-binding site but cannot serve as phosphoryl group donors (enzyme stays in inactive form). These inhibitors lack selectivity; they inhibit other protein kinases. Imatinib (Gleevec®) More selectivity is seen with compounds that fill part of the ATP-binding site but also interact with other part of the protein unique to the target protein kinase. Imatinib mesylate (generic name; brand name: Gleevec®), a small molecule inhibitor, binds to oncogenic Abl kinase active site (it occupies both the ATP-binding site and other part of Abl). It is effective in treating early stage chronic myeloid leukemia (CML). 42 Some FDA-Approved Targeted Cancer Molecules Agent Target bevacizumab humanized monoclonal antibody with a circulatory system target (VEGF-A) cetuximab chimeric monoclonal antibody with a tumor target (EGFR) ipilimumab fully human antibody with an immune system target (CTLA-4) bortezomib small molecule proteasome inhibitor imatinib small molecule tyrosine kinase inhibitor seliciclib small molecule cyclin-dependent kinase inhibitor Ado-trastuzumab emtansine (Kadcyla) HER2 (ERBB2/neu) Breast cancer (HER2+) Afatinib (Gilotrif) EGFR (HER1/ERBB1), HER2 (ERBB2/neu) Non-small cell lung cancer (with EGFR exon 19 deletions or exon 21 substitution (L858R) mutations) Bevacizumab (Avastin) VEGF ligand Various Cancers EGFR (HER1/ERBB1) Colorectal cancer (KRAS wt) Squamous cell cancer of the head and neck Cetuximab (Erbitux) Indication Monoclonal antibodies that bind extracellular portions of specific protein kinases can eliminate the receptor’s kinase activity by preventing dimerization or by causing their removal from the cell surface. In some cases, an antibody selectively binding to the surface of cancer cells could cause the immune system to attack those cells Trastuzumab (Herceptin®), cetuximab (Erbitux®), and bevacizumab (Avastin®) are monoclonal antibodies that target HER2/neu, EGFR and VEGFR, respectively. Herceptin® was the first monoclonal antibody used to treat cancer. Herceptin® binds Her2 receptor and flags the receptor to be destroyed by immune system. It also blocks receptor activation. Avastin® binds to vascular endothelial growth factor and inhibits its binding to receptor and the development of blood vessels in tumors. Erbitux® inhibits EGFR by competing with EGF for the binding site on the receptor, preventing receptor dimerization and activation. It slows tumor growth and metastasis. 44 Immune checkpoint inhibitors Cancer cells express PDL1 (programmed death ligand-1) which binds PD1 receptor on T cells and inhibits T cell activation. This leads to reduced T cell expansion, reduced cytokine expression and decreased T-cell induced cytotoxicity. Anti-PD-1 antibody prevents the PDL1 and PD1 interaction. PD-1 Antibodies: Pembrolizumab (Keytruda®) and Nivolumab (Opdivo®) CTLA-4 inhibitor: Ipilimumab (Yervoy®) 45 46 References: Clark, DP., Pazdernik, NJ. (2016). Biotechnology. Amsterdam [u.a.]:Academic Cell. Nelson, DL., Cox, MM. (2013). Lehninger Principles of Biochemistry. New York: W.H. Freeman and Company. 47 Supplementary Materials (Not on Exam) 48 Supplementary Videos and Links The MAP Kinase (MAPK) signalling pathway https://www.youtube.com/watch?v=npnLnzsWYFg How do tumor cells evade the immune response? And what can be done to defeat escape mechanisms: https://www.youtube.com/watch?v=K09xzIQ8zsg Herceptin: A Multimodal Approach Targeting HER2 Positive Breast Cancer https://www.youtube.com/watch?v=48VSU4AZ-L0 Cancer Treatment: How it was in 2013: https://www.youtube.com/watch?v=Ayi1-OH10HA How does Erbitux work? https://www.youtube.com/watch?v=33zPCz1yVxY Tumor angiogenesis, and how Avastin (Bevacizumab) works: https://www.youtube.com/watch?v=3xmlYr1AGx8 How our immune systems can make us immune to cancer: https://www.youtube.com/watch?v=3kiDfLS0UXQ How does Opdivo (nivolumab) work? https://www.youtube.com/watch?v=s6k8b-SWbfE Battling Cancer by Using Epigenetics: https://www.youtube.com/watch?v=xQH6mcmBRqk 49 Ref: Wikipedia (2017). Schematic of GEF Activation of a GTPase. Retrieved from https://en.wikipedia.org/wiki/Guanine_nucleotide_exchange_factor 50 MicroRNA Regulation of Transcription and Translation Schematic representation of the microRNA generation and silencing mechanisms. Hairpin-forming pre-miRNAs are generated by pri-miRNAs, which is cleaved by Drosha. Later, premiRNAs are transported into the cytoplasm by exportin-5 and further converted into double-stranded mature miRNAs by Dicer. Mature miRNAs are incorporated into the RISC complex, unwound and annealed to the target mRNAs carrying complementary sequences. miRNAs are able to regulate tens to hundreds of mRNAs via the imperfect base pairing between miRNAs and the 3′ or 5′ untranslated region of the target mRNAs. The miRNA-mRNA interaction silences the target genes through mRNA cleavage or translational inhibition 51 miR-21 Regulatory Network Mutations affecting cancer also occur in regulatory RNA like microRNA. The microRNA, miR-21, regulates an unusually high number of other genes, a small selection of which are shown here. miR-21 regulates genes in green rectangles by inhibiting translation of their mRNA. Purple pentagons represent protein translation factors that are directly inhibited. Note the feedback loop by which miR-21 promotes its own synthesis by inhibiting PDCD4, which inhibits the translation factor AP1, which in turn stimulates the synthesis of the precursor to miR-21. The net result of oncogenic mir-21 overexpression is the promotion of metastatic cancer cell growth. Black lines ending in circles = inhibition; green arrows = activation 52 GENERAL STRATEGIES FOR ANTI-RAS DRUG DEVELOPMENT. (i) Molecules that directly bind RAS disrupt its interaction with guanine nucleotide exchange factors or with effectors such as the RAF serine threonine kinases. (ii) Also shown are four indirect approaches that target (ii) proteins modulating RAS spatial organization and association with the plasma membrane (e.g., farnesyltransferase and PDEd), (iii) RAS effector signaling (e.g., RAF and PI3K), (iv) synthetic lethal interactors of mutant RAS, and (v) RAS regulated metabolic processes in cancer cells. 53 (iii) The problem with these approaches is that they may affect normal cells. Converting the 3D structure of mutated KRAS to the wild-type conformation Mutations at codons 12, 13 , or 61 change the amino acids at these sites to modify the 3 dimensional structure of KRAS such that the mutated protein holds on to GDP and is always activated. Dr. Sona Vasudevan has analyzed the 3D structure of these mutated KRAS proteins and has found a new “pocket” that exists only in the mutated proteins. She has designed a small molecule that could bind to this pocket and cause the protein to shift its 3D structure to the wild-type configuration. If this works, then in the presence of the drug, mutated KRAS would now revert to the wild-type configuration, release GDP, and turn itself off. This strategy would not effect normal cells because they have the wild-type KRAS. Work is in progress in my lab to get preliminary data to prove this hypothesis. If it works, we will apply for a $100 million grant from the NIH. 54 Fig. 1. Current and emerging epigenetic therapies. Chromatin exists in two major states: an open relaxed conformation called EUCHROMATIN, within which most transcriptionally active genes reside, and a more condensed compact state called HETEROCHROMATIN, which is largely transcriptionally silent. The dynamic transition between these states is mediated by chromatin modifications such as methylation and acetylation, which are laid down by epigenetic writers, bound by epigenetic readers, and removed by epigenetic erasers. Many epigenetic proteins have more than one functional domain, allowing them to function as epigenetic readers and writers or epigenetic readers and erasers. A growing number of small molecule drugs are being developed to target these epigenetic regulators. Highlighted in red are the targets for epigenetic therapies that are either in routine clinical use or currently being evaluated in clinical trials. IDH1 and IDH2 are marked with asterisks because, although they are not epigenetic proteins, mutations in these proteins profoundly affect epigenetic erasers of DNA methylation. Inhibitors of IDH1 and IDH2 reduce levels of the oncometabolite 2HG and alleviate the inhibition of these epigenetic erasers. 55 EPIGENETIC THERAPIES AIM TO RESET THE CANCER EPIGENOME The most clinically advanced epigenetic therapies in oncology are DNA hypomethylating agents [DNA methyltransferase inhibitors (DNMTi)] and histone deacetylase inhibitors (HDACi). Global alterations in DNA methylation occur in a broad range of cancers and are associated with repression of tumor suppressor genes. An emerging theme in epigenetic cancer therapies: altering the epigenome to enhance immunity with the idea of eliminating host tolerance to its own tumor cells A rapidly increasing body of evidence demonstrates the interdependence of cancer epigenetics and cancer immunology. Epigenetic therapies induce an immunological response that contributes to their efficacy, and accumulating data also demonstrate that epigenetic therapies potentiate the effects of adoptive immunotherapies and immune checkpoint inhibitors. The next frontier—combinations of immunotherapies and epigenetic therapies—is set for clinical evaluation. 56 The Cancer Epigenome Dawson, Science 355, 1147–1152 (2017) 17 March 2017 Chromatin (epigenetic) modifications play an important role in regulating all DNA-templated processes, including transcription, repair, and replication All of these chemical epigenetic modifications are dynamic; they are laid down by “epigenetic writers” and removed by “epigenetic erasers” in a highly regulated manner Mutations in epigenetic writers, readers, and erasers as well as members of chromatin-remodeling complexes are pervasive in cancer 57 Antibody drug conjugates (ADCs) constitute a family of cancer therapeutics designed to preferentially direct a cytotoxic drug to cells expressing a tumor-specific cell-surface antigen recognized by an antibody 58 59 Some Anti-Cancer-Drug Conjugates 60 POSSIBLE MECHANISMS OF ANTIBODY-DRUG CONJUGATE (ADC) TOXICITY. Normal cells expressing target uptake the ADC (upper left); free drug released from ADC in circulation diffuses into normal cells (upper right); nonspecific uptake of ADC by normal cells (lower left); free drug released from cells that uptake ADC, specifically or nonspecifically, diffuses into normal cells (lower right) 61 In spite of possible offtarget effects, antibodydrug conjugates are being tested in several clinical trials 62 GENERALIZED MECHANISM OF ACTION OF ANTIBODYDRUG CONJUGATES (ADCs). a. The ADC binds to the antigen on the target cell. b. The antigen-ADC complex is internalized through receptor-mediated endocytosis and, in most cases, is transported from early endosomes to lysosomes. c. In the lysosome, internal conditions may destabilize the linker or mAb backbone, causing the cytotoxic component to dissociate and circulate freely in the cytoplasm. d. Most cytotoxic components of current ADCs either bind to the minor groove of DNA and induce strand breakage as in (d ), e. or bind to tubulin, resulting in microtubule disruption (e). f. Both processes lead to apoptosis (f). 63 ADOPTIVE T-CELL THERAPY FOR CANCER Christian S. Hinrichs, Steven A. Rosenberg, Immunological Reviews 2014, Vol. 257: 56–71 The complete and durable responses to T-cell Infiltrating Lymphocytes (TIL) therapy have established the principle that infusion of tumor-specific T cells can eradicate advanced cancer in humans. T cells reactive against the neoepitopes of mutated gene products might be generated on an individualized basis for administration to patients. A. A metastatic tumor deposit is resected. The expressed genes of the tumor are sequenced to identify mutations, and antigen processing and major histocompatibility (MHC) binding algorithms are used to predict potential peptide targets spanning these mutations. Simultaneously, T cells from the tumor (both tumor-reactive and non-tumor-reactive) are grown in culture, and those with reactivity against mutated peptides are isolated using fluorescence cell-sorting. The mutated peptide-reactive cells are identified for sorting either by their binding to MHC-peptide multimers or by their expression of activation markers (e.g. 4-1BB) upon coculture with antigen presenting cells (APC) loaded with mutated peptides. 64 B. Tumor-reactive T cells can be isolated directly from resected tumors by generating a single-cell suspension of tumor with its infiltrating T cells. T cells with reactivity against tumor cells can be identified and isolated using cell sorting based on binding to fluorescently labeled antibodies against 4-1BB or other markers. 65 C. As in panel B, tumor-reactive T cells can be identified by their upregulation of 4-1BB or other markers. Based on expression of these markers, single-cell flow sorting can be performed to isolate individual tumor-reactive cells. The TCR genes from these cells can be amplified and sequenced, and expression vectors encoding the TCR alpha and beta chains can be synthesized. These expression vectors can be used to genetically engineer open repertoire T cells that posses an endogenous TCR (gray) to express the tumor-reactive TCR (green and purple). 66 GENE-ENGINEERED T CELLS FOR CANCER THERAPY Michael H. Kershaw, Jennifer A. Westwood and Phillip K. Darcy, Nature Reviews/Cancer, Vol 13, 2013 T cells have the capacity to eradicate diseased cells, but tumors present considerable challenges that render T cells ineffectual. Cancer cells often make themselves almost ‘invisible’ to the immune system, and they sculpt a microenvironment that suppresses T cell activity, survival and migration. Genetic engineering of T cells can be used therapeutically to overcome these challenges. T cells can be taken from the blood of cancer patients and then modified with genes encoding receptors that recognize cancer-specific antigens. Additional genes can be used to enable resistance to immunosuppression, to extend survival and to facilitate the penetration of engineered T cells into tumors. Using genetic modification, highly active, self-propagating ‘slayers’ of cancer cells 67 can be generated. Key points The abilities of T cells to coordinate immunity and to deliver lethal hits against diseased cells can be directed towards tumors by genetically modifying T cells. Genes encoding specific antigen receptors can be inserted into T cells to enable them to recognize and respond to cancer cells. Migration of T cells towards tumors can be facilitated by the expression of specific chemokine receptors in T cells. Genetic modification of T cells can enhance their proliferation and survival, leading to a sustained attack on tumors. Resistance to tumor-derived immunosuppressive factors can be provided genetically to T cells so that they can maintain their activity in a hostile environment. Genes that confer sensitivity to drugs can be used to enable the elimination of T cells if they exert toxicity against vital normal tissues. 68 69 70 Other anti-cancer drug targets DNA topoisomerases: these enzymes take part in uncoiling DNA during chromosome replication. Inhibitors include etoposide and daurorubicin Microtubules of the mitotic spindle: these are vital for cell division and chromosome partition. Inhibitors include colchicine and taxane Cyclin-dependent kinases: these play a major role in regulating the cell cycle. Inhibitors include alvocidib and seliciclib 71 BCHB-526 Core Methods in Biotechnology Lecture 19 Molecular Forensics 1 Conflict of Interest Conflict of Interest Disclosure I have no actual or potential conflicts of interest. I do not have any relationships with companies that manufacture medical devices, pharmaceuticals, biologics, or other companies producing FDA-regulated products. The Genetic Basis of Identity Originally, the word “forensics” referred to debating evidence in a courtroom. It derives from the Latin word forensic, referring to the forum, the public area where meetings and trials were held. Forensic science (or forensic studies) is the use of science in gathering evidence. The term forensics has been used to mean the same as forensic science. Phenotypes can be used for identification. Fingerprints and hair analyses are the class examples of phenotypes used in law enforcement. Retinal scans also provides a form of unique identification, taking advantage of the unique pattern of blood vessels on the retina at the back of the eyes. Most physical differences between people are due to complex interactions of several genes during development. DNA technology has many practical uses. Because every individual has a unique DNA sequence, DNA samples can be used for identification. 3 Blood Typing can be used to establish identity Body tissues and fluids may be used to establish identity. The membranes of red blood cells contain several proteins and lipids with attached carbohydrate portions that are exposed on the outside of the cell. These are highly antigenic and in blood typing, they are referred to as blood antigens. Antigenic glycolipids determine each individual’s blood type (ABO blood group system). Three different glycolipids, A, B and O, are involved. They consist of different carbohydrate structures attached to the same lipid. The A antigen is made by adding N-acetyl-galactosamine to the end of the O antigen. The B antigen is made by adding galactose to the end of the O antigen. Antibodies are made against the A and B antigens, but the shorter O antigen is poorly antigenic and invokes little antibody production. NAGal, N-acetyl-galactosamine; GAL, galactose; NAGLc, N-acetyl-glucosamine; FUC, fucose; GLC, glucose4 3 alleles-A, B and O- are present in the population. Because we all have 2 copies of each gene, we all have 2 alleles for the ABO system. The A or B alleles are both dominant; therefore, if an allele for either A or B is present, that antigen will be expressed. A person with one A and one B allele will express both antigens on his or her blood cell surfaces (AB blood group). Antibodies are not made against endogenous antigens present on their own red blood cells. Each individual makes antibodies against exogenous (foreign) antigens (e.g., people with type A blood express antibodies against the B antigen). People with type O blood express antibodies to both A and B antigens, and those with type AB have neither antibody. ABO gene encodes for glycosyltransferase. 5 If a person is given blood of the wrong type, that person’s antibodies cause the foreign blood cells to clump together or agglutinate. People with type AB blood are considered universal acceptors because they do not react against any type of blood. Conversely, type O people are universal donors but can only accept type O themselves because they have antibodies to both the A and B antigens. 6 Because humans are diploid, a child may belong to a different blood type than either of his or her parents. For example, the AO mother will make A antigen, and a BO father will make B antigen. Despite this, these parents can have a type O child because the child may inherit the O gene on one chromosome from the heterozygous mother and another O gene from the heterozygous father. 7 DNA Fingerprinting Blood typing was once the primary forensic evidence used in many criminal cases. During the 1980s, the use of DNA in forensics became established. Today DNA evidence is regarded as superior to virtually every other type of forensic evidence in establishing identity. DNA extracted from tissue samples taken from suspects are compared with evidence obtained from the crime scene. Several types of analysis may be used to determine whether DNA found at the scene of a crime matches that of the suspect or the victim. The most widespread is known as DNA fingerprinting. DNA fingerprinting relies on the unique pattern made by a series of DNA fragments after separating them according to length by gel electrophoresis. Ref: Blood and DNA. DNA Profiling. Derived from https://bloodanddna.weebly.com/dna-profiling.html 8 RFLPs (Restriction Fragment Length Polymorphisms) The first generation of DNA fingerprinting used restriction enzymes to generate the variation in DNA fragment size between individuals. Variations in the DNA base sequence of restriction enzyme recognition sites result in differences in the size of the fragments. Such sequence differences are called RFLPs. The steps involved in RFLP-based DNA fingerprinting are as follows: 1. The DNA is cut with a restriction enzyme 2. The DNA fragments are separated by length by gel electrophoresis 3. The fragments are visualized by Southern blotting. The separated fragments are transferred from the gel to nylon paper. Then a radioactively labeled DNA probe is added. The probe binds to those DNA fragments with complementary sequences. 10 RFLPs (Restriction Fragment Length Polymorphisms) The steps involved in RFLP-based DNA fingerprinting are as follows: 1. The DNA is cut with a restriction enzyme 2. The DNA fragments are separated by length by gel electrophoresis 3. The fragments are visualized by Southern blotting. The separated fragments are transferred from the gel to nylon paper. Then a radioactively labeled DNA probe is added. The probe binds to those DNA fragments with complementary sequences. 4. The blot is covered with radiation-sensitive film to give an autoradiograph. This shows the location of those DNA fragments that reacted with the radioactive probe. 11 RFLPs (Restriction Fragment Length Polymorphisms) The final product of a DNA fingerprint is an autoradiograph that contains at least 5 essential lanes. The markers are standardized DNA fragments of known size, which have been radioactively labeled. They help determine the size of the various fragments. The positive control (TS) is DNA from a source known to react positively and reliably to the DNA probes and shows whether the test has worked as expected. The experimental lanes have samples from the victim, the defendant, and the crime scene. In this example, the blood from the defendant’s clothing was compared with his own blood and the victim’s blood. The DNA from the clothing actually matches that of the victim. 12 PCR plus Dot Blots for Identification In addition to preparing DNA for fingerprinting, PCR may be used to generate DNA segments for direct comparison by DNA sequencing or hybridization. PCR is especially useful for amplifying small regions of DNA with high person-to-person variability. In this approach, DNA from a forensic sample is amplified by PCR and compared with DNA from the suspect. For hybridization, spots of DNA samples are bound to a membrane and tested for binding a DNA probe that is tagged with a fluorescent dye (radioactive probes were used in early work). In such dot blots, the probe either binds or doesn’t bind, so any spot is either positive or negative. Alternatively, segments of DNA that have been amplified by PCR can be fully sequenced. 13 Using Repeated Sequences in DNA Fingerprinting A variation of DNA fingerprinting is to look at regions of the DNA that contain variable number tandem repeats (VNTRs), sequences of DNA repeated multiple times and different people have different numbers of repeats. VNTRs usually occur in noncoding regions of DNA. Repeat sequences vary greatly in length. For forensic purpose, relatively short repeated sequences are now generally used and are known as short tandem repeats (STRs) or satellite DNA (they are more likely to be intact in low-quality samples often recovered from crime scenes). Originally, restriction enzymes were used to cut out the DNA segment containing the VNTR. Nowadays, DNA fragments for VNTR analysis are generated by PCR. The DNA bands are separated by gel electrophoresis and visualized by Southern Blotting. Picture below shows corresponding DNA fragments from 3 individuals who differ in the number of repeats in the same VNTR. Consequently, the length of the fragment differs from person to person. 14 Using Repeated Sequences in DNA Fingerprinting The STR is a subcategory of VNTR in which the repeat is from 2-6 nucleotides long. Most STRs are not as variable in the number of repeats as VNTRs with longer unit sequences. However, many STR loci are available, and if several are analyzed simultaneously, this will provide enough data that the pattern would be unique for each individual. It is possible to analyze several STR loci simultaneously by running multiple PCR reactions in the same tube using different primers (multiplex PCR). 3 different STR loci are amplified using PCR primers. Each set of primers is labeled with a different fluorescent label to distinguish each locus from the other. The PCR products are run on an agarose gel to determine the length of the fragment and therefore the number of repeats. This individual is heterozygous for each locus because there are 2 different sized bands for each PCR primer set. Multiplex STR analysis is the basis of the national database in countries like United Kingdom and the United States. 15 Advances in DNA Fingerprinting Commercial kits are available for identification of up to 15 STR loci plus a sex marker at once Capillary electrophoresis can detect multiple fluorescently-labeled primers with non-overlapping primer sets. Each dye emits at a different maximum wavelength. In order of shortest to longest, these are: Blue = 6-FAM; Green = VIC; Black = NED; Red = PET A male who is heterozygous for all STR identified by the kit will have 32 peaks – amelogenin (AMEL in red) is a sex marker where one peak at 106nt indicates an individual with only an X chromosome, and a peak at 106nt and at 112nt indicates an individual with an X and a Y chromosome Very widely used in forensics, paternity testing, and monitoring bone marrow engraftment status 16 Advances in DNA Fingerprinting Each STR has a known location on a specific chromosome All except amelogenin are tetranucleotide repeat STR sequences For paternity exclusion, 99.9987625% average For identity: 3.583 x 10-18 average probability that two individuals will have identical genotypes Separation by size: the farther to the right, the larger the sequence Informative alleles – for BMT patients – are those which are not present in the donor prior to transplant, and are not in a “stutter” down (~4 bp) position 17 Single Nucleotide Polymorphism Typing Technology An SNP is formed by a point mutation, where one base pair is substituted by another base pair. Per definition, a genetic variation at a single base pair locus is not considered to be an SNP unless at least 2 alleles have frequencies of more than 1% in a large, random population. The human genome consists of approximately 10 million SNPs (~one SNP per 300 base pairs). Single base extension (SBE) is a method developed for SNP genotyping. The SBE reaction is performed as consecutive cycles of denaturation of double-stranded DNA, annealing of the SBE primers to the PCR products, and single base extension. 18 Single Nucleotide Polymorphism Typing Technology The SBE primer anneals to the single-stranded PCR product immediately upstream of the SNP position, and the DNA polymerase adds a fluorescently labelled di-dioxyribonucleotide, complementary to the nucleotide in the SNP postion, to the SBE primer. SBE reactions can be multiplexed and many SBE products can be analyzed simultaneously by electrophoresis. In the electropherogram, the length of the SBE primer identifies the SNP locus and the color of the fluorescent label identifies the SNP allele. 19 Human Mitochondrial DNA Human mtDNA is ds-circular DNA and contains 16,569 bp of coding and control regions. One strand is purine rich and is termed Heavy (H). The other strand is Light (L) and is pyrimidine rich. Both strands code for proteins. The coding region contains 37 genes (lacks introns), encoding 2 ribosomal RNAs (12S and 16S rRNAs), 22 transfer RNAs (tRNAs) and 13 protein enzymes. The 22 tRNAs are the minimum set required for the translation of mtDNA, and all the 13 proteins are involved in the process of oxidative phosphorylation. All copies of mtDNA are generally identical (homoplasmic). In other words, the mtDNA genome is haploid and its sequence is treated as a single locus (haplotype). The D-loop contains binding sites for the major promoters of transcription and the origin of H-strand replication (OH). This region also contains the HV-I (hypervariable) and HV-II polymorphic regions. Thus, two hypervariable regions (HV-I and HV-II) of mtDNA can be determined by direct sequencing following PCR. The sequencing of HV-I and HV-II (and cytochrome b) not only provides individual identification but also confirms whether the sample is of human origin. 20 PCR Analysis of Hypervariable Regions in the D-Loop 21 Cytochrome B is Used to Confirm Human Sample, using Human-Specific Primers 22 DNA is Also Used to Identify Animals Sequence differences and profiles of PCR fragments generated from mtDNA can be used to identify species origin Swabs taken from Huanan Seafood Wholesale Market in early 2020 Those that tested positive for SARS-CoV2 were analysed for the presence of DNA from animals mtDNA contigs assembled and compared to sequences from wide range of animals Several animals confirmed Raccoon dog Amur hedgehog Bamboo rat Supports zoonotic origin of SARS-CoV2 Specific parts of mtDNA can also be used Hypervariable region I and II Crits-Christoph, Alex, Gangavarapu, Karthik, Pekar, Jonathan E., Moshiri, Niema, Singh, Reema, Levy , Joshua I., Goldstein, Stephen A., Suchard, Marc A., Popescu, Saskia, Robertson, David L., Lemey, Philippe, Wertheim, Joel O., Garry, Robert F., Rasmussen, Angela L., Andersen, Kristian G., Holmes, Edward C., Rambaut, Andrew, Worobey, Michael, & Débarre, Florence. (2023). Genetic evidence of susceptible wildlife in SARS-CoV-2 positive samples at the Huanan Wholesale Seafood Market, Wuhan: Analysis and interpretation of data released by the Chinese Center for Disease Control. Zenodo. https://doi.org/10.5281/zenodo.7754299 23 Tracing Genealogies by Mitochondrial DNA and Y Chromosome Analysis of mitochondrial DNA (mtDNA) can also be used in forensics. The main advantage is that mitochondrial DNA is present in multiple copies per cell and so is relatively easier to obtain in sufficient amounts for analysis. The sequence of mtDNA varies by 1% to 2% between unrelated individuals. Unlike nuclear DNA, progeny inherits its mtDNA directly from the mother (maternally inherited). Mechanisms behind the maternal inheritance of mtDNA include the reduction of paternal mtDNA during spermatogenesis, the simple dilution of spermatic mtDNA at fertilization and ubiquitin-mediated proteolysis of spermatic mitochondria and the active digestion of spermatic mtDNA within a fertilized ovum. mtDNA rarely undergoes recombination; mtDNA genomes are essentially clonal copies of the mother’s mtDNA genomes. The major disadvantage is that mitochondrial DNA does not vary between closely related individuals. Mitochondria are inherited maternally, and mitochondrial DNA sequences are therefore shared among groups of people derived from the same maternal lineage. 24 Tracing Genealogies by Mitochondrial DNA and Y Chromosome Mitochondrial DNA has been used to derive family ancestries. It is now possible to submit personal samples of DNA for analysis to companies like Oxford Ancestors and 23 and Me. Oxford Ancestors’ service allows people of European descent to trace their maternal ancestry back to one of 7 ancestral females (7 daughters of Eve). Ursula (Latin for “she-bear”), Xenia (Greek for “hospitable”), Helena (Greek for “light”), Velda (Scandinavian for “ruler”), Tara (Gaelic for “rock”), Katrine (Greek for “pure”), and Jasmine (Persian for “flower). 25 Results of Amplification and Sequencing the Hypervariable Regions Due to the high copy number of mtDNA, a mutation in some of the mtDNA results in a mixture of variant mtDNA genomes, a condition known as heteroplasmy. Heteroplasmy is defined as the presence of two or more subpopulations (types) of mtDNA genomes within a mitochondrion, cell, tissue, organ or individual, and may be observed in several ways, such as two or more mtDNA types in one tissue sample, and one mtDNA type in one tissue sample and a different mtDNA type in another sample One disadvantage of using mtDNA for forensic individual identification is the possibility that the occurrence of heteroplasmy will confuse the interpretation of the results and potentially lead to an erroneous exclusion rather than a match. However, the presence of heteroplasmy can also increase the power of the match when it is present in both the unknown and reference samples. There are over 200 known SNPs in each the D-Loop and coding regions of mtDNA. Next generation sequencing of mtDNA isolated from tissues and blood found at the crime scene could provide a similar power of identification as the 13 STR alleles that are now routinely analyzed in forensics labs. 26 Y-chromosome markers for male lineage In contrast to mitochondrial DNA, the Y chromosome follows a paternal pattern of inheritance. Most of the human Y chromosome (i.e. the non-recombining region of the Y chromosome, NRY) is male specific and is inherited unchanged from fathers to sons. Males are XY and have only one copy of the Y chromosome and so there is no recombination because there is no second chromosome. This makes Y chromosome polymorphisms (STRs) very useful for male identification. The most important application of Y-chromosome markers for male lineage identification in forensics is in cases of sexual assault. The specific detection of male DNA by analyzing Y-chromosomal STRs in principle avoids the problem of profile overlap (since females do not carry a Y chromosome) and additionally is much more sensitive. Mixing experiments have shown that Y-STRs can be amplified successfully and reliably 27 up to male–female DNA mixtures of 1:2000 Forensic Epigenomics – Age and the Epigenetic Clock Epigenetic analysis could serve as a method for age estimation of a suspect based on blood left at a crime scene. Based on three selected CpG sites (NPTX2, EDARADD and TOM1L1), age predictions correlated with an average precision of ±5.2 years (Bocklandt, et. al.). An epigenetic clock is a type of DNA clock based on measuring natural DNA methylation levels to estimate the biological age of a tissue, cell type or organ. An example for an epigenetic clock is Horvath's clock, which is based on 353 epigenetic markers on the human genome. If all of the 353 epigenetic markers are measured, the median error of estimated age is ±3.6 years across a wide spectrum of tissues and cell types. Epigenetic measurements are better than the correlation of age with telomere length, or mutation accumulation. 28 DNA methylation and Age Increase in DNA methylation level with age of one CpG site (cg16867657) in the promoter of the ELOVL2 (elongation of very long chain fatty acids protein 2) gene and corresponding regression line. 29 Tissue Identification via mRNA Analysis Forensic DNA analysis provides information on personal identity and characteristics. However, it does not reveal which tissues of the body the evidence sample comes from. Different tissues have different patterns of gene expression that is reflected in variation in their messenger RNA composition. A set of 137 tissue-specific candidate genes was selected for 4 different body fluids. In this experiment, mRNA was extracted from 24 Korean body fluid samples, 6 each from semen, blood, vaginal swab, and saliva. Unsupervised hierarchical clustering of 137 candidate genes was performed to yield the diagram shown. Each body fluid has an individual signature that allows it to be distinguished. Tissue-specific, non-coding miRNA (20-25 bases in length) may be a superior replacement of mRNA (mRNA is too large and unstable). Several miRNAs show tissue specific expression and are present in sufficient amount for forensic screening. 30 References: Glick, BR., Pasternak, JJ., Patten, CL. (2010). 0003 DNA. Washington DC: ASM Press. Clark, DP., Pazdernik, NJ. (2016). Biotechnology. Amsterdam [u.a.]:Academic Cell. Nelson, DL., Cox, MM. (2013). Lehninger Principles of Biochemistry. New York: W.H. Freeman and Company. Rapley, R., Whitehouse, D. (2007). Molecular Forensics: John Wiley & Sons, Ltd. 31 Supplementary Materials (Not on Exam) 32 Supplementary Videos and Links How DNA Changed the World of Forensics https://www.nytimes.com/video/us/100000002886783/how-dna-changed-the-world-offorensics.html STRBase http://strbase.nist.gov/ Forensic DNA and Molecular Biology https://www.youtube.com/watch?v=mpqzol3pPok Forensic Biology (DNA and Serology) Evidence https://www.youtube.com/watch?v=V_P0U29Ndvs 33 BCHB-5260 Core Methods in Biotechnology Aging and Apoptosis: why we age is still a mystery. Why Aging Occurs Molecular reason for aging: slow accumulation of wear and tear on our cells, tissues, organs, and ultimately on our bodies as a whole. A. Accumulation of genomic changes (genomic instability) 1. Mutations that are not repaired including loss of chromosomes, copy number variations in genes, base damage that leads to missense mutations, and chromosomal translocations 2. Telomere shortening 3. Epigenetic changes in DNA (Horvath Clock) 4. Persistent DNA damage, endogenous and exogenous sources B. Cellular dysfunction i. These processes may include alterations in intercellular communication, nutrient sensing, metabolism and protein function, and mitochondrial function. Why Does Aging Occur? Aging is a nearly universal feature of biological organisms. Among multicellular organisms, aging is marked by a progressive decline in the function at all biological levels. In organisms with renewable tissues, aging is also marked by an increase in cancers. Why does aging occur? Evolutionary theory holds that aging is a consequence of the declining force of natural selection with age. Extrinsic hazards—accidents, predation, infection, starvation, and so forth—limit the life span of most species, thereby depleting natural populations of older individuals. Consequently, there are generally few old survivors on which natural selection can act to eliminate alleles or genes that have late-acting deleterious effects. Collectively, chronic diseases comprise the majority of global disease burden and are the most common causes of mortality. Aging is also called “life-span”. The goal of life-span research is to increase “healthspan”, that period of our lives in which we are healthy. The major risk factor for health-span is chronic disease. If we can understand how aging enables disease, it may be possible (and even easier) to target this common component of disease. Targeting aging may allow early intervention and damage avoidance, maintaining vigor and activity while offsetting the economic burdens of a burgeoning aging population hampered by multiple chronic diseases. A. Genomic instability: 1. Accumulation of Genetic changes: Oxidative Stress Damages Surrounding Molecules As we age genetic mutations accumulate. A. Genomic instability: 1. Accumulation of Genetic changes: Oxidative Stress Damages Surrounding Molecules As we age genetic mutations accumulate. Oxidative stress can generate mutations in nuclear and mitochondria genomes. Most of oxidizing radicals form during aerobic respiration. Mitochondrial electron transport, which manufacturers most of the ATP, uses about 80% of the oxygen. Partial reduction of the O2 molecule creates highly reactive oxygen species (ROS; e.g., superoxide ions, peroxides, and hydroxyl radicals) ROS attacks mitochondrial DNA, cellular proteins, lipids, and nuclear DNA. Damaged mitochondrial DNA may produce mutant respiratory chain proteins, which in turn make more ROS and/or less ATP. If the damage is extensive, the cell will senesce or die. Senescence is the state where a cell is alive; it undergoes metabolism but is unable to replicate. A. Genomic instability: 2. Telomere Shortening TTAGGG The cells of all mammals contain a genetic clock. At the end of our chromosomes are telomeres, which become slightly shorter every time a cell divides. Consequently, the length of the telomeres reveal the age of our cells. When the telomeres have been ”spent”, the cell dies, leaving the body weaker and older. However, the telomerase enzyme can extend our chromosomes, delaying cell death. The enzyme telomerase repairs the shortened ends of the chromosome by adding more repeats to the ends. Telomerase is a non-typical reverse transcriptase with two components: protein and RNA. The protein component called TERT catalyzes the synthesis of new DNA, and the RNA component called TERC acts as a template. (TERT: telomerase reverse transcriptase. TERC: telomerase RNA component) Unfortunately, we do not have enough telomerase in our cells to overcome the ravages of time, and so, the chromosomes slowly become shorter. Telomerase Shortening Causes Cellular Senescence A cell with long telomeres is able to continually divide and multiply. A cell with short telomeres may enter senescence A. Genomic instability: 2. Telomere Shortening Telomerase consists of a protein (TERT) plus an RNA (TERC) component. The RNA recognizes the telomere repeats, and the protein elongates the telomeres DNA polymerase cannot synthesize the end of chromosomes, and therefore, telomere ends get shorter with each cell division. The enzyme telomerase can lengthen telomeres in some cells. Mutations in telomerase components have symptoms that recapitulate aging, suggesting the telomere length and stability are key genetic factors in aging The human inherited disease dyskeratosis congenital (DKC) is linked to lower telomerase activity. People with DKC have symptoms of aging, e.g., pulmonary disease, graying and loss of hair, learning difficulty, and blocked tear ducts. A. Genomic instability: 3. Epigenetic changes associated with aging DNA methylation (compacting chromatin DNA and preventing transcription factors from accessing the genes) often decreases as we age Some genes are on the other hand hypermethylated during aging, such as lamin A/C gene. Lamins are proteins that line the nucleus to provide architecture around the genome. Mutation of lamin A/C causes premature aging, a symptom known as Hutchinson-Gilford Syndrome, or progeria. Hypermethylation of lamin A/C is believed to associate with age-related decline symptoms seen in normal persons. Histone modifications: determine if other proteins can access the DNA. Reversible. C. elegans and Drosophila have extended life spans when certain histone methylases are deleted. A. Genomic instability: 3. Epigenetic changes associated with aging Sir2 (Sirtuins 2; a NAD+-dependent histone deacetylases) has been shown to induce longer replicative life span in yeast cells (HDAC compacts chromatin, less homologous recombination, less extrachromosomal ribosomal DNA circles, less genomic instability) Chromatin structural changes: Euchromatin and heterochromatin The amount of heterochromatin declines with age. When heterochromatin DNA relaxes, repetitive DNA regions (e.g., telomeres and centromeres) can become transcribed into non-functional mRNA, which in turn destabilizes the genome. HP1 α (heterochromatin protein 1 alpha) is associated with maintaining the amount and location of heterochromatin. Extra HP1 α increases life span. Genomic instability may be another important aspect of aging. Sam Berns B. Cellular dysfunction and aging: Nutrient Sensing and Metabolic Rate Regulate Aging Insulin and IGF-1 signal transduction pathway (IIS pathway): is responsible for coordinating growth, differentiation, and metabolism in response to the environment. When this pathway is impaired (alter) in humans, we develop type 2 diabetes. Also, some evidence suggests that the pathway is involved in longevity. slowing down metabolism rate B. Cellular dysfunction and aging: Nutrient Sensing and Metabolic Rate Regulate Aging Insulin and IGF-1 signal transduction pathway (IIS pathway): description of the signaling pathway When insulin binds to the human insulin receptor, the intracellular domain is activated by phosphorylation. The phosphate is transferred via IRS-1 (insulin substrate 1) to PI3-kinase (phosphatidylinositol 3-kinase), which converts the lipid molecule phosphatidylinositol 3,4-bisphosphate (PIP2) to phosphatidylinositol 3,4,5triphosphate (PIP3) by adding a phosphate. PIP3 triggers the intracellular response to insulin by preventing the FOXO family of transcription factors from entering the nucleus (FOXO: Forkhead box O) FOXO transcription factors activate genes associated with resistance to oxidative stress and decreased metabolism When mutations or caloric restriction block signaling through these pathways, FOXO can enter the nucleus and activate genes associated with reduced oxidative stress and decrease in metabolism, which can extend the life span of the organism. B. Cellular dysfunction and aging: Metabolic Regulators During Reduced Caloric Intake can Affect Aging Mammalian Target of Rapamycin (mTOR), is a master growth regulator that reads environmental cues such as energy levels and cellular stress, through complex cell signaling pathways. mTOR kinase adds phosphates to serine or threonine on other proteins, and is involved in sensing amino acid availability and regulating a variety of anabolic processes to promote cellular growth. mTORC1 is activated by insulin and other growth factors through PI3K and AKT kinase signaling. The kinase is found in one of two complexes, mTORC1 and mTORC2, and rapamycin treatment inhibits mTORC1, which delays age-related diseases and extends the life span of mice. The biotech industry is working to inhibit the mTOR pathway to prevent age-related declines without using rapamycin, since this drug has side effects such as insulin resistance, cataracts, and testicular degeneration. Another molecule that regulates life span is AMP-activated kinase (AMPK), which senses the amount of AMP and ADP that accumulate with ATP utilization. So, energy depletion by caloric restriction, hormones, or exercise triggers AMPK activity (The AMPK protein is a metabolic sensor that acts as a qualified cellular housekeeper, as well as controlling energy homeostasis and resistance to stress) Metformin, an activator of AMPK, has been shown to prevent cancer and cardiovascular disease from developing by regulating blood sugar levels, and therefore, rodents have a longer life span. Protein Degradation Becomes Less Efficient with Aging 1. Chaperones (also known as heat shock proteins) are enzymes found in the cytosol, mitochondria and endoplasmic reticulum that repair improperly folded proteins by unfolding and refolding them. Chaperones are significantly impaired in aged tissues, (leading to accumulation of damaged proteins throughout the cell), and therefore, reinvigorating these proteins may be one mechanism to combat aging. 2. Ubiquitin-proteasome system degrades proteins that are marked with ubiquitin chains. Ubiquitin-ligating enzymes (E1, E2 and E3), as well as proteasome complex undergo age-related decline, leading to accumulation of damaged proteins. Protein Degradation Becomes Less Efficient with Aging 3. The microautophagy-lysosome system, and 4. chaperonemediated autophagy identify defective proteins and move them into the lysosome for degradation. In chaperone-mediated autophagy and microautophagy, lysosomes degrade defective proteins, but how the protein reaches the lysosome differs. Chaperone-mediated autophagy uses a chaperone to recognize the defective protein and deliver it to the lysosome via LAMP-2A (lysosomal-associated membrane protein type 2A) receptor on the lysosome surface, whereas in microautophagy system, lysosome simply engulfs whole area of the cytosol by wrapping its organelle membrane around the area, a direct uptake of the defective protein by the lysosome. In aged tissues, both of these systems do not work as efficiently. Mitochondria and Aging Reactive oxygen species trigger oxidative stress. Mitochondria are a major source of ROS. However, mitochondria turnover declines with age, leading to accumulation of damaged mitochondria within the cells. Autophagy is the mechanism that destroys and recycles defective cellular components. Macroautophagy or autophagy targets whole organelles such as mitochondria to be degraded. A double-membrane vesicle called an autophagosome forms around the defective organelle, fuses with the lysosome to become an autophagolysosome, and then the entire organelle is degraded by the lysosomal enzymes. Autophagy increases when there is nutrient deprivation. Autophagy of defective mitochondria is shown an effective way to prevent some symptoms of aging in mice. Autophagolysosomes Cellular Senescence Senescence prevents the cellular damages from getting out of control. This metabolic state is activated when cells are damaged, and prevents the cell from creating new damaged descendants since senescent cells exit the cell cycle (arrest in G1) and simply stop dividing. Senescent cells are cell cycle arrested, but metabolically active; yet the cells do not replicate their DNA or divide. Cells have active tumor suppressor molecules such as pRb and p53 to control whether a cell enters senescence or apoptosis. Phenotypes Associated with Senescence Cellular Senescence Senescent cells accumulate a substance called senescence-associated β-galactosidase. This enzyme works to degrade sugars in the lysosome of a cell, and the increased amount of the enzyme reflects an increase in the number of lysosomes of senescent cells. Senescence cells also have an altered chromatin structure (senescence-associated heterochromatin foci) these shut-down proliferation-associated genes. Senescent cells secrete more factors, a phenomenon called senescence-associated secretory phenotype. Some secreted substances include pro-inflammatory cytokines and extracellular matrix factors. As we age, the number of some stem cells decreases. Immuno-senescence is one example of stem cell depletion that occurs with aging. (The number of hematopoietic stem cells decline with age and result in fewer white blood cells circulating in the blood) Phenotypes Associated with Senescence Programmed Cell Death When cellular damage accumulates beyond a point of repair, normal cells activate programs to commit suicide in a controlled pathway called programmed cell death. 1. The most studied programmed cell death is apoptosis (Type I). During apoptosis process, the dying cell undergoes a morphologically distinct process. First, the cell membrane starts to form blebs, or regions that balloon out. The nucleus shrinks, condenses. The entire cell shrinks and the nucleus fragments and other cellular components are degraded by enzymes called caspases into large condensed fragments called apoptotic bodies. Macrophages engulf the debris by phagocytosis, thus cleaning up the remnants. The proteins, lipids, and nucleic acids are digested and recycled. 2. Autophagy is another method of programmed cell death, which is used to remove damaged cellular proteins or damaged mitochondria. It can also be used to degrade entire cells (type II programmed cell death). 3. Necroptosis is called type III programmed cell death. Caspases (cellular aspartate-specific protease), enzymes that digest cellular proteins by cutting after aspartic acid residues. (Type I) Mammalian Apoptosis Mammalian cells have two major pathways to trigger apoptosis. The death receptor pathway, or extrinsic pathway, originates at a cell surface receptor protein, or death receptor. An external death signal molecule binds to the extracellular domain on the receptor. The receptor transmits the signal to its intracellular domain and then recruits a variety of internal proteins to the membrane. This ultimately activates the caspases or intracellular proteases. The other pathway is the mitochondrial death pathway, or intrinsic pathway. It is usually triggered by intracellular catastrophe, such as irreparable DNA damage. This activates proteins in the mitochondria, which release different effector molecules to activate the caspases. Mammalian Apoptosis: 1. Death Receptor Pathway An external signaling molecule called CD95, or Fas ligand can initiate cell death. When three molecules of CD95 bind to the CD95 receptor, the three receptors come together to form one complex (trimerize), which activates the intracellular domains. The death-inducing signaling complex (DISC) is then recruited and binds to the receptor. The key component of DISC is caspase-8. Caspase-8 is activated when the prodomain is cleaved off. The main target for caspase-8 is caspase-3. Activated caspase-3 induces apoptosis of the cell. Initiator caspase (e.g., caspase-8) and executioner caspase (e.g., caspase-3) Tumor Necrosis Factor Induction of Apoptosis by the Death Receptor Pathway Three TNFα ligands bind to three TNFR1 receptors to aggregate the receptors into one complex and activate the receptors This recruits TRADD, TRAF2, RIP1, and RIP3 to the intracellular portion of the receptor to form Complex I. Complex I and the receptors are internalized and then associated with FADD and caspase-8 forming Complex II. Two molecules of caspase-8 cleave the inhibitory pro domains from the caspase to activate it. Activated caspase 8 initiates apoptosis via caspase-3 activation. TNFR1: tumor necrosis factor receptor 1 TRADD: TNF receptor-associated protein with a death domain TRAF2: TNF receptor-associated factor 2 RIP1 and RIP3: receptor interacting proteins 1 and 3 FADD: Fas-associated death domain 3 Mammalian Apoptosis: 2. Mitochondrial Pathway of Apoptosis Severe DNA damage signals for an increase in Bax/Bax dimers. These form a channel through which cytochrome c escapes from the mitochondria. Once released, cytochrome c induces formation of the apoptosome, a signaling complex containing cty c, caspase-9 and Apaf-1 (apoptotic protease activating factor 1). Cytochrome c binds to Apaf-1 and induces a conformational change. Activated Apaf-1 assembles into a heptamer, which binds to procaspase-9 and cleaves off the prodomain. Activated caspase-9 (initiator caspase) activates caspase-3 (executioner caspase), and carry out the death sentence. 22 Mammalian Apoptosis: 2. Mitochondrial Pathway of Apoptosis Bax/Bcl-2 Control of Apoptosis The mitochondrial pathway is usually activated by internal stimuli such as irreparable DNA damage. The signal converges on a family of mitochondrial proteins, named after its founding member, Bcl-2 (first identified in a B-cell lymphoma). These proteins are active as dimers. Some Bcl-2 family proteins induce apoptosis, whereas others protect cells from apoptosis. The ratio of pro-apoptotic (Bax) and anti-apoptotic (Bcl-2) dimers controls whether cells die via apoptosis. Western protein analysis Execution Phase of Apoptosis Caspase-3 activates other caspases and each digests different cellular substrates. Caspase 3 activates caspase-activated DNAse (CAD), a nuclease that cuts nuclear DNA between the nucleosomes. The fragments of DNA are about 180bp in length. To determine if cells are in the process of apoptosis, scientists isolate the genomic DNA and look at the fragment sizes. If they are in multiples of approximately 200 base pairs, this implies the cells were going through apoptosis. There are many other substrates of caspases. Proteins that maintain nuclear structure, such as lamins, are digested so that the nucleus shrinks and breaks into small pieces. The cytoskeleton is cleaved, and the cell architecture disintegrates and compacts. Other organelles are digested so they lose their structure, which creates small compact granules of cellular materials called apoptotic bodies. 24 Removal of Apoptotic Bodies In mammals, most apoptotic bodies are engulfed by macrophages through phagocytosis. These recognize cell surface receptors (phosphatidylserine) on the apoptotic body and ingest the entire cellular fragment. Phosphatidylserine, a phospholipid, normally is found only in the inner leaflet of the cell membrane. During apoptosis, phosphatidylserine may be translocated to the outer leaflet. Microphages have receptors for phosphatidylserine, allowing them to recognize the apoptotic body as “self” (this process does not induce an inflammatory nor an immune response) Any remaining proteins are broken down and recycled by the macrophage. 25 Apoptotic Bodies Do Not Trigger an Immune Response by Macrophages A. Normally, macrophages ingest invading pathogens such as bacteria. This triggers the macrophage to release factors that attract other immune cells. B. In contrast, the cell surface signal on apoptotic bodies does not trigger the macrophage to release these factors, so other immune cells are not recruited to the site. (Type III) Necroptosis Necrosis has one main characteristic: the early rupture of the cellular membrane that leaks the cellular contents into the intracellular area, which then elicits an immune response. Necrosis can be regulated. In particular, a necrotic signal transduction pathway centering on serine/threonine kinases called Receptor Interacting Protein 1 (RIP1) and RIP3, is called necroptosis. Necroptosis signals overlap with the extrinsic pathway, or death receptor pathway of apoptosis. When TNFα binds to its receptor TNFR1, one of the 3 different pathways can be activated depending on what molecules associated with the intracellular domain. 27 (Type III) Necroptosis If complex I forms, then NFκB activates cell survival genes. If complex II forms with dimers of caspase-8, then apoptosis is activated. If caspase-8 associates with FLIP (FLICE-like inhibitory protein), caspase-8 can not self-activate, and therefore, caspases are not activated. Instead, the complex activates necroptosis, where the cell ruptures and leaks all of its intracellular contents into the surrounding tissue. NFκB: nuclear factor kappa-light-chain-enhancer of activated B cells FLICE: FADD-like ICE, FADD-homologous ICE/ced-3-like protease 28 Cancer, Aging and Programmed Cell Death The pathways of senescence and apoptosis work to protect the organism from damaged cells. When a cell becomes damaged, it enters senescence early. Severely damaged cells commit suicide via apoptosis. Damaged cells that bypass the senescence program and block apoptosis are can become carcinogenic. Key signal transduction pathways regulated oncogeneinduced senescence: p53 pathway and pRb pathway. If cells are defective for both p53 and pRb, the cell replicative life span is longer than with either mutation alone. Eventually the mutants do stop dividing unless some further oncogenic mutation occurs. Defects in p53 and pRb extend cellular life span and often leads to cancer Cancer, Aging and Programmed Cell Death The normal p53 protein is a transcription factor that regulates its own gene as well as a variety of other genes in the suppression of tumors. In particular, p53 activates transcription of p53-upregulated modulator of apoptosis (PUMA), a member of the Bcl-2 protein family that activates Bax, thus promoting apoptosis. P53 also activates p21, a cell cycle inhibitor that causes the cell to stop dividing. P53 mutations are found in up to 90% of cancers. BIOT-5260 Core Methods in Biotechnology Lecture #21 Cloning and Stem Cells 1 What is a Stem Cell? Stem cells have 3 main characteristics: 1) An undifferentiated cell 2) Potential to differentiate into multiple cell types 3) Can enter mitosis to divide and make: a. More stem cells that remain undifferentiated b. Cells that differentiate into different cell types (when put into the right environment). This provides a source to replace old or damaged cells that are removed by apoptosis. 2 Totipotent and Pluripotent Stem Cells: Early embryonic cells (morula cells) from a zygote are totipotent; these cells have the information to differentiate into all cells in the body and form the entire organism. As the zygote develops, hollow ball of cells called a blastocyst forms. At one end of the hollow ball of cells, more cell division and cell migration leads to a build-up of cells called the inner cell mass. Inner cell mass cells are pluripotent; they are able to differentiate into any tissue in the body except for placenta. These cells are isolated and grown in a culture dish and the resulting cell lines are called embryonic stem cell lines. Trophoblast cells Graphic: https://en.wikipedia.org/wiki/Cell_potency 4 Embryonic development is considerably more complex than simply going from the fertilized egg to the blastocyst. 5 6 Embryonic Stem Cells: When inner cell mass cells are isolated and grown in a cell culture dish, they must be assayed to check if they maintain these stem cell properties: Can they divide indefinitely? Can they remain undifferentiated (check for uniform cell shape and size) Do they express transcription factors specific for stem cells (OCT4 and NANOG). Can they be triggered to differentiate. Treat cells with specific growth factors; cells will form embryoid bodies first, then differentiate into somatic cells like neurons or muscle. The ability to control which types of cells that embryonic stem cells can differentiate into is one key goal of stem cell research (this ability could provide a source of cells that could regenerate damaged human tissues). Embryonic stem cells aggregate and form large spherical masses when they are unable to adhere to a cell culture dish. Source: Novusbio, v6.5 mESC line. 7 Multipotent Stem Cells (Adult Stem Cells): Adult stem cells (or somatic stem cells) are multipotent; they can only differentiate into a small subset of cells. They are found throughout the body and divide to replenish dying cells and regenerate damaged tissues. Examples of adult stem cells: hematopoietic (blood-forming) stem cells, small intestine stem cells, and skin stem cells Adult stem cells are rare (e.g., about 1 in 10,000-15,000 cells in the bone marrow is a hematopoietic stem cell. These cells are dispersed in tissues throughout the mature animal and behave very differently, depending on their local environment. 8 Ref: NIH. Stem Cell Information. The Adult Stem Cell. Retrieved from https://stemcells.nih.gov/info/2001report/chapter4.htm Example of Multipotent Progenitor Cells - Hematopoietic Stem Cell Differentiation 9 Identifying Adult Stem Cells: Adult stem cell population is often a very small percentage of the tissue or organ In some tissues, these stem cells are scattered. In some tissues, they are found in a defined niche. There has not been one single biomarker that definitively identifies every adult stem cell; each adult stem cell type is unique. Some stem cells (e.g., intestine, skin, lungs, blood and muscle) express signal transduction proteins like Notch to prevent differentiation. Signal transduction proteins may persist even after a stem cell has fully differentiated, and therefore may not be specific enough for identifying stem cells. 10 Identification of Adult Stem Cells Fate mapping or lineage analysis is used to identify stem cells: label the potential stem cell with a molecular marker and then trace where the marker is found after many generations. If the marker labels a true stem cell, as the stem cell divides the marker will pass to the daughter cells and remain in the stem cell. 11 Key Features of A Stem Cell Niche A stem cell niche is a microenvironment that directly promotes or protects a population of stem cells The environment supports the stem cells maintaining the undifferentiated state The stem cells can receive signals through direct interaction with niche cells and/or extracellular matrix. The stem cells can also receive signals from cell surface receptors. The signals can be secreted by themselves (autocrine) or from niche cells (paracrine). Proper niche function maintains the stem cell population to support tissue regeneration. 12 Open and Closed Stem Cell Niches: Stem cell niches can be categorized by their make-up. Open stem cell niches have no geographical boundaries for the microenvironment and the stem cells. Interaction between neighboring niches is common where stem cells from one region can migrate to another. Example: open stem cell niche of seminiferous tubules. The spermatogonial stem cells (green ovals with red dots) are found in an open niche situated between Sertoli cells (purple cells) and a basement membrane (blue line). 13 Open and Closed Stem Cell Niches cont’d: In contrast, closed stem cell niches have fixed boundaries that enclose the environment and prevent the stem cells from migrating. A closed niche is surrounded by an extracellular matrix or a capping cell. Example: C. elegans oocyte stem cells express Notch The stem cells responsible for creating more oocytes in C. elegans are found in a niche that is capped by a single cell called the distal tip cell. The distal tip cell caps the area and sends long processes or fibrils that surround the area. This cell emits signals that activate the Notch pathway, which in turn keeps the stem cell from differentiating. As the stem cell moves away from the niche, the Notch signals fade and then the cell can enter meiosis. As the stem cell divides, cells on the periphery of the niche are displaced and exit the range of the distal tip cell signals. 14 Stem Cell Divisions Can be Symmetrical or Asymmetrical Asymmetric division Stem cells contained within a niche often undergo asymmetric cell division to produce one daughter cell that differentiates into a specialized cell and another daughter cell to maintain the stem cell population. Symmetrical renewal and symmetrical differentiation are also possible. In symmetrical renewal, stem cell division creates 2 daughter cells and they are both stem cells. In symmetrical differentiation, stem cell division creates 2 daughter cells, and they both differentiate. 15 Asymmetrical Stem Cell Division The signals that affect each of the 2 daughters are different, and can either arise from an intrinsic asymmetry between the 2 daughter cells or from an extrinsic asymmetry. Intrinsic asymmetry results in the distribution of differing amounts of a cell-fate determinant to each daughter cell (e.g., protein, RNA) which tells the daughter cells to differentiate, or stay a stem cell. Extrinsic signals from the niche often maintain cells in their stem cell fate, and when the daughter moves to a new environment, the loss of the signal or the addition of a new signal triggers this daughter to differentiate. The other cell remains in the same environment and therefore remains a stem cell. Both examples are of ‘asymmetric division’ 16 Intestinal Epithelial Stem Cells The tissue of the small intestine completely replenishes every 3-5 days (mouse), and ~7 days (humans). Adult stem cells provide the various cell types found in the intestine. Crypts between 2 different finger-like projections called villi, harbor a population of stem cells (CBC, crypt base columnar cells) that replenish the villi with new absorptive epithelial cells (enterocytes), enteroendocrine cells, and goblet cells. The cells migrate up the villi and then are shed from the villus into the lumen, where they are excreted with the waste. The stem cell niche contains Paneth cells, which secretes antimicrobial molecules that regulate the type of bacteria found in the intestine. (stem cells) Enterocytes: absorb nutrients from the intestinal lumen and pass them into the bloodstream. Goblet cells: secrete mucous to lubricate the mucosal surface Enteroendocrine cells: secretes 17 digestive hormones Intestinal Epithelial Stem Cells Intestinal stem cells can differentiate into all 4 types of cells found in the villi of the intestine: absorptive epithelial cells (enterocytes), goblet cells, enteroendocrine cells, and Paneth cells. Initially, the stem cell daughter cells exist in an intermediate state of differentiation, called transit amplifying cells (TA cells). The transit amplifying population retains the ability to divide by mitosis, but only a finite number of times. Fully differentiated intestinal cells cannot divide. (crypt base columnar cells 18 Lgr5 lineage tracing experiment: Lgr5, which is a membrane receptor for Wnt signaling pathway. Wnt signaling promotes stem cell proliferation. Lgr5+ stem cells divide rapidly up the sides of each adjacent villi. Lgr5+ cells expressing CFP are colored blue (CFP expression under the control of Lgr5 promoter). 1 day after induction: only one CBC cell is blue in 2 different crypts. After 6 months, one entire villus has cells with CFP that they inherited from the original CBC cell, whereas the other labeled CBC stem cell did not create descendants that populated the villus with cells. 19 Induced Pluripotent Stem Cells (iPSC) 20 Induced Pluripotent Stem Cells (iPSCs): Differentiated somatic cells can be converted into an undifferentiated pluripotent stem cells (Induced Pluripotent Stem Cells, iPSCs) through action of key transcription factors. – Adult cells of one tissue have the ability to become other types of cells using this technique. The use of somatic cells to create the undifferentiated cells for regenerative medicine holds true promise. 21 Ref: R&D Systems. Differentiation Potential of Induced Pluripotent Stem Cells. Retrieved from https://www.rndsystems.com/resources/articles/differentiation-potential-induced-pluripotent-stem-cells Induced Pluripotent Stem Cells (iPSCs) Human differentiated somatic cells (fibroblasts) can be isolated and cultured in dishes. If the transcription factors OCT4, SOX2, NANOG, and LIN28 are activated, the cells return to their undifferentiated stem cell state (reported by Yu et al. in 2007). In a similar way, mouse somatic cells (fibroblasts) can be reprogrammed from the differentiated state to an undifferentiated stem cell fate by expressing 4 transcription factors: OCT4, SOX2, KLF4, and c-MYC (reported by Takahashi and Yamanaka in 2006). Only about 1% of cells eventually become fully reprogrammed. Other unknown regulatory elements exist. 22 Induced Pluripotent Stem Cells (iPSCs): iPSCs bring a lot of promise to use one of the patient’s somatic cells to regenerate a damaged organ (personalized and immunologically compatible treatment) iPSCs circumvent the use of embryos for studying cellular differentiation May allow for discovery of new ways for cells in our body to be directly reprogrammed from one state to another (e.g., reprogram cancer cells into normal cells) OCT4 is a transcription factor encoded by the gene called Pou5f1. This transcription factor is present in the zygote during the early cell divisions and is ultimately expressed in the inner cell mass of the blastocyst. – OCT4 is an important regulator for NANOG protein expression. It is believed to be the “master regulator” of embryonic development (no OCT4 = no inner cell mass). SOX2 can form a heterodimer with OCT4 to activate transcription of key genes for the pluripotent phenotype, such as Nanog. SOX2 is not expressed as early in development as OCT4 and is preferentially found in early development of neural stem cells. KLF4 and c-MYC are other key molecules to create iPSCs. c-Myc appears to be mouse specific since its overexpression in human cells is lethal. KLF4 is essential for self-renewal and maintenance of stem cells NANOG is used to stabilize the pluripotent phenotype rather than establish it. LIN28 is a key regulator of developmental timings and to maintain a state of “stemness” in the iPSCs. 23 Cardiomyocytes (Heart cells) derived from iPSC stem cells beat in culture dish Ref: https://www.youtube.com/watch?v=WLtJTywFpoI 24 Hematopoietic Stem Cells: Hematopoietic stem cells (HSCs) is likely the most widely used stem cell therapy to date. The bone marrow is a rich source of HSCs. Bone marrow transplants are widely used today to treat people with cancers of the white blood cells (e.g., leukemia). Donor bone marrow is derived from the patient (autologous) by harvesting his or her own stem cells before undergoing chemotherapy. These stem cells are then replaced when the chemotherapy is finished (rescue transplant). Allogeneic hematopoietic stem cell transplants use HSCs harvested from donors with the same blood type and similar HLA types. Umbilical cord blood is another rich source of HSCs. These cells can ben frozen and stored following delivery. Source: stemcells.nih.gov 25 Stem Cell Therapy Another source is peripheral, circulating blood. Stem cells leave their niche area and migrate into the bloodstream. If the patient receives a dose of granulocyte-colony stimulating factor (G-CSF), a cytokine that induces the migration of stem cells out of the marrow and into the bloodstream. The HSCs can be purified from the blood by collecting the white blood cells with a cell surface protein called CD34. About 525% of CD34+ cells will be HSCs. This way of collecting HSCs is by far the easiest and has much lower morbidity; therefore, it has become the method of choice. 26 Somatic Cell Nuclear Transfer: The somatic nucleus has the information to create the entire organism. The nucleus just needed the correct environment. The major accomplishment for this field of research was the creation of Dolly the sheep in 1996 at the Roslin Institute in Scotland. Cloning animals relies on the technique of nuclear transplantation. Fuse a somatic cell with an enucleated egg cell results in the donor nucleus being placed into a completely undifferentiated cytoplasm. A brief electrical pulse fuses the 2 cell membranes into 1 embryo. Dolly was the first mammal to be produced using a nucleus from a differentiated cell line (mammary gland or udder cells). The success shows that it is possible to reset the clock of an adult cell to zero and start development again, a process that is reproduced in making pluripotent stem cells. 27 Somatic Cell Nuclear Transfer In Dolly’s case, the trick was to starve cultured udder cells from the donor animal so that both the cell and the DNA stopped dividing. When the resting G0 nucleus is placed in an egg cell, whose nucleus has been removed, it starts dividing again. The egg is then transplanted into a female animal, where it will develop into an embryo. Since Dolly’s birth (7/5/96), a variety of other animals have been cloned. 28 Somatic Cell Nuclear Transfer Since Dolly’s birth, a variety of other animals have been cloned. There are practical reasons for cloning animals. – For example, genetically identical animals will give wool, milk, eggs, or meat of a more standardized quality. Cloning also allows us to genetically rescue rare breeds of endangered species. Strictly speaking, Dolly was not a complete clone. In addition to the nucleus, which contains the majority of the genetic information, animal cells contain a few genes in their mitochondria. In Dolly’s case, only the nuclear DNA was cloned. The mitochondrial DNA was provided by the egg cell that received the nucleus. 29 Back to iPSC for a moment… 30 iPSCs in Brain-derived Endothelial Cells (BBB) DiVito KA, et al. Biomaterials. 2017 31 References: Clark, DP., Pazdernik, NJ. (2016). Biotechnology. Amsterdam [u.a.]:Academic Cell. Nelson, DL., Cox, MM. (2013). Lehninger Principles of Biochemistry. New York: W.H. Freeman and Company. 32 Supplementary Materials (Not on Exam) 33 Supplementary Videos and Links What are Induced Pluripotent Stem Cells? (iPS Cells): https://www.youtube.com/watch?v=i-QSurQWZo0 https://www.youtube.com/watch?v=HXvRbffAhn8 Pluripotency at the Molecular Level https://www.youtube.com/watch?v=_PPRV7GWi2I Intestinal Organoid Culture - The Next Dimension in Intestinal Research https://www.youtube.com/watch?v=84_CtzDLsS0 The Story of Dolly the Cloned Sheep https://www.youtube.com/watch?v=tELZEPcgKkE This powerful documentary looks at the controversial attempts to clone a human being https://www.youtube.com/watch?v=-zTDUZKFb6k What is Adult Stem Cell Therapy- Trinity Spine and Wellness Center New Port Richey https://www.youtube.com/watch?v=kss25TF4ckY 34 Cloning and Stem Cells What are Stem Cells? New Concept: Differentiation is Reversible! Petting Zoo Version Jungle Version They divide asymmetrically, without apparent limit. (Imagine culturing them for >120+ years!) Totipotent stem cells have the ability to generate the precursors of all of the specialized cell types. Multipotent stem cells are more limited and can give rise to subsets of differentiated cell types. Differentiation inducers are known. Maintenance of pluripotency culture conditions are known. Stem cells are the key to regenerative medicine. Embryonic Stem Cells. Embryonic Stem Cells. Come from Blastocyst – ~5 day old embryo Are Pluripotent – Can form any cell type Unlimited self-renewal – Enhanced telomerase activity (resists aging) Can form teratoma – Can also occur in oter stem cells Animal pathogens – Reagents during cultivation Immune reactions – MHC expression levels Ethics – Banned in some states but generally legal Adult Stem Cells From adult tissue Self renews many times – May be limited Multipotent – Can be extracted from several tissue types Immune-privileged Many sources No ethics problems Differentiation capacity Rarity in somatic cells Animal pathogens – If cultured Adult Stem Cell Treatments Stem Cell Therapy Patients Report An Overall Improved Quality Of Life….. Adult Adipose (fat) Stem Cell Therapy does Generalized Body Repair with Intravenous Administration. Stem Cell Therapy has been known to help improve a variety of conditions, including: Adult Stem Cells for High Blood Pressure Adult Stem Cells for Diabetes Adult Stem Cells for Joint Pain Adult Stem Cells for COPD (Chronic Obstructive Pulmonary Disease) Adult Stem Cells for Systemic Lupus Erythematosus (Lupus or SLE) Adult Stem Cells for Scleroderma Adult Stem Cells for Erectile Dysfunction Adult Stem Cells for Crohn's Disease Adult Stem Cells for Rheumatoid Arthritis Adult Stem Cells for Osteoarthritis Adult Stem Cells for Vaginal Rejuvenation Adult Stem Cells for Breast Lifts Adult Stem Cells for Musculoskeletal Injury Adult Stem Cells for Hair Restoration Adult Stem Cells for Skin Rejuvenation Adult Stem Cells for many Neurological disorders Stem Cell Therapy patients have also reported higher energy levels, better sleep and overall improved quality of life. No treatment can be guaranteed, and outcomes will vary from patient to patient. Your doctor will conduct an individual assessment to determine the best course of action. Cellular Architecture in the crypt of the small intestine Homeostasis is sustained by crypt-resident multi-potent intestinal stem cells, Crypt Base Columnar (CBC) cells. Pluripotency of these cells are maintained through Wnt signaling supplied mostly by Paneth cells and myofibroblasts. CBCs give rise to highly proliferative progenitor cells: Transit-amplifying (TA) cells (12h cell cycle) TA cells divide, and differentiate to all intestinal lineages as they migrate upward (stimulated by BMP signaling). Enterocytes: absorb and transport Goblet cells: secret mucus to lubricate the mucosal surface Enteroendocrine cells: release peptide hormones Paneth cells: produce bactericidal products (e.g., lysozyme) and Wnt ligand. They descend towards the crypt base. The villi have fully mature cells to perform digestion and resorption functions. Increased Wnt signaling promotes stem cell proliferation. Bone Morphogenetic Proteins (BMPs) inhibits intestinal stem cell proliferation and support full differentiation of the secretory cell lineage. (A reserve stem cell Population) (Actively dividing stem cells) Ref: Krausova, M. and Korinek, V. (2014). Wnt signaling in adult intestinal stem cells and cancer. Cellular Signaling, 26 570-579 43 Abbreviation Full Name Function β-cat Beta-catenin Effector protein Axin Axis Inhibition Protein Destruction complex, brings β-cat and kinases to