Organisation of Genes in Prokaryotes and Eukaryotes PDF
Document Details
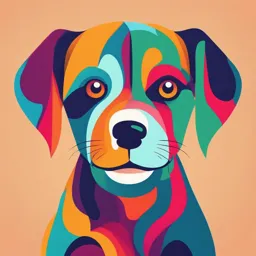
Uploaded by GlisteningPsaltery
Tags
Summary
This document provides an overview of the organization of genes in prokaryotes and eukaryotes, including the roles of genes and non-coding DNA. It explains concepts like genes, alleles, and the different types of non-coding functional RNA genes.
Full Transcript
Organisation of Genes in Prokaryotes and Eukaryotes The hereditary nature of every living organism is defined by its ‘genome’. The genome consists of a long sequence of nucleic acid that provides the information needed to construct an organism. A genome consists of the ent...
Organisation of Genes in Prokaryotes and Eukaryotes The hereditary nature of every living organism is defined by its ‘genome’. The genome consists of a long sequence of nucleic acid that provides the information needed to construct an organism. A genome consists of the entire set of chromosomes for any particular organism. It, therefore, comprises a series of DNA molecules (one for each chromosome), each of which contains many genes. The genome itself does not perform any function, rather a sequence of nucleic acids determines the hereditary features. By a series of interactions, the DNA sequence produces all the proteins of an organism at an appropriate time and place. The genome contains the complete set of hereditary information for any organism. Physically, the genome may be divided into a number of different DNA molecules or chromosomes. The genome may be defined as the sequence of DNA of each chromosome. Functionally, the genome is divided into genes. Genes Genes are the functional units of the genome. They are the hereditary units consisting of DNA sequences that occupy a specific location on a chromosome, which are called a ‘genetic locus’, and determine a particular characteristic in an organism. A gene is a sequence within the nucleic acid that codes for a single protein. Each of the discrete nucleic acid molecules of the genome may contain large number of genes. The number of genes varies in different organisms; it may vary from about 500 genes (for mycoplasma) to as many as 40,000 genes (for human beings). Genes hold the information to build and maintain an organism’s cells. All organisms have many genes corresponding to various biological traits such as eye colour, blood type, increased susceptibility to diseases and the thousands of basic biochemical processes that comprise life. Gene Organization A gene is a sequence that produces a single strand of another nucleic acid, RNA, with a sequence that is identical to one of the two polynucleotide strands of DNA. In many cases, the RNA is successively used to direct the production of proteins; in other cases, they are functional end products; for example, rRNA and tRNA genes. Thus, a gene is a sequence of DNA that codes for RNA; however, in protein-coding regions or structural genes, the RNA successively codes for proteins (Figure 2.1). A gene may exist in alternative forms. An ‘allele’ is one of two or more versions of a gene. Every individual inherits two alleles for each gene, one of paternal origin and the other of maternal origin. The alleles of a gene are the different forms that are found at its locus. ‘Cistron’ is the same as the gene. Basically, cistron describes a stretch of DNA that functions as a unit to give rise to an RNA or protein. Many bacterial mRNAs are ‘polycistronic’, i.e., a single mRNA molecule includes the coding region for several proteins that function together in a biological process. For example, the mRNA encoded by lac operon. In contrast, most eukaryotic mRNAs are ‘monocistronic’, i.e., each mRNA molecule encodes a single protein. Non-coding DNA Non-coding DNA describes the DNA sequences that do not encode for protein sequences. In eukaryotes, a larger percentage of the genome is non-coding DNA. The proportion of non-coding DNA in an organism varies greatly among various species. Much of the non-coding DNAs do not have a known biological function; therefore, they are referred to as ‘Junk DNA’. However, many types of non-coding DNA sequences are involved in transcription and translation regulation of protein-coding sequences. Many non-coding sequences have undetermined functions. Approximately 98 per cent of the human genomes are non-coding, that is, they do not encode protein sequences. An organism’s evolutionary complexity is related to the overall size of the genome and the amount of non-coding DNA; however, there are exceptions. For example, the genome of the pufferfish (Takifugu rubripes) is only one-eighth of the size of the human genome; however, it has a comparable number of genes. Most of the variations in genome size are seen in the non-coding DNA. These extensive variations in nuclear genome size among eukaryotes are known as ‘C-value enigma’ or ‘C-value paradox’. The total amount of DNA contained in a haploid genome is sometimes referred to as the ‘C-value’ for the species. Types of non-coding DNA Non-coding functional RNA genes Non-coding functional RNA genes are the gene sequences of RNA molecules that are not translated into proteins; for example, rRNA, tRNA and microRNAs. Cis and Trans regulatory elements Cis and Trans regulatory elements are the sequences that control gene expression; for example, promoter and enhancer sequences in eukaryotes, and operator and repressor protein-binding sequences in prokaryotes. Introns Introns are the non-coding regions of a gene. They are the intervening sequences of an mRNA and are not translated into protein sequences. Pseudogenes Pseudogenes are the DNA sequences that are related to the known genes that have lost their proteincoding ability or the genes that are no longer expressed in the cell. Pseudogenes, which are also called ‘genomic fossils’, are non-functional due to mutations. ‘Repetitive DNA sequences and telomeres’ (repetitive DNA at the end of a chromosome) are also non-coding sequences. Functions of non-coding DNA sequences Many non-coding DNA sequences have important biological functions. Disease-causing genetic variants lie in the non-coding DNA. Short, specific sequences of non-coding DNA have been discovered, which give rise to non-coding RNA such as microRNA and siRNA. These RNAs function as regulatory elements and have also been linked to diseases in humans. Non-coding DNA are also associated with chromosome structure, centromere function and homologue recognition in meiosis. Non-coding DNA sequences act as gene regulators, controlling the expression of genes Prokaryotic Gene Organization The bacterial genomes are organized into definite bodies. The genetic material can be seen as a fairly compact clump or series of clumps that occupies about a third of the volume of the cell and is called the ‘nucleiod’. Protein HU is a dimer that condenses DNA, possibly wrapping it into a bead-like structure. It is related to IMF (integration host factor) another dimer which has a structural role in building a protein complex in specialized recombination reactions. Another protein, protein H1 (also known as H–NS), binds DNA, interacting preferentially with sequences that are bent. The nucleiod consists of approximately 80 per cent DNA by mass. Bacterial DNA has independently coiled domain. Each domain consists of loop of DNA; the ends of which are secured in some unknown way. There are nearly 100 such domains per genome. Each domain consists of 40 Kb (13 μm) of DNA, which is organized into a compact fibre. The existence of separate domains could permit different degrees of supercoiling to be maintained in different regions of the genome. The 1-mm-long DNA molecule of E. coli chromosome is contained within cells that are only about 2 μm long and about 0.5–1 μm wide. This is possible because several mechanisms operate to compact E. coli chromosome, so that it can fit inside the bacterial cell. For example, the large volume filled by free DNA is due to charge repulsion between positively charged phosphate groups. In the cell, this effect is reduced by associating DNA with positively charged polyamines, such as spermine or spermidine, which shield the negative charges of DNA Gene Organization in Eukaryotes The cells must accommodate the amounts of DNA that are many times longer than the cells they are in. DNA packaging must be very effective while still allowing for DNA replication and transcription to occur. Eukaryotes package DNA in the nucleus into chromatin and chromosomes. Chromatin consists of fibres that contain protein, DNA and small amount of RNA. The DNA in the chromatin is very tightly associated with the proteins called ‘histones’. These have molecular weight between 11,000 and 21,000 and are very rich in the basic amino acids arginine and lysine. There are five major classes of histones found in all eukaryotic cells, namely H1, H2A, H2B, H3 and H4. The histones H3 and H4 are nearly identical in amino acid sequence in all eukaryotic cells; however, H1, H2A and H2B show a lesser degree of homology between eukaryotes. Nucleosomes The DNA in the chromatin is very tightly associated with histones, which package and order the DNA into structural units called nucleosomes. These are fundamental units of organization upon which the higher-order packaging of chromatin is built. Each nucleosome contains eight histone molecules, two copies each of H2A, H2B, H3 and H4. The spacing of the nucleosome beads along the DNA defines a repeating unit typically of about 2,000 base pairs, of which 146 base pairs are bound tightly around the histone core and the remaining base pairs serve as linkers among nucleosomes. The histone H1 is not a part of nucleosome core; however, it is generally bound to the linker DNA. The histone core does not bind randomly to the DNA, but nucleosomes tend to position themselves at certain specific locations. It appears that nucleosomes form where A=T base pairs are abundant, wherever the minor groove of the DNA helix contacts the nucleosome core. Histones contain a flexible amino terminus of 11–37 residues extending from fixed structures of the nucleosome. These termini are called histone tails. The histone tails are required for condensing the DNA from ‘bead on string’ conformation into 30-nm fibre. Several positively charged lysine residues in the histone tails may interact with linker DNA and the tails also interact with neighbouring nucleosomes. The histone-tail lysines, especially those in H3 and H4, undergo reversible acetylation and deacetylation by enzymes. When acetylated, the positive charge of the lysine e¢-amino group is neutralized, thereby eliminating its interaction with a DNA phosphate group. Thus, the greater the acetylation of histone N-termini, the lesser is the chromatin condensation. Histone acetylation is correlated with the resistance of chromatin DNA digestion by nucleases and also plays a role in eukaryotic gene regulation. The 30-nm fibre Wrapping DNA about a nucleosome core compacts it several fold. The total compaction in a chromosome is greater than 10 fold, which provides ample evidence for higher orders of structural organization. Nucleosomes are organized to form a structure called 30-nm fibre. This packaging requires only one molecule of the histone H1 per nucleosome. Organization into 30-nm fibre does not extend over the entire chromosome but is punctuated by the regions that are bound by the sequencespecific non- histone DNA-binding proteins. The structure observed also depends on the transcriptional activity of the particular region of DNA. The regions that are being transcribed are usually in a less ordered state that contains little histone H1. The 30-nm fibre provides approximately 100-fold compaction of the DNA Nuclear scaffold Certain regions of the DNA associate with a nuclear scaffold. The scaffold-associated regions are separated by the loops of DNA with 20,000 to 1,00,000 base pairs. The DNA in these loops may contain a set of related genes. The scaffold itself contains several proteins, notably large amounts of H1 and topoisomeraseII, which is essential for maintaining the chromatin structure Higher organization Evidence exists for the additional layers of organization in eukaryotic chromosomes, each enhancing the compaction multiplicatively. DNA compaction in eukaryotic chromosomes is likely to involve coils upon coils, resulting in the chromatin