A Textbook of Veterinary General Pathology PDF
Document Details
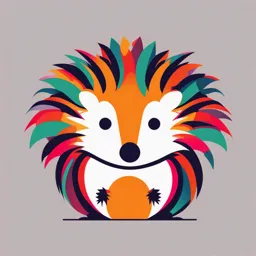
Uploaded by StrikingEducation6984
2007
J. L. Vegad
Tags
Summary
This textbook covers veterinary general pathology, a study of structural and functional changes in animal cells, tissues, and organs. The second edition provides an updated perspective on molecular mechanisms of disease processes. The book incorporates recent insights, numerous illustrations, and a new chapter on concretions, according to the Veterinary Council of India (VCI) syllabus.
Full Transcript
A Textbook of Veterinary General Pathology J. L. VEGAD International Book Distributing Co. I / A Textbook of Veterinary General Pathology (Also useful for Medical and Dental students) J. L. VEGAD Former Profe...
A Textbook of Veterinary General Pathology J. L. VEGAD International Book Distributing Co. I / A Textbook of Veterinary General Pathology (Also useful for Medical and Dental students) J. L. VEGAD Former Professor and Head Department of Pathology College of Veterinary Science and Animal Husbandry Jabalpur - 482001, M.P., India International Book Distributing Co. (Publishing Division) Published by INTERNATIONAL BOOK DISTRIBUTING CO. (Publishing Division) Khushnuma Complex Basement 7, Meerabai Marg (Behind Jawahar Bhawan) Lucknow 226 001 V.P. (INDIA) Tel.: 91-522-2209542,2209543, 2209544,2209545 Fax: 0522-4045308 E-Mail: [email protected] Second Edition 2007 First Reprint November 2008 ISBN 978-81-8189-181-5 ©Publisher All Rights Reserved No part of this publication may be reproduced, stored in a retrieval system, or transmitted, in any form or by any means, electronic, mechanical, photocopying, recording or otherwise, without the prior written permission of the publisher. Composed & Designed at: Panacea Computers 3rd Floor, Agrawal Sabha Bhawan Subhash Mohal, Sadar Cantt. Lucknow-226 002 Phone: 0522-2483312, 9335927082 E-mail: [email protected] Printed at: Salasar Imaging Systems C-7/5, Lawrence Road Industrial Area Delhi -110 035 Tel. : 011-27185653,9810064311 Preface to Second Edition The widespread popularity of the first edition, both with students and teachers, has prompted me to bring out this Second Edition. I do hope this edition proves equally useful and is received with the same fervour. Since the first edition was published in 1995, there have been further spectacular advances in our understanding of the molecular mechanisms involved in the pathogenesis of disease processes. This accelerating pace of knowledge necessitated revision and updating of the book. The second edition has been extensively revised and most chapters are completely rewritten. As such, it contains the latest information on molecular pathology. The changes in particular include: An effort has been made to incorporate the most recent information on the molecular mechanisms of disease processes. For an easy grasp of the complicated molecular mechanisms, a large number of illustrations have been added, 79 in all, in the form of flow charts, line diagrams, and diagrammatic representations of pathological processes. Keeping in view the Veterinary Council of India (VCI)' s syllabus, a new chapter on 'Concretions' (Chapter 12) has been added. Also, in view of the VCI syllabus, a new sub-topic I Avian Inflammation' has been added in the chapter on 'Inflammation' (Chapter 4). The main points are given in bold type. In the Index, where there are more page numbers for the same topic, the number that covers the main discussion is shown in bold. As the book deals with basic pathology, it will be very useful for medical and dental students. I am grateful to Mr. Suneel Gomber, Manager, International Book Distributing Co., Lucknow, for the publication of this book. I extend my sincere thanks to Dr. Madhu Swamy for going through the typescript. Dr. Priti Mishra checked the index, while Mr. Anand Parmar and Mr. Vijay Parmar of Jabalpur Graphics were most generous in extending help relating to computer and other work. I am thankful to them all. I am especially grateful to my wife Nita and eldest brother Amrit Lal Vegad for the moral support and for their faith in me and my task. J. L. Vegad "This page is Intentionally Left Blank" Preface to First Edition Recent years have witnessed explosion of knowledge in molecular biology, and consequent thereupon, in the field of molecular pathology. The study of pathology is no longer confined to morphological alterations. Molecular mechanisms involved in the pathogenesis of diseases are being continuously unraveled. No area of pathology has remained untouched by the molecular strides. Since this book deals with General Pathology, that is, study of the basic pathological processes, an attempt has been made to bring out the most recent concepts of molecular mechanisms. For, it is the conceptualization of the underlying principles that is paramount in laying the foundations of systemic pathology, and in fact, medicine as a whole. The book is intended for both undergraduate and postgraduate students. The underg:aduate may, at places, find the test a little too extensive. They are advised to skip these portions. However, should they find ~e new information rewarding, the idea is to make it readily available at one place. I only hope I could succeed in transmitting the excitement of the remarkable insights gained into the biomolecular origins of disease processes. The complex mechanisms have been explained in a simplified way using line diagrams, so that they are readily understood. Also, before dealing with the pathogenetic mechanisms, physiological, biochemical, and other related aspects have been briefly reviewed, for an easy comprehension of the subject. Thus, discussion of free radical mediation of cell injury is preceded by a consideration of what free radicals are; or that of mechanisms of healing by an examination of extracellular matrix, or a discussion of thrombosis by a brief consideration of haemostatic mechanisms. Another feature of the book is that, wherever considered appropriate, aspects of human pathology have been narrated side by side. The text will therefore act as a useful exercise in comparative pathology. As such the book may serve as a good reference for medical, dentistry, and zoology students. In fact, it should prove useful to workers in all branches of science who wish to learn about the basic disease processes. I am grateful to Dr. S. K. Ranjhan who encouraged me to write this book and to Shri C. M. Chawla of Vikas Publishing House for its publication. It is a pleasure to acknowledge the help of my colleagues v - Dr. A. K. Katiyar, Dr. H. K. B. Parekh, Dr. R. G. Dhawedkar and Dr. B.c. Sarkhel- for their many contributions. Finally, my deepest gratitude to members of my joint family for their patience and understanding. I am especially grateful to my wife Nita and my eldest brother Amritlal Vegad for the moral support, and for their faith in me and my task. J. L. Vegad, Department of Pathology, College of Veterinary Science & A.H., Jabalpur - 482001 vi Abbreviations AD = Anno Domini ADCC = Antibody-dependent cell-mediated cytotoxicity BC Before Christ BCR = B-cell receptor BM = Basement membrane CD Cluster of differentiation CTL Cytotoxic T- cell DIC Disseminated intravascular coagulation DTH = Delayed-type hypersensitivity ECF Eosinophil chemotactic factor ECM Extracellular matrix EGF Epidermal growth factor F French FGF = Fibroblast growth factor G = Greek IFN = Interferon Ig = Immunoglobulin IL Interleukin IVP Increased vascular permeability L = Latin LPS Lipopolysaccharide LT = Leukotriene LX Lipoxin MAC Membrane attack complex MHC Major histocompatibility complex mm = Millimetre (thousandth part of a metre) MPS Mononuclear phagocyte system vii ~m Micron / micrometre (one millionth of a metre) NCF Neutrophil chemotactic factor NK cell = Natural killer cell nm = Nanometre (one billionth of metre) NO = Nitric oxide PAF Platelet activating factor PDGF = Platelet-derived growth factor PG = Prostaglandin PGI2 Prostacyclin R = Receptor, e.g., IL-2R TCR = T- cell receptor TGF = Transforming growth factor TNF = Tumour necrosis factor TX Thromboxane VEGF = Vascular endothelial growth factor vWF von Willebrad factor viii Contents Chapter 1 Introduction 1 Chapter 2 History of Pathology 7 Chapter 3 Cell Injury and Cell Death 19 Chapter 4 Inflammation 105 Chapter 5 Tissue Repair (Healing) 183 Chapter 6 Haemodynamic Disorders 217 Chapter 7 Cellular Adaptations of Growth and Differentiation 267 Chapter 8 Neoplasia 277 Chapter 9 Diseases of Immunity 397 Chapter 10 Nutritional Diseases 469 Chapter 11 Physical and Chemical Injuries 501 Arrow-1.tif Chapter 12 Concretions 515 Chapter 13 Genetic Diseases 521 Chapter 14 Disturbances in Development 539 Chapter 15 General Pathology of Infectious Diseases 547 Index 563 ix "This page is Intentionally Left Blank" Chapter 1 Introduction P athology is the study of disease (G. pathos = suffering or disease + logos = study). Disease is that condition in which the individual suffers from discomfort (L. dis = not + ease, i.e., not at ease). Just as anatomy is the study of the structure and physiology its functions, pathology is the study of structure and function of the body in disease. Pathology therefore deals with the structural and functional changes in cells, tissues, and organs that underlie disease. It is a discipline that bridges basic science and clinical practice. To understand the structural and functional changes, pathologists use modern microbiological, immunological, and molecular techniques. The object of pathology is to acquaint the student with the changes occurring in tissues as the result of disease. By studying pathology the student learns to apply the knowledge of basic subjects, and ~s one progresses to junior and senior years, one has a better understanding of the changes that take place within an animal as the result of disease. The student is then able to visualize tissue changes and appreciate why the symptoms appeared. Thus, pathology serves as a bridge between the basic subjects of anatomy, physiology, and biochemistry on the one hand, and medical, surgical, and gynaecological treatment on the other. In other words, pathology is that correlating study by which the pre-pathology courses are co- ordinated so that a better understanding of clinical subjects is possible. Pathology is therefore central to an understanding of disease. It explains how altered structures produce lesions and disordered functions symptoms. Thus, pathology occupies a pivotal position in the study of veterinary medicine. Earlier, pathology was mostly confined to the study of morphological changes, both gross and microscopic. With advances in knowledge, immunological and molecular mechanisms that underlie the morphologica1changes became an integral part of modem pathology. Remarkable progress in molecular pathology has now clearly established that disease is produced first at a molecular level, that is, biochemical or molecular lesion. This, in turn, induces structural changes, first at an electron microscopical level 1 Textbook of Veterinary General Pathology (ultrastructural lesion), then light microscopical lesions develop, and these when extensive, produce gross lesions. Pathology is divided into "General Pathology" and "Systemic or Special Pathology". General pathology is concerned with the basic reactions of cells and tissues to injurious stimuli. The systemic or special pathology is the application of these basic reactions to the various body systems, or to various specific diseases.This book deals with the basic principles of general pathology. Pathology covers five aspects of a disease process: (1) its cause (aetiology), (2) the mechanisms of its development (pathogenesis), (3) structural changes produced in cells and organs (morphological changes), (4) the functional consequences of the morphological changes (clinical significance), and (5) result or termination. 1. Cause (Aetiology): There are two major classes of aetiological factors: genetic (intrinsic) and acquired (extrinsic) (infectious, nutritional, chemical, physical, etc.). However, the concept of "one cause - one disease" is no longer adequate. Genetic factors clearly affect environmentally induced diseases, and the environment may have profound influence on certain genetic diseases. 2. Pathogenesis: Pathogenesis is the progressive development of a disease process from the time it is initiated to its conclusion, in recovery or death. In other words, pathogenesis refers to the sequence of events in the response of the cells or tissues to the causal agent, from the initial stimulus to the ultimate expression of the disease. The study of pathogenesis remains pivotal to the subject of pathology. 3. Structural and functional changes: These are the morphological and associated functional alterations in cells or tissues. Lesion is the macroscopic or microscopic alteration occurring in the tissue as the result of injury, e.g., fracture in a bone is a lesion. Pathognomonic lesion is an alteration that indicates without doubt the cause of a particular disease. For example, button- shaped ulcers of the large intestine in swine fever; zebra markings of the large intestine in rinderpest; punched-out ulcers in the abomasum in theileriasis; and Negri body in the cells of the hippocampus and cerebellum in rabies. Disturbance in function may show itself as symptom of disease. Thus, symptom is a clinical sign (fever, swelling, diarrhoea, vomiting, lameness, 2 Introduction etc.) manifested by the individual as the result of tissue changes. 4. Clinical significance: The structural and functional changes influence normal functions and determine the clinical features (symptoms and signs), course, and prognosis of disease. Clinical pathology involves use of laboratory methods by clinicians to help them in arriving ata diagnosis. It includes the examination of blood, urine, faeces, exudates, skin scrapings, and biopsy material. Clinical pathology is so named because some of the work, especially the collection of material, is done at the bedside of patient (G. clinics, klinike = bed). Diagnosis is the art or act of identifying a disease from its signs and symptoms, and also through various laboratory tests. In other words, diagnosis is to know or recognize disease states through clinical signs (G. dia = through + gnos = to know). Prognosis is the prospect of recovery as expected from the usual cause of disease, or peculiarities of the case. That is, forecast about the likely course and outcome of a disease. In other words, prognosis is a knowing beforehand (L. & G. pro = before + gnos = to know), or foreknowledge of the chances of recovery. 5. Result or termination: It occurs in three ways: (1) recovery, (2) death, and (3) invalidism. If the damage produced by the disease is not much and is repaired, the individual recovers. If body defences are overcome and the tissue damage is great, then life cannot be maintained and individual dies. If, on the other hand, the body is not able to completely repair and the extent of injury is not so great as to kill the animal, invalidism occurs. That is, the animal continues to suffer from prolonged ill health, as in Johne's disease or tuberculosis in cattle. Let us also consider a few other definitions, before proceeding to history of pathology in the next chapter. Macroscopic or gross pathology is examination of tissue without the help of a microscope. Microscopic pathology or histopathology is examination of tissue with the help of a microscope, and involves the use of stained tissue sections. Chemical pathology is the study of chemical alterations of the body fluids and tissue that result from disease. Postmortem examination is examination of an animal after death to establish a disease, that is, cause of its death. Postmortem 3 Textbook of Veterinary General Pathology examination of animals and birds is called necropsy. The term autopsy is used for the postmortem examination of humans. Morbid changes are alterations found in tissues at necropsy as a result of disease. Biopsy is examination of tissue removed from the living (G. bios=life) animal to determine the cause of disease. Biopsy is normally performed in a suspected cancer. Syndrome: Syndrome (G. syn = together + drome = to run) literally means' a running together'. Thus, syndrome refers to a group of symptoms that occur together and characterize a particular abnormality, that is, they are characteristic of a certain disease. Health and disease: Health is the condition in which the individual is in complete harmony with its environment! surroundings. Disease is a change in that condition, as a result the individual suffers from discomfort. In other words, disease is a condition in which an individual shows a structural, functional, or chemical deviation from the normal. However, at times it is difficult to determine if an individual is in health or disease. For example, one kidney may be absent. The remaining kidney may become twice its normal size, since it assumes the function of the missing kidney. This change is called compensatory hypertrophy, and if it comes about gradually, the individual remains clinically healthy and continues to lead a normal life. But, pathologically, he is in disease, because absence of a kidney is a structural deviation from the normal. Thus, the pathologist's concept of a disease is not necessarily the same as that of a clinician. Cause (aetiology): Causes are of two types: intrinsic or endogenous, and extrinsic or exogenous. The intrinsic (endogenous) causes of disease, also known as the predisposing factors, are those characteristics (genus, breed, sex, age, etc.) over which an individual has no control and which determine the type of disease present. At the basis of the first three factors, namely, genus, breed, and strain, underlie genetics and heredity. Genus: Examples: Swine fever is a disease of the pig and no other animals are infected with it. Canine distemper is primarily a disease of the dog. It does not affect the horse, cow, sheep, or pig. Breed: Dairy cattle are much more susceptible to diseases than beef cattle. Within the dairy cattle, certain breeds are less resistant to some diseases than others. The same is applicable with certain breeds 4 Introduction of dogs. For example, brain tumours are common in the bulldog breeds. The boxer dog has a very high incidence of brain tumours than other breeds. Strain: Certain strains of animals possess unusual resistance to certain diseases. For example, strains of chickens that have unusual resistance to leukosis can be produced, in contrast to other strains which have a very high mortality rate from this disease. Other examples include hernias in pigs, and calves with hydrocephalus (accumulation of fluid in the ventricles of brain). These problems are observed with greater frequency in certain family of animals than others. Age: Certain diseases are found in a definite age group. Tumours are commonly observed in older animals. Likewise, strangles is a disease of young horses. Caecal coccidiosis in the chicken is a disease of young birds. Sex: Reproductive diseases are more common in the female than in the male. Certain diseases (metritis, mastitis, milk fever) are confined to the female. Nephritis in the dog is 2 to 3 times more common in the male than in the female. In cattle, nephritis is more common in the female. Colour: Cancer melanosarcomas are very common in grey and white horses, but very rare in brown or black horses. Animals with non-pigmented skin are much more susceptible to photodynamic diseases. If pigment is present in the skin, it protects the animal by preventing the sunlight from penetrating the skin, thus inhibiting the chemical reaction (see' photosensitization, Chapter 11). Idiosyncrasy is an unusual reaction to some substance. Some animals exhibit an unusual reaction when a drug is administered and may even die, or show serious manifestations of unusual toxicity. The extrinsic (exogenous) causes of disease are those environmental factors (physical, chemical, thermal, infectious, immunological, nutritional) that are capable of producing disease in the individual. These factors are described in Chapter 3, under "Causes of cell injury". 5 "This page is Intentionally Left Blank" Chapter 2 History· of Pathology H istory of pathology is fascinating. Disease is as old as life itself. In fact, history of pathology (or disease) and history of medicine go hand in hand. That is, they are closely interwoven. Explorations into the past tell us how the present day knowledge was reached. What were the contributions of those dedicated souls who made supreme sacrifices so that the science flourishes, and human and animal lives are saved from the scourge of disease. They tell us how the concept of disease evolved from its most primitive form in the Stone Age to the present day. Who, in fact, were the early physicians, and how did they treat their patients? How steadily the science of anatomy, physiology and pathology progressed. How, indeed the veterinary medicine came into being? While these aspects of the history are deeply engrossing, their detailed accounts are beyond the scope of this book. At times, it has so happened that one single event has changed the course of history and resulted in an entire revolution of medicine. These milestones of medical progress stand out quite clearly as we delve into the past. For example, circulation of blood by William Harvey was an epoch-making discovery, because it explained how nutrients and oxygen are carried to cells in the body. It also explained many other alterations observed in pathology. The work of Leeuwenhoek, Bichat and Miieller opened the field of histopathology. Virchow put forward the concept that all forms of injury start with molecular or structural alterations in cells, in his book on cellular pathology. The study of bacteria by Pasteur, Koch and Klebs demonstrated that these minute organisms were, in fact, the cause of disease. Cohnheim and Metchnikoff described the vascular and cellular changes that occurred in inflammation. Here, however, we shall consider very briefly, somewhat chronologically, only the most outstanding contributions of the glittering.scientific geniuses. Veterinary medicine, through ages, has been closely allied with human medicine, just as it is today. The diseases of animals are often the diseases of humans, and therefore their histories are closely linked. 7 Textbook of Veterinary General Pathology The First Concept of Disease Man, in stone or prehistoric age, was barbarous, brutal and cruel. He hunted animals and ate their flesh; and even indulged in cannibalism (i.e., eating of human flesh by a human). Then, he came to believe in the existence of evil spirits or demons and dreaded them. The study of demons or evil spirits is called demonology'. He further I believed that if man got possessed by a demon, that is, he became a demoniac, then he was taken ill and suffered disease. The cause of disease was considered to be the displeasure of evil spirits, and the treatment was directed toward appeasing them. The demons were even worshipped. Thus originated the first notion about the nature of an illness - the demoniac concept of disease. He further assumed and accepted it as true that the evil spirit used to enter the head. To expel the evil spirit (exorcize), the wild medical man trephined the skull by a sharp long pointed stone. Thus, the skull surgery came into existence. The medicine and treatment were controlled by the witch doctor, who used secret methods, magic, spells, incantations, magical ceremonies, vegetable and animal concoctions and even noise to drive away the evil spirits, and wore hideous dress. Thus, the first physician was a witch doctor. In one of the caves in the Pyrenees mountains, that are between France and Spain, there is a rock painting of a witch doctor. The portrait is believed to have been made some 17 thousand years ago. And, it is from the witch doctor that the medical man of today is descended. The Theological Concept of Disease Then came the theory of the theological cause of disease. About 4000 B.C., in Egypt, embalming (i.e., preservation of a dead body against putrefaction) of thousands of mummies (dead bodies) was carried out. Bodies were taken into the embalming temples, and no one but the priest saw them. Though priests recognized the changes in the organs, they kept the information secret. On the other hand, they put forward the theory that illness was the result of Divine displeasure. That is, if man disobeyed or displeased God, he suffered God's wrath (intense anger) and became ill. By so doing, priests could maintain their theological hold on the people. Thus, the theological concept of aetiology and mysticism (spiritualism) was nourished, and now the priest played the role of a physician. 8 History of Pathology The Humoral Concept of Disease With the arrival of Greek culture, a scientific study of medicine began, and rejection of demon worship occurred. Hippocrates (460- 377 B.C.) was undoubtedly the most outstanding individual in the history of medicine. His impact on medical science was so great he is honoured as the 'Father of Medicine'. He formulated the 'Oath of Hippocrates', concerning the practice and ethics of medicine. His writings formed the basis for the 'humoral theory of disease'. He put forward that the body consists of four humours (four fluids): (i) blood, which was warm and moist like air, (ii) phlegm, which was cold and moist like water, (iii) yellow bile, which was warm and dry like fire, and (iv) black bile, which was cold and dry like earth. The origin of humours was interesting. The blood came from the heart, the phlegm originated in the brain, the yellow bile came from the liver, and the black bile (the most serious of all) came from the spleen. Hippocrates taught that health was due to a correct mixture, a eucrasia (G. eu = well + krasis = mixture) of the four fluids of body, and that disease was due to an incorrect mixture, dyscrasia (G. dys = bad). Hippocrates explained that when phlegm that originated in the brain, begins to gravitate downward and appears at the nose, the individual has a cold. If it gravitates into the lungs, the patient has pneumonia. If it continues to gravitate into the lungs for a long period, tuberculosis is the result. When gravitation extends still lower and reaches the intestine, dysentery occurs. Finally, when the phlegm descends into the rectum, haemorrhoids occur. Diseases of the black bile were considered to be the most serious. Although the humoral theory of Hippocrates seems odd to us today, it served as the basis for medical practice for two thousand years. In fact, historically, it is the most important pathological theory yet propounded. It was not until the end of the nineteenth century that its hold on the medical world was finally broken, when modem physiology, pathology, and bacteriology proved it to be wrong. Development&ln Anatomy, Physiology and Pathology Aristotle (384-322 B.C.), another Greek philosopher, was the originator of modern anatomy and physiology. He dissected many animals and carried out experiments in physiology. Claudius Galen (131-206 A.D.), a Greek physician practising in Rome, contributed greatly to human physiology and also dissected animals. He is considered as 'Father of Anatomy'. Galen is perhaps the greatest 9 Textbook of Veterinary General Pathology medical figure of all times. Even after his death, for thirteen centuries his teachings prevailed. Another important person of this era (period) was Cornelius Celsus (30 B.C. - 38 A.D.), a Roman writer of the first century A.D. Celsus was not a physician, but had a wide variety of interests. He described the four cardinal signs of inflammation - redness, swelling, heat, and pain. First Textbook on Veterinary Medicine A Roman veterinarian, Renatus Vegetius (450-500 A.D.) is credited as being the first to write a textbook on veterinary medicine, known as 'Book of the Veterinary Art'. His concepts of the diseases of animals and their control had great influence on many people, and on the animal industry of that time. Because of the book he wrote, and the impact he had on veterinary medicine, he is considered as the 'Father of Veterinary Medicine'. However, the Roman slave Androc1es, who extracted a thorn from the paw of a lion, is credited as the first veterinarian. Century of Anatomy Soon D.ivine displeasure as the cause of disease began to disappear, and people were beginning to resort to surgery, medicine, and common sense. Gradually there was elimination of superstition and fear. Medical and veterinary literature began to appear. Antonio Benivieni (1440-1502),.an Italian, was a remarkable pioneer in reporting postmortem examinations. A gifted pathologist, he is credited as 'Father of Pathological Anatomy', and also as 'Founder of Pathology' before Morgagni. Leonardo da Vinci (1452-1519) was the greatest artist and scientist of the Italian Renaissance (golden period of the Italian civilization). Despite being an artist, he contributed to anatomy so much that he is honoured as 'First Modern Dissector'. He also wrote anatomy of the horse in 1499. Andreas Vesalius (1514- 1564), a Belgian, was the most outstanding figure in European medicine after Galen and before Harvey. At the young age of 24, he became professor of anatomy at the University of Padua, in Italy. There were plenty of dissectors and dissections before Vesalius, but he alone made anatomy what it is today - a living, working science. The 16th century is often called as the century of anatomy. Discovery of Blood Circulation Sixteenth and seventeenth centuries witnessed certain monumental discoveries in medicine. William Harvey (1578-1657), 10 History of Pathology an Englishman, described the blood vascular system and made the epoch-making discovery of blood circulation, in 1628. It is a gripping account of his genius. He dissected vascular system of no less than 80 different species of animals over a period of 14 years. His discovery had the greatest impact and changed the very course of medicine. It marked a new phase. Without the knowledge of blood circulation, most of the alterations in pathology could not be explained. Disturbances in circulation like hyperaemia, haemorrhage, embolism and many other alterations in pathology had remained unexplained unless circulation of the blood was understood. In fact, no single discovery has had a more far-reaching effect on pathology than the discovery of blood circulation. His discovery also clarified certain myths. For example, earlier, 'artery' was called as 'airtree'. Etymologically, the word was coined from the belief that arteries contained air and the veins blood, and the arteries also bifurcated like the branches of a tree. (Etymology is the study of the origin and history of words and their meanings). Discovery of circulation clarified the myth; the word 'airtree' became a misnomer (a wrong name), and was changed to 'artery'. Marcello Malpighi (1628-1694), an Italian, extended Harvey's work and discovered the capillaries and erythrocytes; and made masterly microscopic descriptions of the kidney, lungs, and spleen. He discovered Malpighian layer of the skin, and proved that the papillae of tongue are organs of taste. Inv_tlon and Application of Microscope An event of great significance was the invention of the microscope by Hans and Zacharias Jannsen in Holland (The Netherlands). Cornelius Drebbel, also of Holland, made a better microscope and the credit for the introduction of the microscope really goes to him. The next major advance in medicine was made by Antony van Leeuwenhoek (1632-1723), a Dutch draper (a cloth merchant), and yet a very great microscopist. He made 200 microscopes before he was satisfied and the best magnification he could obtain was 160. However, he is not given the credit for inventing the microscope because others had demonstrated magnification of lenses long before his time. All the same, he is credited for being the first to show that the microscope had practical importance in the study of tissues, and other minute objects. He was the first to see protozoa under the 11 Textbook of Veterinary General Pathology microscope, in 1675. Without the microscope, the study of histology would be impossible. By demonstrating the practical use of the microscope, Leeuwenhoek paved the way for the minute examination of tissues. With his simple crude instrument, he observed many objects. 'He even demonstrated the capillary anastomosis between the arteries and veins. First Textbook on Pathology One of the first compilers of medical literature was a Frenchman by the name Jean Fernel (1497-1558). He collected the information of his time, and was the first to codify (organize systematically) the new knowledge of pathology. He attempted to bring medical information into a form that could be used by others in the study of disease. He was the first to write and describe the diseases according to organs, or parts of the body. His writings formed the 'First Textbook in Pathology', which was the main text in teaching pathology for many years. Jean Femel (1497-1558) was followed by a famous Italian compiler - Giovanni Battista Morgagni (1682-1771). Modem pathology began with Morgagni. At the age of seventy, he published five volumes entitled 'The Seats and Causes of Disease' - an incredible feat of achievement. In these, he systematically described 700 complete autopsies, and attempted to correlate the structural changes to the symptoms shown by the individual. This was the first time that anyone had attempted to correlate pathological alterations in the dead with the symptoms shown during life. That is, he introduced the'anatomical concept' into the practice of medicine. His book was an extremely important text. Father of Histology A young Frenchman Marie-Fran~ois Xavier Bichat (1771-1802) opened a new field in medical science. During his brief life-span of 31 years (he died from tuberculosis), he established the foundation for the study of histology. He presented a new concept of anatomy and showed that body is composed of twenty-one tissues. The remarkable thing about Bichat's discovery was that his observations were made entirely by physical and chemical methods since he did not possess a microscope, and yet his contributions immortalized him as the 'Father of Histology'. It is a delightful story as to how he instilled the new concept. With simple methods such as maceration, chemical diSintegration using acids and bases, cooking, putrefaction and several 12 History of Pathology others, he was able to divide the tissues of the body into 21 groups. The word 'tissue' was first introduced by him. Etymologically, it is derived from the French word 'tissu', which means fabric or texture. In other words, Bichat meant that body is made up of fabrics of different textures! Father of Immunology Edward Jenner (1749-1823), an Englishman, discovered vaccination for smallpox, and performed his first vaccination on May 14, 1796 on a village boy, James Phipps. The immunization proved successful. The story of how he came to practise smallpox vaccination is fascinating. He observed that dairymaids (women working in a dairy), who had contracted cowpox through milking, did not suffer from smallpox. Jenner then thought of putting his observation to practice, and put forward the theory that vaccination against cowpox protects from smallpox. He thus became one of the greatest benefactors (one who does good to others) of mankind. He is honoured as the 'Father of Immunology'. Later, Jenner also contributed on a dog disease - canine distemper. Establlshmentof the First Veterinary College Modern veterinary medicine originated in France. In 1664, man by the name Jacques Labessie De Solleysel (1617-1680) published the first complete book on veterinary medicine. The publication of this work, with its influence on veterinary medicine, was the outstanding event of the seventeenth century in veterinary medicine. The second dominating veterinarian in France was Claude Bourgelat (1712-1779). Bourgelat was engaged in the practice of law, but was greatly interested in horses. In 1751, he wrote a book on equine medicine. He also investigated the glanders outbreak, and was successful in eradicating this disease for the French army. He was then requested to establish a veterinary college in Lyon, France, which he did. Thus, the first modern veterinary college was established in Lyon, France, on January 1, 1762. First Experimental Pathologist The next major contribution to medicine was made by a Scot - John Hunter (1728-1793). He is best known for his contributions on blood diseases and inflammation, gunshot wounds, and for a monograph 'venereal disease'. He recorded 700 autopsies. He was man of great curiosity. His great zeal for experimental pathology led 13 Textbook of Veterinary General Pathology him to infect himself with syphilis in order to study the disease more closely. He then developed typical case of the disease, which became the cause of his death. His saga of supreme sacrifice to enrich the medical science is an overpowering, spellbinding, heroic tale. He is credited as the 'First Experimental Pathologist'. Matthew Baillie (1761-1823), another Scot, wrote the first systematic text on pathological anatomy. In each case, the autopsy was correlated with a full case history. Supreme Descriptive Pathologist The Germans soon came to dominate the field of pathology. Carl Rokitansky (1804-1878), who lived in Vienna, is considered as the supreme descriptive pathologist of all time. The apogee (highest point) of gross descriptive morbid anatomy was reached by his work. He firmly established the structural basis of disease. He had conducted 70, 000 autopsies in his life. It was he who established postmortem techniques - the systematic examination of every organ by methods that preserved organ continuity yet re~ealed the lesions they contained. Cellular Pathology Johannes Mueller (1801-1878), a German, who lived in Berlin, was one of the first to use the microscope in the study of tissues. He impressed upon the world the necessity of examining tissues with the microscope in order to appreciate and understand the changes that were occurring Rudolph Virchow (1821-1902) was greatest of all German scientists. His foremost contribution was the publication of his book 'Cellular Pathology', in 1858, nearly one hundred and fifty years ago, in which he put forth the concept that all forms of injury start with molecular or structural alterations at the cellular level. All areas of pathology were clarified by his concept of cellular pathology. He gave pathology the push it needed, and from then on, its development was rapid. Thus, ,the rise of modern pathology and medicine is inseparably connected with his name. He is known as the 'Father of Cellular Pathology', or even as 'Father of Modern Pathology'. Most of the terms used in pathology, such as thrombosis, embolism, fatty degeneration, and amyloidosis, were coined by him. Serm Theory of Disease The importance of infectious organisms was demonstrated by 14 History of Pathology Louis Pasteur (1822-1895) in France. He was one of the originators of the new field of bacteriology and of the theory that bacteria were the cause of some diseases - known as the 'germ theory of disease'. His studies carried him into the field of human and animal diseases (pasteurellosis, anthrax, rabies). He showed that individuals could be successfully immunized by vaccine prepared with organisms and carried out immunization against anthrax. He established the science of bacteriology. Robert Koch (1843-1910), a German bacteriologist, isolated many microorganisms, showed that many bacteria caused diseases, and was the first to use artificial solid media to obtain pure cultures. In 1882, he discovered the tubercle bacillus. Further, he established 'Koch's postulates', a procedure necessary for proving a specific microorganism as the cause of a specific disease. That is, the organisms must be present in disease, must be obtained in pure culture and maintained by subculture, and on their inoculation the disease must be reproduced in healthy animals. Although Louis Pasteur and Robert Koch did much to show that bacteria caused disease, it was Edwin Klebs (1834-1913), who demonstrated the importance of bacteria in pathology. Originator of Modern Experimental Pathology Julius Cohnheim's (1839-1884) methods of investigation were experimental, and because of this he is credited as 'Originator of Modern Experimental Pathology'. He was a German and his contributions, particularly in the field of inflammation, are monumental. His most notable experiment concerned the vascular and cellular changes in the mesentery of the frog when acted upon by the irritant. He opened the abdomen of a frog and spread the mesentery across the stage of a microscope. He then placed a drop of dilute acetic acid on the mesentery and recorded the changes. His simple observations formed the basis for the pathology of inflammation. He was the first to provide microscopic observations of inflammation and wrote descriptions that can hardly be improved upon. Discovery of Phagocytosis The cellular changes in inflammation were clarified by the Russian biologist Elie Metchnikoff (1845-1916), in 1882. He inserted rose thorns into the larvae of a starfish. The next morning he observed a 15 Textbook of Veterinary General Pathology grey zone around each of the rose thorns. He found this grey zone to be an accumulation of large cells, and also observed ingestion of rose thorns by these cells. Because the cells were large and possessed phagocytic properties, he named them macrophages (G. macro = large + phagein = to eat), in contrast to the smaller phagocytic cell, the neutrophil, which is designated as the microphage (G. micro = small). He thus discovered the process of phagocytosis. In 1884, he described phagocytosis of bacteria by mammalian neutrophils. He concluded that the purpose of inflammation was to bring phagocytic cells to the injured area to engulf the invading bacteria. Discovery of Lysosomes(Sulclde Bags) Lysosomes are small vesicular bodies within a cell that contain a variety of very potent digestive enzymes. Because of the whole spectrum of acid hydrolases that they contain, lysosomes have come to dominate the events in pathology. Activation of these enzymes can lead to enzymatic digestion of every cell component (see 'Cell injury and cell death', Chapter 3). Because lysosomes, under certain circumstances, destroy the very cells that contain them, they are sometimes referred to as 'suicide bags'. The discovery of lysosomes is very interesting. Their existence was first postulated by Christian de Duve and his associates working at Louvain University, Belgium, in 1955. In fact, they were examining the enzyme content of mitochondrial fraction.. that could be separated from homogenates of rat liver cells by differential centrifugation. In one of the fractions, they unexpectedly found a number of digestive enzymes. After conducting many experiments, they postulated that the digestive enzymes must have been contained in some membrane- bound bodies. Realizing that the little bodies in this fraction were not mitochondria, but a new type of cytoplasmic organelle, they proposed the name lysosomes. They thought that these vesicular bodies contained enzymes that could digest cell structures (G. lysis = digestion, dissolution + soma = body), hence the name 'lysosomes'. A few years later, existence of these postulated lysosomes, was demonstrated by electron microscope by Novikoff and his associates; and this proved that the assumption of Christian de Duve and his team was correct. Thus, paradoxically, a structure that could be seen only with an electron microscope was discovered without its use. Ukewise, Bichat, through his ingenuity earned for himself the title 'Father of Histology', 16 History of Pathology without using a microscope. It is tempting to draw a similarity between the two discoveries, and marvel at the creative genius of their discoverers. Discovery of Prlon. 'Prions' are the most recent addition to the class of agents that cause diseases in domestic animals and humans. 'Prion' is a proteinaceous infective particle. Recent work has revealed that prion is a heavily glycosylated specific protein (a polypeptide) of 30 kilodaItons (30-kD), called 'prion protein (PrP). Since it is a proteinaceous particle closely associated with infectivity, the term 'proin' was coined from 'proteinaceous infective particle'. The word 'proin' thus formed from 'pro' and 'in' was changed to 'prion' to sound rhythmic. Although both 'prions' and 'viruses' replicate, their properties, structure, and modes of replication are fundamentally different. Prions lack nucleic acid (RNA or DNA), and also do not produce any inflammatory or immune reaction in the host. Thus, prions are the most unconventional agents. Because they lack nucleic acids, prions are remarkably resistant to many agents that normally inactivate viruses, such as ultraviolet light and standard disinfectants. In fact, they can be classified as an entirely separate category. For his discovery of 'prions', Stanley Prusiner, professor of Biochemistry at the University of California, San Francisco, USA, won the 1997 Nobel Prize in Physiology and Medicine. Both, in domestic animals and humans, prions cause a group of diseases known as 'transmissible spongiform encephalopathies (TSEs)' (see 'Prions', Chapter 15). To conclude, history of pathology has sequentially passed through the 'demoniac', 'theological or theistic' and 'humoral' phases, and is now in the 'cellular and molecular' phase. However, the rapid strides made recently in the field of molecular biology, and consequent thereupon in the field of molecular pathology, make one wonder if pathology is not again swinging somewhat in the direction of humoral pathology. More and more diseases are being explained by effects of the released chemical mediators as well as by antigen- antibody reactions and endocrine glands. Thus, diseases are manifestations of humoral products like various interleukins, tumour necrosis factor, several other cytokines, hormones, leukotrienes, prostaglandins, thromboxane A2, nitric oxide, and a host of others carried in the blood. 17 "This page is Intentionally Left Blank" Chapter 3 Cell Injury and Cell Death Introduction More than a hundred years ago, Rudolph Virchow, 'Father of Cellular Pathology', put forward the concept that disease begins at the cellular level. This has been abundantly established since then, by the phenomenal progress made in the field of molecular pathology. As all forms of tissue injury commence with molecular and structural changes in cells, we shall begin consideration of pathology with an examination of disease at the cellular and molecular levels. The normal cell1ives in a hostile environment In other words, it exists in a state of striking disequilibrium with its external environment. For example, the concentration of calcium ions outside the cell is 10,000 times higher than that inside. If all this calcium were to enter the cell, it will prove toxic, and kill the cell. This can happen during cell injury. It is the plasma membrane of the cell that constitutes the structural and functional barrier, which separates the intracellular from the hostile extracellular environment. It maintains a constant internal ionic composition, against very large chemical gradients between the intracellular and extracellular components. The membrane selectively admits small molecules, while throws out others. DefInitions The normal cell has to live within a fairly narrow range of function and structure. Even then, 1t is able to handle normal physiological demands, so-called normal homeostasis (L. staying same). Somewhat more excessive physiological stresses, or some pathological stimuli, bring about adaptation. That is, the cell modifies its structure and function in response to changing demands and stresses. It then acquires a new but steady state and preserves its health. For example, the bulging muscles of a race horse result from cellular adaptations. The increase in muscle mass is due to an increase in the size of the individual muscle fibres. The workload is thus shared by a greater mass of cellular components, and each muscle fibre is spared from excess work and therefore escapes injury. This adaptive response is called hypertrophy. Conversely, atrophy is a response in which there 19 Textbook of Veterinary General Pathology is a decrease in the size and function of cells. Hyperplasia and metaplasia are the other examples of adaptive responses. All these cellular adaptations are considered in detail in Chapter 7. If the adaptive capability is exceeded, or in certain cases when adaptation is not possible, a sequence of regressive changes occurs, collectively known as cell injury (L. re, retro = back; gress = step, i.e., step back, go back, fall in health; also called retrogressive or degenerative changes). In classical or traditional pathology, these changes were termed "degenerations", but now they are called cell injury. Within certain limits, injury is reversible and cells return to a normal state, but with severe and persistent stress, the cell reaches a 'point of no return', suffers irreversible injury and then dies. Adaptation, reversible injury, irreversible injury, and cell death are states of progressive encroachment on the cell's normal function and structure. Cell death, the ultimate result of cell injury, is one of the most important events in pathology, affects every type of cell, and is the main result of ischaemia (lack of blood flow), infections, toxins, and immune reactions. There are two main patterns of cell death: necrosis and apoptosis. Necrosis (typically coagulative necrosis) is the more common type of cell death, and occurs after a loss of blood supply or after an exposure to toxins. It is characterized by cellular swelling, denaturation and coagulation of cytoplasmic proteins, and breakdown of the organelles. Apoptosis occurs when a cell dies through activation of an internally controlled 'suicide' programme. After this, the dead cells are removed. Apoptosis is designed to eliminate unwanted cells during development of embryo and in various physiological processes. It also occurs under pathological conditions, where it is sometimes accompanied by necrosis. Cau... of Cell Injury 1. Hypoxia: Hypoxia (loss of oxygen supply) is an extremely important and common cause of cell injury and cell death. It affects cells aerobic oxidative respiration. Hypoxia should be differentiated from ischaemia, which is a loss of blood supply due to obstructed arterial flow or reduced venous drainage in a tissue. In contrast to hypoxia during which glycolytic energy production can continue, ischaemia affects the availability of metabolic substrates, including glucose. For this reason, ischaemia injures tissues faster than 20 Cell Injury and Cell Death hypoxia. Reduction in the oxygen-carrying capacity of the blood, as in anaemia, may also result in significant cell injury. 2. Physical agents: Physical agents include mechanical trauma, extremes of temperatures (burns and deep cold), radiation, electric shock, and sudden changes in atmospheric pressure. 3. Chemical agents and drugs: Virtually any chemical substance or drug can cause cell injury. Simple chemicals such as glucose or salt in hypertonic coricentrations may cause cell injury directly, or by deranging their osmotic environment. Even oxygen, in high concentrations, is severely toxic. Agents commonly known as poisons cause severe damage at the cellular level, and can result in the death of the whole organism. Many of these chemicals or drugs cause changes by acting on some vital functions of the cell, such as membrane permeability, osmotic homeostasis, or the integrity of an enzyme or cofactor. Other potentially toxic agents include air pollutants, herbicides, carbon monoxide, and asbestos. Even therapeutic drugs can cause cell or tissue injury. 4. Infectious agents: These agents range from the submicroscopic viruses to the large tapeworms. In between are the bacteria, fungi, rickettsiae, chlamydiae, mycoplasma, protozoa, and higher forms of parasites. The ways by which infectious agents cause injury are wide-ranging and are discussed in Chapter 15. 5. Immunological reactions: Although the immune system serves in the defence against infectious agents, immune reactions may also cause cell injury. Examples include anaphylactic reaction to a foreign protein or a drug, and autoimmune diseases. 6. Nutritional imbalances: Nutritional deficiencies such as avitaminoses and others are important causes of cell injury. Ironically, excesses of nutrition are also important causes of morbidity and mortality. In humans, diets rich in animal fat, cause atherosclerosis and obesity. 7. Genetic defects: Genetic defects may cause cell injury. The genetic injury may result in a defect as gross as congenital malformations, or in as subtle a change as the single amino acid substitution in haemoglobinS in sickle cell anaemia. The several inborn errors of metabolism due to congenital enzymatic deficiencies are examples of cell damage due to alterations at the level of DNA. 21 Textbook of Veterinary General Pathology General Conslderatlonsof Cell Injury The biochemical mechanisms responsible for cell injury and cell death are complex. Injury to cells has many causes. Also, there are a number of pathways to cell death that interact with one another. Thus, to find out exact cause and effect may be difficult. All the same, there are certain principles that are applicable to most forms of cell injury. 1. The morphological changes of cell injury become noticeable only after some critical biochemical system within the cell has been deranged. Thus, the first lesion to develop is biochemical (molecular) in nature. This, in turn, causes structural changes first at an electron microscopicallevel (ultrastructural lesion), then light microscopic lesions develop, and these, when extensive, produce gross lesions. 2. The cellular response to injurious stimuli depends on the type of injury, its duration, and its severity. For example, low doses of toxins or brief periods of ischaemia may cause reversible injury, whereas larger toxin doses or more prolonged ischaemia may result in irreversible injury and cell death. 3. The results of an injurious stimulus depend on the type, status, adaptability, and genetic make-up of the injured cell. The same injury has different result depending on the cell type. For example, striated skeletal muscle in the leg can tolerate complete ischaemia for 2-3 hours without irreversible injury, whereas cardiac muscle dies after only 20-30 minutes. Similarly, following loss of blood supply neurons die within 3-5 minutes; myocardium, hepatocytes and renal epithelium between 30 minutes to two hours; whereas fibroblasts, epidermis and skeletal muscles survive for many hours. The nutritional (or hormonal) status can also be important. For example, a liver cell full of glycogen will tolerate ischaemia much better than one that has just burned its last glucose molecule. Genetically determined variations in metabolic pathways can also be important. For example, exposure of two individuals to exactly the same concentrations of a toxin, such as carbon tetrachloride, may be without effect in one and may produce cell death in the other. 4. Although the exact biochemical site of action for many injurious agents is difficult to determine, four intracellular systems are particularly exposed to attack (1) Cell membrane. It is on the maintenance of the integrity of cell membrane that the ionic and osmotic homeostasis of the cell and its organelles depends, (2) 22 Cel/lnjury and Cel/ Death Anaerobic respiration involving mitochondrial oxidative phosphorylation and production of adenosine triphosphate (ATP), (3) Synthesis of enzymic and structural proteins, and (4) Preservation of the integrity of genetic apparatus of the cell. 5. The structural and biochemical components of a cell are so closely inter-related that, whatever the exact point of initial attack, injury at one site leads to wide-ranging secondary effects. For example, impairment of aerobic respiration disrupts the energy-dependent sodium pump in the membrane that maintains the ionic and fluid balance of the cell, resulting in the alterations in the intracellular content of ions and water. As a result, the cell can rapidly swell and rupture. Common Biochemical Mechanisms With certain injurious agents, the biochemical mechanisms are well defined. For example, cyanide inactivates cytochrome oxidase in mitochondria and certain bacteria can producephospholipases that attack phospholipids in cell membranes. However, with many injurious stimuli the exact pathogenic mechanisms that lead to cell death are incompletely understood. In spite of this, there are several common biochemical pathways in the mediation of cell injury and cell death, whatever the causative agent. These include: 1. ATP depletion: High-energy phosphate in the form of ATP is required for many processes within the cell. ATP is produced in two ways: (1) The major route is oxidative phosphorylation of ADP, a reaction that requires oxygen, and (2) the second is the glycolytic pathway that can generate ATP in the absence of oxygen using glucose obtained either from body fluids, or from hydrolysis of glycogen. ATP depletion and decreased ATP synthesis are common consequences of both ischaemic and toxic injury. 2. Lack of oxygen or generation of oxygen-derived free radicals: A lack of oxygen certainly underlies the pathogenesis of cell injury in ischaemia, but partially reduced activated oxygen species are also important mediators of cell death (Fig. 1). Cells generate energy by reducing molecular oxygen (02) to water. During this process, small amounts of partially reduced reactive oxygen forms are produced as an unavoidab1e by-product of mitochondrial respiration. They are highly toxic molecules that can damage lipids, proteins, and nucleic acids (discussed later). These molecules are referred to as activated or reactive oxygen species. Cells have defence 23 Textbook of Veterinary General Pathology systems to prevent injuries caused by these products. An imbalance between free radical-generating and free radical-inactivating systems results in oxidative stress, a condition associated with cell injury. Radiation 1 Inflammation Oxygen toxicHy ~ ~Chem°2... Isch,ia 1 Activated oxygen species ~ (°2'": H20 2 , OH·) J,°2 ! ~CellinjUry Fig.1. The role of oxygen in cell injury. Ischaemia causes cell injury by reducing its oxygen supply. Other stimuli, such as radiation, induce damage through toxic activated oxygen species (i.e., free radicals). 3. Loss of calcium homeostasis (stable state): Calcium (Ca++) in cytosol is normally maintained at extremely low concentrations. (Cytosol is fluid portion of the cytoplasm). Concentration of calcium in cytosol is up to 10,000 times lower than the concentration of extracellular calcium, or of calcium sequestered (isolated) within mitochondria and endoplasmic reticulum. Normally, most of the intracellular calcium is sequestered in mitochondria and endoplasmic reticulum. Such gradients are maintained by membrane-associated, energy-dependent Ca++, Mg++ -ATPases. Ischaemia and toxins increase cytosolic calcium concentration due to a net influx (entry) of extracellular calcium (Ca++) through the plasma membrane, and also because of the release of Ca++ from mitochondria and endoplasmic reticulum (Fig. 2). Increased cytosolic calcium, in turn, activates a number of enzymes, with harmful cellular effects (Fig. 2). The enzymes activated by calcium include: (1) phospholipases, which cause membrane damage, (2) proteases, which break down structural and membrane proteins, (3) ATPases, which accelerate ATP depletion, and (4) endonucleases, which break down nuclear chromatin. Although cell injury results in increased intracellular calcium and this in turn causes a variety of harmful effects, including cell death, loss of calcium homeostasis is not always a necessary preceding event in irreversible cell injury. 24 Cel/lnjury and Cel/ Death Injurious agent ! - - - - - - - - Cell membrane 1 ~:~~[~... re",".m Increased cytosolic Ca" ! ! ATPase 1 Phospholipase 1 Protease Endo- nuclease ! Decreased ! Decreased ! Disruption of ! Nuclear ATP phospholipids membrane and chromatin cytoskeletal damage proteins Fig. 2. Consequences of increased intracellular calcium in cell injury. 4. Defects in membrane permeability: The plasma membrane may be directly damaged by certain bacterial toxins, viral proteins, complement components, cytotoxic lymphocytes, and a number of physical or chemical agents. Changes in membrane permeability may also be secondary to a loss of ATP synthesis, or may result from calcium-mediated activation of phospholipases. 5. Mitochondrial damage: Since all cells depend on oxidative metabolism, mitochondrial integrity is mostimportantfor cell survival. Irreparable damage to mitochondria wiII ultimately kill ceIIs. Mitochondria are almost always involved in most types of injury. They can be damaged by a variety of stimuli, such as increases of cytosolic calcium, by oxidative stress, and by breakdown of phospholipids through phospholipase A2 (discussed later). The damage ultimately results in the formation of high-conductance channels in the inner mitochondrial membrane. These channels are also called as 'mitochondrial permeability transitions' (Fig. 3). Due to these pores, the proton gradient across the mitochondrial membrane disappears, thereby preventing ATP generation. Mitochondrial damage also results in the leakage of cytochrome c (an important soluble protein in the electron transport chain) into the cytosol (Fig. 3), where it activates apoptotic death pathways (i.e. cell death). (see Apoptosis' and Figs. 11 and 12). I 25 Textbook of Veterinary General Pathology Mitochondrial injury or dysfunction (Increased cytosolic Ca++, oxidative stress, hpld peroxidation) Cytochrome c I 'J e membrane Cytochrome c I Mitochondrial permeability transition (MPT) Secondary apoptotlc effects Fig. 3. Mitochondrial permeability transition. Reversible and Irrever.,le Cell Injury To trace the sequence of events in reversible and irreversible cell injury, we shall discuss two model systems (1) ischaemic and hypoxic injury, and (2) free radical-induced cell injury. Ischaemic and Hypoxic Injury This is the most common type of cell injury and has been studied extensively in humans, in experimental animals, and in culture systems (Fig.4). Hypoxia (loss of oxygen supply) must be differentiated from ischaemia which is a loss of blood supply. In contrast to hypoxia, during which glycolytic energy production can continue (although less efficiently than by oxidative pathways), iscahemia affects the delivery of substrates for glycolysis (e.g., glucose) supplied by the flowing blood. Therefore, in ischaemic tissues, anaerobic energy generation will stop after glycolytic substrates are exhausted. For this reason, ischaemia injures tissues faster than hypoxia. Rever.lbleCelllnJury The first point of attack of hypoxia is the cell's aerobic respiration, that is, oxidative phosphorylation by mitochondria (Fig. 4). As the oxygen tension within the cell decreases, there is loss of oxidative 26 Cell Injury and Cell Death Ischaemial Hypoxia --+ Direct membrane Reversible ! Decreased oxidative damage (Membrane injury) injury phosphorylation See Fig 5 for mechanisms of ! Decreased ATP cell death throygh this pathway 1 ! , Decreased Na pump activity ! Increased Ca- Influx Increased HP & Na' influx Incre1ed K' efflux Cellular swelling Loss of microvilli dlebs ER swelling Myelin figures Release & activation of Irreversible lysosomal enzymes Injury l Increased basophilia Decreased ribonucleoprotein Nuclear changes Protein digestion l Cell death (necrosis) Fig. 4. Sequence of events in ischaemic injury. phosphorylation and decreased generation of adenosine triphosphate (ATP). The depletion of ATP (the energy source) has widespread effects on many systems within the cell. As is known, a normal cell has a higher intracellular osmotic pressure exerted by a greater intracellular than extracellular concentration of proteins. To balance this, sodium is maintained at a higher concentration outside the cell than inside by an energy-dependent (ATP-driven) "sodium pump", that is, by Na+ and K+-adenosine triphosphatase (ATPase) enzyme. This pump also keeps concentration of potassium significantly higher inside the cell than outside. The decreased ATP concentration, following acute hypoxia, reduces activity of the plasma membrane "sodium pump", which then fails to regulate the active transport of 27 Textbook of Veterinary General Pathology ions. This results in the accumulation of sodium inside the cell, and diffusion of potassium out of the cell. The net gain of sodium increases the osmotic pressure inside the cell. The extracellular osmotic pressure now being low, water enters into the cell, producing acute cellular swelling. This is worsened by the increased osmotic load from the accumulation of other metabolites, such as inorganic phosphates, lactic acid, and purine nucleotides. Cellular swelling is the first manifestation of almost all forms of injury to cells. The entry of water into the cell is associated with early dilation of the endoplasmic reticulum. The decrease in cellular ATP and associated increase in adenosine monophosphate (AMP) stimulate the enzyme phosphofructokinase and phosphorylase. This results in an increased rate of anaerobic glycolysis to maintain the cell's energy supply by generating ATP from glycogen. Glycogen is thus rapidly depleted (Fig. 4). This can be noticed microscopically if tissues are stained for glycogen with periodic acid-Schiff (PAS) stain. Gycolysis results in the accumulation of lactic acid and inorganic phosphates from the hydrolysis of phosphate esters. This reduces the intracellular pH. This reduced pH causes early clumping of nuclear chromatin (Fig. 4). Decreased pH and ATP levels cause detachment of ribosomes from the granular endoplasmic reticulum (Fig. 4) and dissociation of polysomes into monosomes, with a resultant reduction in protein synthesis. If hypoxia continues, diminishing mitochondrial function and increasing membrane permeability, cause further morphological changes. Ultrastructurally, blebs may form at the cell surface. 'Myelin figures', that is, concentric laminations derived from plasma as well as organellar membranes, may be seen within the cytoplasm or extracellularly. At this time, mitochondria, endoplasmic reticulum, in fact the whole cell usually appears swollen due to a loss of osmotic regulation. However, all these changes are reversible if oxygen is restored. But if ischaemia persists, irreversible injury follows. Irreversible eelllnJury Irreversible injury is associated morphologically with severe swelling of mitochondria, extensive damage to plasma membranes, and swelling of lysosomes. Extracellular calcium enters into the cell. Large, amorphous, calcium-rich densities accumulate in the mitochondrial matrix. After this, there is continued loss of proteins, essential coenzymes, and ribonucleic acids from the hyperpermeable plasma membrane. The cells may also leak metabolites, which are 28 Cell Injury and Cell Death vital for the reconstitution of ATP, thus further depleting net intracellular high-energy phosphates. The falling pH (due to accumulation of lactic acid and inorganic phosphates) causes injury to the lysosomal membranes. This is followed by leakage of their lysosomal enzymes into the cytoplasm and activation of acid hydrolases. Lysosomes contain RNases, DNases, proteases, phosphatases, glucosidases, and cathepsins. Activation of these enzymes leads to enzymatic digestion of cytoplasmic and nuclear components (Fig. 4). After death, cellular components are progressively digested by lysosomal hydrolases, and there is a leakage of potentially destructive cellular enzymes into the extracellular space, and entry of extracellular macromolecules into the dying cells. Finally, the dead cell may become replaced by large masses composed of phospholipids called myelin figures. These are then either phagocytosed by other cells, or degraded further into fatty acids. Calcification of such fatty acid residues may occur with the formation of calcium soaps. Mechanisms of Irreversible Injury The biochemical changes described so far are a continuous sequence of events from the onset of cell injury to ultimate digestion of the irreversibly damaged cell by lysosomal enzymes. But at what stage did the cell actually die? And what is the critical biochemical event responsible for the 'point of no return'? Two phenomena consistently characterize irreversibility. The first is the inability to reverse mitochondrial dysfunction (lack of oxidative phosphorylation and ATP generation) even after correction of the original injury (e.g., restoration of blood flow), and the second is the development of profound disturbances in membrane function. Cell Membrane Dama.e It is now clear that cell membrane damage is a central factor in the pathogenesis of irreversible cell injury. The cell membrane consists of a lipid-protein mosaic made up of bimolecular layer of phospholipids and globular proteins embedded within the lipid layer. An intact plasma membrane is essential for the maintenance of normal cell permeability and volume. There are several biochemical mechanisms that cause membrane damage (Fig. 5). 29 Textbook of Veterinary General Pathology Ischaemia ~ Oxygen deficiency ! r Decreased ATP 1 Increased cytosolic Ca++ ! Decreased phospholipid ~ I reacylation/synthesis Phospholipase Protease Leukocyte '·"'"i activation activation e ! ,.,. ,'". j Increased phospholipid 1., r Cytoskeletal damage PhDSPI'PId 10.. Lipid breakdown Lipid products peroxidatlon ~ ~ Membrane Membrane Membrane Membrane damage damage damaae damage Fig. 5. Mechanisms of membrane damage in ischaemia. 1. Progressive loss of membrane phospholipids: Normally, the turnover of membrane phospholipids is associated with their re- synthesis so that the integrity of cell membrane is maintained. The normal intracellular calcium concentration is 10,000 times (10-iM) lower than the concentration of calcium ions (Ca++) in extracellular fluids (10-3M), or of sequestered (isolated) mitochondrial and endoplasmic reticulum calcium. Moreover, the intracellular calcium is sequestered in mitochondria and endoplasmic reticulum. Oxygen deprivation (hypoxia) releases sequestered calcium from mitochondria and endoplasmic reticulum, thus raising cytosolic calcium (Fig. 5). The increased cytosolic calcium concentration induced by ischaemia, following acute hypoxic injury, activates endogenous phospholipases, whose activation is strongly calcium dependent. The phospholipases, in turn, cause extensive cell membrane damage. They do so by causing increased phospholipid degradation, and thus, phospholipid loss. Progressive phospholipid loss can also occur from decreased de novo 30 Cel/lnjury and Cell Death (fresh) synthesis of phospholipids, or decreased ATP-dependent reacylation (acylation is introduction of an acyl group). Whatever, the cause, following phospholipid degradation, lipid breakdown products (un-esterified free fatty acids, acyl carnitine, and lysophospholipids) accumulate within ischaemic cells, and cause further cell membrane damage and changes in permeability. 2. Mitochondrial dysfunction: Whatever the mechanism of cell injury, loss of membrane integrity causes further influx of calcium from the extracellular space, where it is present in high concentration, into the cells. Calcium is taken up greedily by mitochondria. Here it activates mitochondrial phospholipases and results in accumulation of free fatty acids. Phospholipases and free fatty acids together cause changes in the permeability of the inner mitochondrial membrane, such as mitochondrial permeability transition, as well as of the outer mitochondrial membrane (see Fig. 3). 3. Cytoskeletal abnormalities: Cytoskeletal filaments bind firmly plasma membrane to interior of the cell. Activation of proteases by increased intracellular calcium may cause damage to cytoskeleton (Fig. 5). In the presence of cell swelling, this damage results in detachment of the cell membrane from the cytoskeleton, exposing it to stretching and rupture. 4. Toxic oxygen radicals: As will be discussed in detail later, partially reduced oxygen free radicals are highly toxic molecules that cause injury to cell membranes and other cellular constituents (Fig. 5). Such oxygen radicals are increased in ischaemic tissues. Whatever the mechanism of membrane damage, loss of membrane integrity causes massive influx (entry) of calcium into the cell from the extracellular space, where it is present in high concentration. Calcium is then readily taken up by mitochondria and permanently poisons them, inhibits cellular enzymes, denatures proteins, and causes the cytoplasmic changes characteristic of coagulative necrosis. To summarize, hypoxia affects oxidative phosphorylation and hence the supply of vital ATP; membrane damage is a critical step in the development of lethal cell injury; and calcium is an important mediator of the biochemical and morphological alterations in cell death. 31 Textbook of Veterinary General Pathology Free Radical-Induced Cell Injury As mentioned in the discussion of I cell membrane damage', injury induced by free radicals, particularly that induced by activated oxygen species, is an important mechanism of cell 4amage. It occurs in such widely different processes as chemical and radiation injury, oxygen and other gaseous toxicity, bacterial killing by phagocytic cells, inflammatory cell damage, cellular ageing, tumour destruction by macrophages, and other injurious processes. It is therefore extremely important that we understand this topic, as to what are free radicals. How do they mediate the cell injury, and once formed, how does the body get rid of them? For an easy understanding of this rather complicated topic, it would be helpfulif we first briefly review certain basic facts of physical chemistry. All matter in the universe is composed of the basic unit known as an element. There are 103 known elements. Elements are fundamental substances, that consist of atoms of only one kind, e.g., hydrogen, nitrogen, calcium, phosphorus, iron, etc. Although 103 elements are known, only a few play an important role in living organisms. In fact, just four elements, hydrogen, oxygen, carbon and nitrogen account for 96 percent of the body weight, and over 99 percent of the atoms in the body. Atoms are composed of three fundamental particles: protons, neutrons, and electrons. An atom has a central nucleus (containing protons and neutrons), and surrounding electrons. The proton has a positive charge, electron a negative, and neutron is electrically neutral. The electrons revolve (rotate) around the nucleus much as the planets revolve around the sun. The number of electrons, characteristic of an atom, is equal to the number of protons present in the nucleus. The atomic number of an element indicates the number of protons within the nucleus. For example, the atomic number of hydrogen is 1, therefore it has only a single proton in its nucleus; that of carbon is 4, so four protons, and thatof oxygen is 8, it has 8 protons and so on. Within an atom, the electrons are arranged within a series of shells or orbitals (orbits). Electrons in the orbitals are arranged in pairs and spin in opposite directions, thus cancelling each others physicochemical reactivity. The inner (first) orbital fills first, and for an atom to be stable, it must have two electrons (i.e., paired and the maximum number it can hold), otherwise it will be unstable and highly reactive. For example, an atom of hydrogen has only one electron in 32 Cel/lnjury and Cel/ Death its orbital and is therefore unstable and highly reactive. It therefore reacts with another atom of hydrogen and, in combining, the two atoms share electrons so that the orbital has now two electrons, and form a molecule of hydrogen. Each pair of shared electrons is called a covalent bond, which 'holds' two adjacent atoms together. Thus, forming a covalent bond involves a sharing or an exchange of electrons between two atoms. The resultant stable group of two or more atoms, linked together by covalent bonds, is called a molecule. When atoms combine to form molecules, electrons in only the outer orbital are involved. As per the electronic theory of valency, for an atom to be stable, the outer orbital must have a group of 8 electrons (octet). That is, the most stable electron configuration is one in which the outer orbital contains maximum number of electrons, otherwise the atom will be unstable and will react until octet is formed. For example, an atom of oxygen (atomic number 8) has only 6 electrons in the outer orbital (2 being in the inner orbital). It therefore combines with another atom of oxygen, shares electrons through covalent bonds, fulfils its requirement of eight, and forms a stable molecule; or it may react with two atoms of hydrogen, achieve its electronic configuration of octet, and thus form a stable molecule of water. After this brief consideration of an atom and its electron configuration, it is now easy to understand what a free radical is. A free radical is an atom, or a group of atoms, that has one or more unpaired electrons in the outer orbital. In other words, free radicals are chemical species that have a single unpaired electron in the outer orbital. In such a state the free radical is extremely reactive and unstable, and enters into reactions in cells with inorganic or organic substances (proteins, lipids, carbohydrates), particularly with the key molecules in membranes and nucleic acids. A second feature of free radicals is that they initiate autocatalytic reactions. This means that the molecules that react with free radicals are themselves converted into free radicals, which in turn propagate the chain damage. An unpaired electron can be associated with almost any atom, butthose derived from oxygen arethe most important in cell injury. However, nitric oxide (NO), generated by many types of cells can act as a free radical, or can be converted into highly reactive nitrite species. Oxygen-derived free radicals are produced during normal metabolic processes in reduction-oxidation (redox) reactions. For 33 Textbook of Veterinary General Pathology example, during normal respiration molecular oxygen (02) is sequentially reduced in mitochondria by the addition of four electrons to generate water. In this process small amounts of toxic metabolites are produced. These include superoxide radicals (Ot) , hydrogen peroxide (H20 2), and hydroxyl radicals (OH·). These can be produced by the activity of a variety of oxidative enzymes in different sites of the cell, that is, endoplasmic reticulum (P-450, b5 oxidases), mitochondria (P-450, b5 oxidases), plasma membrane (NADPH oxidase), cytosol (xanthine oxidase, transition metals (Cu, Fe), peroxisomes (multiple oxidases), and lysosomes (in phagocytes - myeloperoxidase, NO synthase). 1. Superoxide (0;'"): Superoxide is a molecule of oxygen that has 13 electrons in its outer orbital, instead of normal 12. Because of the presence of an unpaired single electron in the outer orbital, it is extremely reactive. Since it carries net negative charge (anion), it is a negative radical (oxide), and because it is extremely reactive (super), it is called a 'superoxide anion', termed simply as superoxide. It is generated either directly during auto-oxidation, or by the transfer of a single electron to 02 in reactions catalyzed by cytoplasmic enzymes, such as cytochrome P450, xanthine oxidase, and the respiratory burst oxidase (NADPH oxidase) present in neutrophils. O2 + e- NADPH oxidase ~ O;r Rapid bursts of superoxide production occur in activated neutrophils during inflammation. Once produced (OiT) can be inactivated either spontaneously or more rapidly by the enzyme superoxide dismutase (SOD), forming H 20r Superoxide dismutases are found in many types of cells. The group includes both manganese-superoxide dismutase, which is present in mitochondria and copper-zinc-superoxide dismutase, which is found in the cytosol. 2. Hydrogen peroxide (H20 2): ~02 is produced either by the dismutation of IS up eroxide' as just mentioned, or directly by oxidases present in peroxisomes. Peroxisome is a cytoplasmic organelle containing enzymes, both for the production and degradation, of hydrogen peroxide. Catalase, present in peroxisomes, decomposes hydrogen 34 Cel/lnjury and Cel/ Death peroxide into oxygen and water. 2H2 0 2 Being a free radical, hydrogen peroxide is an unstable compound. Glutathione peroxidase (GSH-Px), an enzyme present in cytosol also protects against injury by catalyzing free radical breakdown. H2 0 2 + 2GSH CSSG + 2~0 or 20H- [reduced] [oxidized] or 20H-+2GSH - - - - -... 2H2 0 + GSSG The ratio of oxidized glutathione (GSSG) to reduced glutathione (GSH) within the cell indicates the oxidative state of the cell. It is an important aspect of the cell's ability to inactivate reactive oxygen species. 3. Hydroxyl radicals (OH·): These are generated by: (a) Hydroxyl radicals are produced by interaction with transition metals (e.g., iron, copper) in the Fenton reaction. In this reaction, iron and copper donate or accept free electrons during certain intracellular reactions and thereby catalyze free radical formation (Fenton reaction). Fe++ + H2 0 2 Fe+++ + OH-+ OH- [ferrous] [ferric] Since most of the intracellular free iron is in the ferric (Fe+++) state, it must first be reduced to the ferrous (Fe++) form to participate in the Fenton reaction. This reduction step is catalyzed by superoxide. Thus, iron and superoxide synergize to produce maximal oxidative cell damage. (b) Hydrolysis of water by ionizing radiation. This is due to the absorption of radiant energy (e.g., ultraviolet light, X-rays). Ionizing radiation can hydrolyze water into hydroxyl (OH·) and hydrogen (H·) free radicals. H 2 0 - - - -... OH"+ H" Hydroxyl radical has only seven electrons in the outer orbital, thus one is left unpaired. Hydroxyl radicals are the most reactive in inducing cell damage. 4. Singlet oxygen: Singlet oxygen is a form of oxygen in which one electron is shifted into a high energy orbit. Because of its distorted 35 Textbook of Veterinary General Pathology electron configuration, singlet oxygen is very unstable and reactive. 5. Nitric oxide (NO): NO is a soluble, free radical gas produced by endothelial cells, macrophages, neurons, and other cell types. It is an important chemical mediator (see Chapter 4). Apart from itself acting as a free radical, it can also be converted to highly reactive peroxynitrite anion (ONOo-) as well as N02' (nitrogen dioxide) and N03-. Mechanlsmsof Cell Injury The effects of free radicals are wide-ranging. However, the following three reactions are most important in cell injury. 1. Lipid peroxidation of membranes: Free radicals in the presence of oxygen may cause peroxidation of Iipids in plasma and organellar membranes. Oxidative damage begins when double bonds in unsaturated fatty acids of membrane lipids are attacked by oxygen- derived free radicals, particularly byhydroxyl radicals (OH'). Double bonds are vulnerable to attack by free radicals. The lipid-radical interactions yield peroxides, which are themselves unstable and reactive. Therefore, an autocatalytic chain reaction follows (called _ propagation), which can result in extensive membrane, organellar, and cellular damage. Termination takes place when the free radical is captured by a scavenger, such as vitamin E, embedded in the cell membrane. Also, vitamin A, C and beta-carotene either block the formation of free radicals or inactivate them once they are formed. 2. Cross-linking of proteins: Free radicals act on the sulphydryl bonds (-SH-HS-) of proteins. Cross-linking of proteins by the for~ation of disulphide bonds (-5-5-) in such labile amino acids as methionine, histidine, cystine, and lysine causes extensive damage throughout the cell. In particular, the free radicals inactivate the sulphydryl enzymes. 3. DNA fragmentation: Free radical reactions with thymine in nuclear and mitochondrial DNA produce single-stranded breaks. This induces mutations in the genetic code. Such DNA damage has been implicated in both cell killing and malignant transformation of cells. To conclude, the final effects induced by free radicals depend on the net balance between free radical formation and termination. Cellular Swelling Cellular swelling (previously known as cloudy swelling, parenchymatous, or albuminous degeneration) is a disturbance of 36. Cel/lnjury and Cel/ Death cellular metabolism in which cells swell and cytoplasm of the cell becomes more granular than normal. Cellular swelling is the most common disturbance of cell metabolism and is the first readion of a cell to injury. It is caused by the mildest irritants and results from the shift of extracellular water into the cell, caused by mechanisms described earlier (see 'reversible injury'). The term cloudy swelling earlier referred to gross appearance of the affected organ, but is now outdated and no longer in use. Aetiology: Since cellular swelling is caused by the mildest irritants, it can be produced by any factor that interferes with cellular metabolism. The causes include: (1) bacterial toxins (the most common cause). Cellular swelling occurs in infectious diseases. (2) a rise in body temperature (fever), (3) metabolic diseases (diabetes and acetonaemia), (4) organic or inorganic poisons (lead, arsenic, chloroform, and alcohol), and circulatory disturbances (anaemia, infarction, passive hyperaemia, and haemorrhage) when insufficient oxygen is brought to the cell. Macroscopically, the affected organ (liver, kidney) is slightly enlarged, the edges are slightly rounded, and there is an increase in weight. The changes are due to an increased amount of fluid in the affected cells. The organ appears pale or anaemic, because the swollen cells compress the capillaries reducing the amount of blood in the organ. When incised, the cut surface bulges and its capsule draws back slightly. The cut surface is cloudy, slightly opaque, and appears as if it had been slightly scalded or cooked. Microscopically, cellular swelling is best observed in the liver, the convoluted tubules of the kidney, or in skeletal and cardiac muscle. The mechanism of cellular swelling has been discussed under reversible injury. Due to accumulation of fluid within the cells, the cells become swollen, and their edges become rounded. The cytoplasm stains slightly more intense with eosin. The internal structures of the cell are slightly hazy. The cytoplasm of the cell is more granular than normal. These granules are soluble in acetic acid, but not in lipid solvents such as chlorof9rm. Significance and result: Cellular swelling is a reversible injury and indicates the cell has been exposed to a mild irritant, or that hypoxia has been present. As soon as the cause is removed, the granules disappear, the fluid leaves the cell, and the cell returns to normal. Subcellular Responsesto Injury So far the changes described refer to either whole tissue, or the 37 Textbook of Veterinary General Pathology cell as a unit. However, certain conditions are associated with changes involving only subcellular organelles and cytoplasmic proteins. These indude: Lysosomal catabolism Primary lysosomes are membrane-bound intracellular organelles containing a variety of hydrolytic enzymes. These fuse to form secondary lysosomes or phagolysosomes that contain material meant for digestion. Lysosomes break down the ingested materials in two ways: heterophagy and autophagy. Heterophagy: In this, materials from external environment are taken up through the process of endocytosis. Uptake of particulate matter is called phagocytosis (G. phagein=to eat), and that of soluble macromolecules as pinocytosis (G. pinein=to drink). Endocytosed vacuoles and their contents ultimately fuse with a lysosome. This results in degradation of the engulfed material. Examples: bacteria are ingested and degraded by neutrophils, and macrophages engulf and degrade necrotic cells. Autophagy: In this process, intracellular organelles are isolated from the cytoplasm in an autophagic vacuole formed from rough endoplasmic reticulum (RER). The autophagic vacuole then fuses with primary lysosomes to form an autophagolysosome. Autophagy is a common phenomenon and is involved in the removal of damaged or senescent (old) organelles and in the cellular remodelling associated with cellular differentiation Itis prominent in cells undergoing atrophy induced by nutrient or hormonal deprivation. The enzymes in lysosomes can completely break down most proteins and carbohydrates, but some lipids remain undigested. Lysosomes with undigested debris may persist within cells as residual bodies, or may be thrown out. Lipofuscin pigment granules, discussed later, represent indigestible material that results from intracellular lipid peroxidation. Certain indigestible pigments, such as carbon particles, can persjst in phagolysosomes of macrophages for decades. Lysosomes are also places where cells isolate materials that cannot be completely metabolized. Hereditary lysosomal storage disorders caused by deficiencies of enzymes that break down various macromolecules, result in abnormal collections of intermediate metabolites in the lysosomes of cells all over the body, particularly neurons, leading to severe abnormalities. 38 Cel/lnjury and Cel/ Death Hypertrophy of Smooth Endoplasmic Reticulum Prolonged use of barbiturates leads to increased tolerance. The patients are then said to have 'adapted' to the medication. This adaptation is due to hypertrophy (increased volume) of liver cell smooth endoplasmic reticulum (SER). Barbiturates are metabolized in the liver through the P- 450