Animal Health and Veterinary Profession Neurophysiology PDF
Document Details
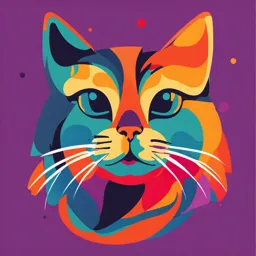
Uploaded by ValuableAntagonist
School of Physiology, Pharmacology and Neuroscience
Dr Richard Helyer
Tags
Related
- Structure of cells & Neurophysiology PDF
- Neurophysiology Summer 2023 Past Paper PDF
- Neurophysiology: Spinal Cord (Summer 2024) PDF
- Neurophysiology Part 17 - Central Control of Movement Summer 2024 PDF
- Neurophysiology III: Reflex Arcs Lecture Notes PDF
- Neurophysiology Part 18 - The Vestibular System Fall 2024 PDF
Summary
These lecture notes cover neurophysiology, focusing on membrane excitability, ionic gradients, action potentials, and the relationship between these concepts and the electrocardiogram (ECG), in the context of animal health and veterinary science.
Full Transcript
Animal Health and the Veterinary Profession Neurophysiology Dr Richard Helyer, School of PPN [email protected] Learning from this lecture & associated tasks Outline what makes a membrane excitable. Explain the basis of the membrane potential. Outline the importance and role of ion channels i...
Animal Health and the Veterinary Profession Neurophysiology Dr Richard Helyer, School of PPN [email protected] Learning from this lecture & associated tasks Outline what makes a membrane excitable. Explain the basis of the membrane potential. Outline the importance and role of ion channels in excitability. Explain the ionic basis of action potential (both nodal and myocardial) with particular reference to the ECG. Summarise the basis of nerve conduction. Any basic textbook; Physiology at a Glance Excitability is through ion channels and ionic gradients The gradient is a property of the cell membrane and the internal and external environment typically electrochemical – chemical concentration gradient but an electrical gradient as well (charge differences across the membrane; capacitance – ability to ‘store’ charge) a gradient predisposes to the possibility of flow, if there’s a way for the ion to move through the membrane – through an ion channel Na+ 145mM K+ 4mM Ca++ 2.5mM Na+ 20mM K+ 150mM Ca++ 0.0001mM OUT IN plus lots of other ions and charged molecules Ion channels allow current flow If an ion channel opens, ions (current) can flow across a membrane a membrane with no open channels is resistant to flow increasing the ‘open probability’ of channels increases flow (conductance) Ohm’s law V=IR, so I=V/R driving force) (I = current, R = resistance, V here is the gradient or channels closed K+ channels open Na+ Ca++ we can make measurements of this – electrophysiology, some research vets do this Ion channels Have a number of key properties membrane proteins with properties of enzymes (environment must be correct etc) can be highly selective, but not always can be ‘modulated’ can activate, deactivate and inactivate (but don’t always do all those things) may be receptor proteins transport is ‘passive’, but involves a gradient Equilibrium potentials We already know that ions can move, or be impeded, due concentration and electrical differences across the membrane. If one ion can flow (selectively permeable membrane), will it flow forever? As an ion moves, the balance of electrical charge across the membrane changes. This changes the ‘driving force’ for the ion. The ion reaches a point of equilibrium, the ‘equilibrium potential’ Equilibrium potentials under physiological conditions K+ -95mV Na+ +61mV Ca++ +137mV Cl- -65mV which helps predict this, the shape of the nerve action potential Calculating equilibrium potentials Nernst Equation Where R = Gas constant (8.31 J/mol/OK) T = Temperature (OK) zK = ionic charge F = Faraday constant (9.6x104 C/mol) which simplifies, at physiological conditions (for a monovalent positive ion) to: EmV = 61.5* x log10 [ion]out [ion]in (just appreciate the simplified one) Task 1 - calculate equilibrium potentials a) Using the simplified Nernst equation below, and the [K+] values from previous slides, calculate EmV for K+ under normal physiological conditions EmV = 61.5* x log10 [ion]out [ion]in b) Now, assume that the animal has become hyperkalaemic. Using a [K+] of 50 mM for the ECS (out) recalculate the EmV for K+. c) What does this imply if the animal were accidently given a bolus of KCl solution IV (also see task 4)? All cells have a membrane potential although some are less excitable than others The Goldman (GHK) equation predicts membrane potential which, for the 3 main permeant ions under physiological conditions is: What can vary a lot, here? Permeability! How is it determined if a channel is open or not? Channels are ‘gated’ ligand-gated, eg. acetylcholine receptor, NMDA-receptor – lots more later voltage-gated, eg. Na+ and K+ channels in nerve cell membrane Depending on when open, underlie membrane depolarisation or repolarisation Task 2 – define these two terms before you proceed The ‘state’ of the sodium channel cycles membrane at rest, channel deactivated activated after initiation of AP absolute ‘refractory period’ – ions cannot pass inactivation – quite rapid Ionic current flow underlies the nerve (nodal) action potential Task 3 – before proceeding with this lecture, try and describe the states of the membrane at 1,2,3 & 4 and suggest what ions may flow at each point Choose from: depolarisation membrane at rest repolarisation Na+ in or out K+ in or out no net flow Initiating an action potential AP is a ‘positive feedback’* event – opening of Na+ channels Na+ channels are voltage-gated – sufficient open to initiate an AP when membrane potential reaches threshold may take one or more ‘events’ to reach threshold – ‘all or none’ * most physiological events are ‘negative feedback’ – in homeostatic mechanisms Current flows underlie the nerve action potential Movement of Na+ ions towards an equilibrium underlies part of the nerve action potential requires opening of Na+ channels allows Na+ to enter cell – membrane depolarisation Na+ will move* down the concentration gradient, towards equilibrium the movement changes the balance of charges either side of the membrane the Na+ ions are moving towards an equilibrium – their equilibrium potential *note ‘flow’ is a bad word, suggests movement of more ions than actually happens Current flows underlie the nerve action potential The cell membrane depolarises during the AP, then repolarises Na+ ions move towards equilibrium, but process terminates the change in membrane potential to ‘more +ve’ starts another process opening of K+ channels : ‘voltage-gated’ channels process of membrane repolarisation So channel state underlies the stages of the AP Resting/deactive Activated 1 2 3 Inactivated Not all channels do this- most K channels activate/deactivate) but a very important process in Na channels Mapping the AP to ion flux When Na channels inactivating, K channels are still opening! But how much (or far) will flow of an ion move the membrane? - in theory, to the equilibrium potential, E, eg. Ena for the permeant ion in question Remember - ionic conductances in the AP key processes are: membrane depolarisation membrane repolarisation and repeat…. Action potentials are not only important in nerves Nerve and muscle – skeletal, cardiac, smooth Cardiac APs underlie contraction of heart muscle Stages of the myocyte action potential Phase 4: the resting membrane potential, largely determined by permeability to K+ Phase 0: activation of voltage-gated Na+ channels, inward current moves Vm towards ENa Phase 1: early repolarisation due largely to inactivation of Na+ channels Phase 2: ‘plateau’ phase; inward current through slowly activating and inactivating voltage-gated Ca++ channels. Phase 3: repolarisation phase; inactivation of Ca++ channels and increase in permeability to K+ Shape, size and duration different in different parts of the heart Action potentials & the ECG Task 4 – why might injection of a bolus of KCl lead to heart failure? Initiating an action potential in a nerve e.g. motor nerve or Vagus nerve An action potential is a rapid, all or none change in the membrane potential followed by a return to resting membrane potential. In nerve cells, but also other tissues including muscle. Triggers a further event, and/or propagates down a nerve – at the same size and shape. In a nerve cell, usually initiated at the ‘axon hillock’ Soma Terminal arborisation excitatory An initiating event must place – some form of stimulation or receptor event on the membrane signals from other nerves via synapses, and external stimuli there can be excitatory and inhibitory inputs inhibitory Propagation of the AP - initiation initiation ‘event’ ‘passive’ spread initiates next openings direction of AP ‘travel’ Propagation of the AP – repeated firing another AP could follow at some point if initiated – channels closed (deactivated), membrane excitable frequency of APs can encode a signal, but frequency is limited – until excitable again, max ~ 1kHz Speed of the action potential propagation -rapid We know Na+ channel properties underlie AP propagation in nerves But what about speed? speed (normally) depends on size of axon, and how ‘leaky’ it is wide axons are less resistant and if there are less leaks, allows wave of depolarisation to move ahead further myelination allows increase in speed in same size neurone Some pain is conducted by small fibres, block well with local anaesthetics