A2-A Thread Overview PDF
Document Details
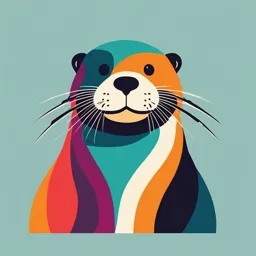
Uploaded by PurposefulNirvana
Tags
Summary
This document provides a high-level overview of the basic physics behind x-ray, CT, and ultrasound imaging, focusing on how these technologies produce images. The document also describes the properties of electromagnetic and pressure waves, highlighting their interaction with tissue.
Full Transcript
Thread A2: X-Ray, CT, Ultrasound Final Thoughts The tools we considered in the modules are summarized in Figure 4. The main point of this high-level review of these tools was to consider the basic physics and how they can be used to produce an imaging result. In all cases, a signal is measured outsi...
Thread A2: X-Ray, CT, Ultrasound Final Thoughts The tools we considered in the modules are summarized in Figure 4. The main point of this high-level review of these tools was to consider the basic physics and how they can be used to produce an imaging result. In all cases, a signal is measured outside of the patient. 1. Planar x-ray. An x-ray source outside the patients beams x-rays through the patient to a sensor on the other side. The different tissues and bone block (absorb) different amounts of the x-rays. The sensor measures how much of the x-ray beam made it though a small volume of tissue. The image is a map of how much the x-rays are blocked and therefore a 2D map of tissues and bones. a. X-ray Computed Tomography (CT). Rotates the x-ray equipment to take images all around the patient. The images are combined to create a 3D image. 2. Ultrasound. A sound (pressure) wave is sent into the tissue and the reflection is measured. The image is a 2D map of reflection depths from the sensor. Different tissues will create different reflections. The image is then a depth map of the tissues. Note that ultrasonic waves are pressure waves and are different than the electromagnetic waves in the other sensing modes. Figure 1: Summary of imaging tools (Image Tburg, 2023). Waves The commonality between x-ray, CT, and Ultrasound is that they all use the interaction of a wave with tissues to create an image of the anatomy. X-ray and CT use the same electromagnetic waves and ultrasound imaging uses sound waves. It is important to distinguish the two types of waves: • Electromagnetic Waves, which include light and x-rays (see Figure 2), propagate (move, travel) through materials as perpendicular electric and magnetic field waves. See the top left area in Figure 5. We didn’t mention the two components so just consider as a single traveling wave. Electromagnetic waves are modeled as both a particle and a wave. Version 11/7/2023 Page 1 of 10 • Figure 2: Electromagnetic Spectrum Pressure Waves, sound waves (see Figure 3), propagate (move, travel) through materials as moving areas of higher and lower pressure. See the bottom left area in Figure 5. Acoustic waves are modeled as a wave. Figure 3: Acoustic Spectrum The terms we use to describe waves are similar as shown in Figure 5. For both types of waves: • • • The wavelength (λ) describes how the wave varies in a material or tissue, it is a measure of how often the wave repeats in space (in the material or tissue). The velocity is how fast a point on a wave moves through a medium, such as air or a tissue. The frequency is derived from a plot of amplitude versus time. Here is the subtle part, it is drawn from the perspective of an observer who doesn’t move and watches the waves go by – they see the wave go up and down. The period is the amount of time before the wave repeats and the frequency is just the inverse of the period. Version 11/7/2023 Page 2 of 10 Figure 4: Main properties of electromagnetic waves (top) and pressure (sound) waves (bottom) in the spatial domain, moving through a material and a plot of amplitude in the time domain. All waves follow similar interactions with materials including: • • • • • • • • Constant velocity in a specific medium Absorption Reflection Doppler Shift Scattering Refraction Diffraction Destructive Interference More Thoughts about Waves To help appreciate the idea of a traveling wave, here is a photo of some ocean waves traveling toward an abandoned peer on the shore in Figure 3. Your experience probably tells you that the wave peaks are moving past the pilings of the old peer and toward you, the wave is traveling toward you. The pilings, the remining posts from the peer covered in algae, are numbered (as well as possible) with the labels showing each 5th piling. In the scene the pilings are fixed observers. We can draw a distance plot to show how the wave is distributed along the pilings as seen in the top, right plot of Figure 3. With a little imagination you can see peaks of the wave at pilings 1, 10, and 20. The wavelength is the physical distance between waves in the distance plot. Here the wavelength is about the distance between 10 pilings. Using more imagination, the second plot shows that after a short amount of time (less than a second) the peak that was at piling 10 has moved, traveled forward, to peer 15. The wave is traveling in the medium (ocean water). The wavelength is measured on the distance plot. Version 11/7/2023 Page 3 of 10 Figure 5: Waves traveling on ocean toward an old peer on the shore. (ocean image , https://pixabay.com/photos/sunset-monolithic-part-of-the-waters-3076772 19-2023) The second plot we are always interested in is the amplitude versus time plot. Here we choose a fixed observer and measure the height of the wave as it goes past the fixed observer. We will use piling 10 as our fixed observer and measure the wave height on the piling at different times. One point we can see from the photo, the wave is at maximum height at this time at piling 10, we will put this at the start of our time plot. We keep plotting heights as fast as we can to complete the rest of the plot. One point we talked about in Figure 3, imagined the distance plot a short time after the photo when the peak has moved from piling 10 moved to piling 15, and the lowest part of the wave is now at piling 10. Remember, the time plot is only showing what a fixed observer sees (at piling 10). The period, and hence frequency, is measured on the distance plot. Figure 6: The wave measured at the fixed observer Piling 10. (ocean image , https://pixabay.com/photos/sunset-monolithic-part-of-the-waters-3076772 19-2023) The Basics of X-ray and CT The x-ray process is shown in Figure 7. The x-rays are created in three-part process. Note that the electric current and electric field (the force that accelerates electrons) set the properties of the x-ray waves. The fact that different tissue types absorb x-rays differently means that we can “see” the Version 11/7/2023 Page 4 of 10 different tissues. Note that by tradition, the lightest area on an x-ray image is where the fewest x-rays get through the patient and the darkest areas are where there is little tissue. Bones appear very bright because they absorb a lot of x-rays and the areas outside the patient are black because all x-rays reach the sensor. Figure 7: Overview of x-ray process Both x-ray and CT use a similar process but the energy, frequency, and exposure time are adjusted for any specific application. CT uses a series of many x-ray images. All About Making an Estimate of the Anatomy We used the term "projection" to mean that when you shine the x-rays through an object onto the sensor (the plane below), this is the image. It is important to remember that in the x-ray image, we lose all depth information because any pixel in the image is an aggregation of what the beam saw going through that part of the patient. The CT process is used to overcome this limitation, to create an estimate of the patient anatomy from a series of x-ray images (projections). We use "estimate" to emphasize what that each x-ray projection has lost depth information about the patient. We would love to take a single projection and reverse the xray process to know about the patient anatomy, including depth information but this is not possible, the depth information is gone. However, with two x-ray projections from different angles, we can start reclaiming some of the depth information and better recreate the patient, it is not perfect but better than with one projection. The more projections from different angles the better we can reclaim the depth information to create an estimate of the patient. Back-projection is the technique for taking the projections and trying to recreate the anatomy that was observed in the x-rays. In a sense, we would like to "undo" the projections to get back the original anatomy; we can never to do this perfectly so our CT reconstruction is an “estimate”. Version 11/7/2023 Page 5 of 10 X-Ray Beam Shape Figure 7 shows the x-ray beam shape as a cone. Ideally the x-rays would be parallel going into and exi�ng the subject, but the x-ray tube is more like a point-source and the x-rays spread out from the point in a cone. This can cause some distor�on in the planar projec�on. Maybe an analogy is using a pencil to trace your hand on a piece of paper. If the pencil stays ver�cal, then the drawing should be a good representa�on of your hand. However, if you hold the pencil at an angle things get distorted. In the same way, we want the x-ray beams going from top to botom without an angle. Unfortunately, the x-ray source is like a light bulb and the rays spread out from the bulb as illustrated in Figure 8. If you stand close to a lightbulb, your shadow on the wall is huge but if you can get further away the rays are more parallel and your shadow is closer to the correct size. Looking at the picture with shadows from a light source, if the light rays are parallel then the shadows would be the same from both loca�ons. Figure 8: Difference in the projected image between parallel and fan shaped beams. The Challenge of CT Imaging The challenge of CT imaging is to use a series of flat projections to estimate the 3D anatomy of the patient. Just to highlight this challenge Figure 6 shows that a series of photographs taken from different angles around a statue can be used to make a 3D image of the statue. If you look at any single photograph, you can’t measure depth - while the shades and contours coupled with your experience of the head may lead you to “perceive” depth, there is no depth information in the photos. However, there is usable length and height information. For example, as illustrated in Figure 7, I could use the front view to estimate the features of the nose, like width, and a side view to measure the other features, like length, from which I could make a decent 3D drawing of the nose. The imaging software takes this approach to automatically create the 3D model. All this to say, there is power in having multiple planar projections of a solid and we harness this power to take and combine multiple x-ray images (planar projections) to create 3D estimates of patient anatomy. Version 11/7/2023 Page 6 of 10 Figure 9: On the left, a series of images taken around a statue. On the right side they have used a computer software to turn the images into a 3D model. (Image from https://www.youtube.com/watch?v=GaYfpGcXxmA) Figure 10: A single photograph, a flat projection, contains width and height information but no depth information. Combining different views of the object can be used to estimate depths. The Simplicity of Ultrasound The basic tenet of ultrasound imaging is that tissue will conduct, reflect, and scatter sound waves according to the type of tissue. Version 11/7/2023 Page 7 of 10 Figure 11: Overview of ultrasound process The piezoelectric actuator is an interesting device. Piezoelectric materials, certain crystals and ceramics, produce electric charge, and hence a voltage, in response to an applied force. As shown in the top path of Figure 10 the piezoelectric effect results when the material is distorted, either compressed or stretched, to produce a voltage. The converse piezoelectric effect, shown in the bottom path of Figure 10, causes a distortion of the material when a voltage is applied. Figure 12: Two behaviors of a piezoelectric material. Clinical ultrasound displays the information collected about the reflections as the B-Mode and M-Mode images and the A-Mode plot sketched in Figure 8 • B-Mode shows a 2D map of the reflections. o M-Mode shows progression of one line of the B-Mode over time. If nothing in the patient moves, then the M-Mode is a constant image. o A-Mode shows the amplitude (intensity) of one line of the B-Mode at a single time instance. Version 11/7/2023 Page 8 of 10 Figure 13: Three mode of the modes of ultrasound imaging with no motion in the patient. The gray circle represents an organ. The Impact of Imaging Measurements Returning to the basic purpose of biomedical imaging illustrated in Figure 13, we reiterate the value clinical proposition that the imaging process must create more treatment benefit than the potential harm it may cause. All imaging modes have some potential risk and the benefit gained in treating a patient must be balanced against that risk. That is of course easier to say than to practice! Figure 14: Biomedical imaging tools must provide the clinician with useful information about the patient health that could not be obtained cheaper or safer by other means. (Image Tburg, 2022) One principle that can help guide the decision-making process is the “ALARA” approach, where ALARA stands for “as low as reasonably achievable”. To parse this phrase suggests: • • “low” directs us to o seek to perform the minimum number of tests o choose the least impactful mode (ultrasound, x-ray, …) o minimize exposure (time, energy, frequency) “reasonably achievable” reminds us Version 11/7/2023 Page 9 of 10 o o zero risk is not possible there is a clinical need to be fulfilled. A test that doesn’t answer the clinical question has created an unnecessary exposure, e.g. trying to go too “low” and not getting the needed information. In summary, “ALARA” means we need to seek “right the first time” in biomedical imaging. Version 11/7/2023 Page 10 of 10