Moon Exploration Document PDF
Document Details
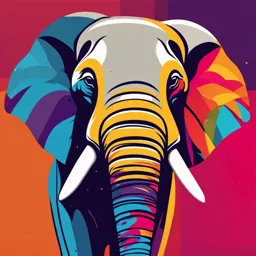
Uploaded by AstoundedFactorial
Tags
Summary
This document outlines the exploration of the moon, discussing its general properties and the various missions involved. It explores the composition and structure of the moon, highlighting the recent discovery of water ice near the lunar poles.
Full Transcript
Introduction ------------ The Moon is the sole celestial body that humans have ever visited. What does it feel like to stand on the surface of our natural satellite? And what knowledge can we gain from exploring there and bringing back fragments of a different world? We commence our examination of...
Introduction ------------ The Moon is the sole celestial body that humans have ever visited. What does it feel like to stand on the surface of our natural satellite? And what knowledge can we gain from exploring there and bringing back fragments of a different world? We commence our examination of the planets as pockmarked entities with two relatively uncomplicated celestial bodies: the Moon and Mercury. Unlike Earth, the Moon is devoid of geological activity, being a place that has utilized all its internal energy sources. As its atmosphere-less surface preserves ancient events, the Moon offers a glimpse into earlier stages of solar system history. The planet Mercury bears many similarities to the Moon, which is why they are discussed in tandem: both are comparatively small, lack atmospheres, have limited geological activity, and are primarily influenced by impact cratering. Nevertheless, the processes that have shaped their surfaces are not exclusive to these two worlds. It will become apparent that they have also affected numerous other members of the planetary system. General Properties of the Moon ------------------------------ The Moon\'s mass is only 1/80th that of Earth and it has approximately 1/6th of Earth\'s surface gravity, which is insufficient to keep an atmosphere (See Figure 5.2). Gas molecules in motion can escape from a planet in a similar manner to a rocket, and the lower the gravity, the easier it is for the gas to seep into space. Although the Moon can briefly acquire an atmosphere from comet impacts, this atmosphere is rapidly lost through freezing onto the surface or escaping into surrounding space. Presently, the Moon is notably lacking in a wide variety of volatile substances, which are elements and compounds that evaporate at relatively low temperatures. Table 5.1 provides a summary of some of the Moon\'s characteristics, with corresponding values for Mercury included for comparison. ### Exploration of the Moon Much of our knowledge about the Moon comes from the US Apollo program, which landed 12 astronauts on its surface between 1968 and 1972. Before that, astronomers had mapped the side facing Earth with limited detail. The era of spacecraft exploration began in the early 1960s, with Russia initially taking the lead with Luna missions. However, the Apollo missions, summarized in Table 5. 2, outshone these efforts. Initially focused on flat plains for safety, later missions targeted more interesting geological sites. Each mission increased scientific exploration with longer stays and better equipment. The last Apollo mission even included a geologist, Jack Schmitt, among the astronauts. Overall, the Apollo program significantly expanded our understanding of the Moon and its geology, building on previous studies and laying the foundation for future exploration. In the aftermath, the giant Apollo rockets were left to decay on NASA center lawns, though some have since been relocated to museums. More than 50 years later, there is renewed interest in human lunar flights, with NASA\'s Artemis program planning to return astronauts to lunar orbit by the mid-2020s, focusing on landings near the poles and including diverse astronaut crews. China has also expressed interest in sending humans to the Moon, having completed their own space station in Earth orbit as a preliminary step. While some skeptics question the Moon landings, suggesting they were faked, the scientific community continues to study the Moon with enthusiasm. Several scientific spacecraft from various countries have orbited or landed on the Moon in recent years, highlighting the ongoing interest in lunar exploration and research. Lunar exploration is a global effort, with various countries sending robotic spacecraft to study the moon. In the 1960s, the USSR launched robot sample returns, and China has recently been active with three landers and a sample return mission. The focus now is on finding accessible water ice near the lunar South Pole. Some of the latest lunar missions are listed in Table 5. 3. ### Composition and Structure of the Moon The Moon\'s composition differs from Earth\'s, with a lower average density of 3. 3 g/cm3 indicating it is mostly made of silicate rock and depleted in iron and metals. The absence of a large metal core has been confirmed through seismic studies using Apollo program seismometers and more precise inward tracking by GRAIL spacecraft. Lunar samples show depleted water and volatiles in the crust, with chemically bound water found in the rocks. Water ice has been discovered in shadowed lunar craters near the poles, confirmed by NASA\'s LCROSS mission in 2009 and measurement by the Lunar Reconnaissance Orbiter (LRO). The estimated quantity of water ice in the polar craters is in the hundreds of billions of tons, potentially crucial for future human habitation or a lunar base for travel to Mars and beyond. If mined, the ice could provide water and oxygen for human use, as well as hydrogen and oxygen for rocket fuel. This discovery sheds light on the possibility of utilizing lunar resources for human exploration and advancement in space. The Lunar Surface ----------------- ### General Appearance When looking at the Moon through a telescope, one can observe various impact craters of different sizes covering its surface. The most notable surface features visible to the naked eye, often referred to as \"the man in the Moon,\" consist of large dark lava flows. Early lunar observers from centuries ago mistakenly believed the Moon had continents and oceans, naming the dark areas \"seas\" (maria in Latin). These names, like Mare Nubium and Mare Tranquillitatis, are still used today. While the land areas between the seas remain unnamed, thousands of craters have been named after great scientists and philosophers, such as Plato and Copernicus. The Moon\'s geological history is distinct from Earth due to its lack of internal activity, air, and water. Lunar features like craters and mountains have origins different from their terrestrial counterparts and do not resemble Earth\'s geological processes. ### Lunar History To understand the Moon\'s history, we must date individual rocks. Apollo astronauts brought back lunar samples for radioactive dating, revealing ages ranging from 3. 3 to 4. 4 billion years. This is older than most Earth rocks. Earth and the Moon formed around 4. 5 to 4. 6 billion years ago. The Moon\'s crust is mainly anorthosites, making up 83% of the lunar highlands. These rocks solidified early in the Moon\'s history, between 4. 1 and 4. 4 billion years ago, and are heavily cratered from impacts by debris over billions of years. The highlands\' low-density rock resembles slag floating on a smelter\'s surface, showing the Moon\'s ancient history of violent collisions. Unlike Earth\'s mountains, the Moon\'s highlands lack sharp folds and have low, rounded profiles similar to significantly eroded mountains on Earth. The absence of atmosphere and water on the Moon has prevented the formation of cliffs and sharp peaks typically seen on Earth. Instead, gradual erosion from impact cratering has shaped the smooth features of the highlands over time. Maria on the Moon have fewer craters than highlands, covering 17% of the lunar surface mainly on the Earth-facing side. ([Figure 5.6](#_bookmark13)). The dark-colored basalt found in the maria on the Moon was formed billions of years ago from volcanic lava. These lava flows filled impact basins created by collisions with large objects early in the Moon\'s history. This basalt is similar to Earth\'s ocean crust and terrestrial volcanic lavas. The Mare Orientale is the youngest lunar impact basin. Volcanic activity on the Moon began early but mainly ended about 3. 3 billion years ago. Since then, the Moon\'s interior has cooled, with limited volcanic activity in small areas. The primary surface changes now come from external forces rather than internal volcanic activity. The Moon\'s history of volcanic activity dates back to its early beginnings. ### On the Lunar Surface "The surface is fine and powdery. I can pick it up loosely with my toe. But I can see the footprints of my boots and the treads in the fine sandy particles." ---Neil Armstrong, Apollo 11 astronaut, immediately after stepping onto the Moon for the first time. The Moon\'s surface is covered in a fine soil made up of tiny rock fragments. Astronauts\' footprints kicked up dark basaltic dust from lunar maria, spreading it throughout their equipment. The upper layers of the surface are porous, with dust packed in loosely causing boots to sink several centimeters deep. This lunar dust is a result of impacts over billions of years, breaking up the rock surface into small particles. As a result, much of the Moon\'s surface layer is now composed of particles the size of dust or sand due to continuous cratering events. Due to the lack of air, the temperature on the lunar surface varies greatly, reaching boiling points during the day and dropping to extremely low temperatures at night. The Moon\'s porous dusty soil cools quickly, causing temperatures to plummet to about -173°C. Despite being at a similar distance from the Sun as Earth, the Moon\'s surface experiences more drastic temperature changes due to the absence of an atmosphere to regulate heat. In contrast, Earth\'s atmosphere helps to moderate temperature extremes. Impact Craters -------------- ### Volcanic Versus Impact Origin of Craters The Moon serves as a valuable tool for understanding the history of our solar system, as it retains a preserved record of impact history unlike Earth, which has erased much of its own through geological activity. By studying the Moon, we can potentially gain insights that can be applied to other celestial bodies. Our Moon, being in close proximity to Earth for over 4 billion years, offers a unique perspective on planetary history. Initially, scientists mistakenly believed lunar craters were volcanic in origin due to the rarity of impact craters on Earth. It wasn\'t until Grove K. Gilbert proposed in the 1890s that lunar craters were the result of impacts, pointing out their distinct characteristics compared to volcanic craters on Earth. His hypothesis, though initially rejected, laid the groundwork for modern lunar geology. Gilbert\'s observation of large, circular, mountain-rimmed lunar craters with floors below surrounding plains contrasted with the smaller, deeper volcanic craters on Earth. This realization challenged the prevailing beliefs of the time and expanded our understanding of our Moon\'s unique geological features. Gilbert\'s research showed that lunar craters result from impacts, with their circular shape due to escape velocity. This speed is needed to break free from a body\'s gravity, ensuring incoming projectiles hit with a specific force. For Earth, this speed is 11 km/s, and for the Moon, it\'s 2. 4 km/s. The impact energy creates symmetrical explosions, excavating material symmetrically. Bomb and shell craters on Earth exhibit circular shapes due to explosions, resembling impact craters. After World War I, scientists recognized the similarities between explosion and impact craters, validating Gilbert\'s hypothesis. Unfortunately, Gilbert passed away before witnessing widespread acceptance of his theory. ### The Cratering Process At high velocities, a collision generates a crater. Upon impact, a fast-moving object can traverse two to three times its own diameter before coming to a halt. The object\'s kinetic energy is then transformed into a shock wave that permeates the target and into heat, which vaporizes the projectile and some of the surrounding material. The shock wave causes the target\'s rock to fracture, while the expanding silicate vapor creates an explosion similar to that of a nuclear bomb detonated at ground level (Figure 5.10). The size of the resulting crater is primarily determined by the impact speed, typically being 10 to 15 times the projectile\'s diameter. The type of impact explosion described earlier results in a specific type of crater, as demonstrated in Figure 5.10. Initially, the central depression has a bowl-like shape (the term \"crater\" originates from the Greek word for \"bowl\"), but the crust\'s rebound partially fills it, resulting in a flat floor and potentially forming a central peak. The rim of the crater features terraces created by landslides. The force of the explosion causes the rim of the crater to be raised, elevating it above both the floor and the surrounding terrain. An ejecta blanket surrounds the rim, composed of material expelled by the explosion. This debris descends, forming a rugged, hilly area, usually about the same width as the diameter of the crater. Higher-speed ejecta falls further from the crater, sometimes producing small secondary craters upon impact. Streams of ejecta can extend for hundreds or even thousands of kilometers from the crater, resulting in the prominent bright crater rays seen in lunar photographs taken near the full phase. The brightest lunar crater rays are linked to large, recently formed craters such as Kepler and Tycho. ### Using Crater Counts The calculations for the Moon suggest that a 1-kilometer crater forms approximately every 200,000 years, a 10-kilometer crater forms every few million years, and one or two 100-kilometer craters form every billion years, assuming the cratering rate has remained constant. These calculations indicate that it would have taken several billion years to create all the craters visible in the lunar maria, aligning with the age determined for the maria from radioactive dating of returned samples, which is 3.3 to 3.8 billion years old. The agreement between these two calculations supports the original assumption that comets and asteroids, in roughly their current numbers, have been impacting planetary surfaces for billions of years. However, there are strong indications that prior to 3.8 billion years ago, the impact rates were significantly higher. This is evident when comparing the numbers of craters on the lunar highlands with those on the maria - typically, there are 10 times more craters on the highlands than on a similar area of maria. Yet, the radioactive dating of highland samples suggests that they are only slightly older than the maria, typically 4.2 billion years rather than 3.8 billion years. If the impact rate had been consistent throughout the Moon\'s history, the highlands would have needed to be at least 10 times older, suggesting a formation 38 billion years ago - before the universe itself began. In scientific inquiry, when an assumption yields an improbable conclusion, the assumption must be re-evaluated. In this case, the contradiction is resolved by considering that the impact rate varied over time, with a much more intense bombardment occurring prior to 3.8 billion years ago, which resulted in most of the craters visible today in the highlands. The concept we have been investigating, that significant collisions (particularly in the early stages of the solar system) had a significant influence on the formation of the celestial bodies we observe, is not exclusive to our examination of the Moon. While you go through the remaining sections about the planets, you will come across additional evidence suggesting that many of the current characteristics of our system might stem from its tumultuous history. The Origin of the Moon ---------------------- ### Ideas for the Origin of the Moon Modern science tends to inquire about the origins of things. Planetary scientists have found it challenging to comprehend how the Moon came into being. The main obstacle stems from our extensive knowledge of the Moon, which is unusual in the field of astronomy. One significant issue is the Moon\'s intriguing resemblance to Earth and its frustrating disparities. Earlier theories about the Moon\'s origin generally fell into three broad categories. 1. The fission theory--- the Moon was originally a part of Earth but became separated from it during their early history. 2. The sister theory--- the Moon formed together with (but independent of) Earth, as we believe many moons of the outer planets formed. 3. The capture theory--- the Moon originally formed in another part of the solar system and was later captured by Earth. The capture theory, fission hypothesis, and co-formation theory all present challenges when explaining the origin of the Moon. The capture theory faces criticism due to the lack of a plausible mechanism for Earth to capture such a large moon, as well as the expected eccentric orbit of a captured object. The fission hypothesis, suggesting the Moon separated from Earth, is now considered impossible based on modern calculations and the difficulty of explaining the chemical differences between Earth and the Moon. The co-formation theory, proposing that the Moon formed alongside Earth, or a modified fission hypothesis, are the remaining options. However, as scientists uncover more about the Moon\'s composition and characteristics, these traditional explanations become less convincing. Notably, the similarities in oxygen isotopes between Earth and the Moon raise doubts about a completely independent origin for our celestial neighbor, challenging researchers to find a more viable explanation for how the Moon came to be. Mercury ------- ### Mercury's Orbit Mercury, much like the Moon, lacks an atmosphere and features a heavily cratered surface. It also shares with the Moon the potential for a violent birth described later in this chapter. Being the closest planet to the Sun, Mercury has the shortest period of revolution around the Sun (88 of our days) and the highest average orbital speed (48 kilometers per second) in accordance with Kepler's third law. Its name is fitting as it is named after the fleet-footed messenger god of the Romans. Due to its proximity to the Sun, Mercury can be challenging to spot in the sky and is best observed when its eccentric orbit takes it farthest from the Sun. The semimajor axis of Mercury's orbit, which denotes the planet's average distance from the Sun, is 58 million kilometers, or 0.39 AU. However, due to its orbit\'s high eccentricity of 0.206, Mercury\'s actual distance from the Sun ranges from 46 million kilometers at perihelion to 70 million kilometers at aphelion (the concepts and terms related to orbits were introduced in Orbits and Gravity). ### Composition and Structure Mercury is the smallest terrestrial planet, with a mass that is one-eighteenth that of Earth. Its diameter of 4878 kilometers is less than half the size of Earth\'s. A density of 5.4 g/cm3 makes Mercury much denser than the Moon, suggesting a substantial difference in composition between the two objects. The composition of Mercury makes it unique among the planets and is one of its most interesting aspects. The planet\'s high density indicates that it is likely composed mainly of heavy materials, such as metals. Current models of Mercury\'s interior propose that 60% of its total mass consists of a metallic iron-nickel core, while the rest is primarily made up of silicates. The core, with a diameter of 3500 kilometers, extends to within 700 kilometers of the surface. A way to envision Mercury is as a metal ball the size of the Moon surrounded by a 700-kilometer-thick rocky crust (Figure 5.13). In contrast to the Moon, Mercury possesses a weak magnetic field, which is consistent with the presence of a large metal core. The existence of this magnetic field suggests that at least a portion of the core must be liquid in order to generate the observed magnetic field. ### Mercury's Strange Rotation The surface features of Mercury were originally interpreted as evidence of the planet showing the same face to the Sun, much like the Moon does to Earth. Consequently, it was commonly accepted that Mercury\'s rotation period matched its 88-day revolution period, resulting in one side being constantly hot and the other perpetually cold. However, radar observations of Mercury in the 1960s definitively demonstrated that the planet does not always keep one side facing the Sun. When a planet rotates, one side appears to be approaching Earth while the other side is moving away from it. As a result, the transmitted radar-wave frequency is spread or widened into a range of frequencies in the reflected signal, as depicted in Figure 5.14. The extent of this widening provides a precise measurement of the planet\'s rotation rate. Mercury takes 59 days to rotate with respect to the distant stars, a period that is two-thirds of its revolution around the Sun. It has been observed by astronomers that a 2:3 ratio between a planet\'s spin and orbit leads to a stable situation. Due to its proximity to the Sun, Mercury experiences extreme heat on its daylight side, while the lack of a substantial atmosphere causes it to become remarkably cold during its long nights. Surface temperatures on Mercury can soar to 700 K (430 °C) at noontime, but drop to 100 K (--170 °C) just before dawn. Craters near the poles that receive no sunlight experience even lower temperatures. This results in a temperature range of 600 K (or 600 °C) on Mercury, which is greater than that of any other planet. ### The Surface of Mercury In 1974, the US spacecraft Mariner 10 provided the first detailed visual examination of Mercury as it passed within 9500 kilometers of the planet\'s surface and transmitted over 2000 images back to Earth. These images revealed features with a resolution as fine as 150 meters. The MESSENGER spacecraft, launched in 2004, extensively mapped the planet after conducting multiple flybys of Earth, Venus, and Mercury, eventually entering Mercury\'s orbit in 2011. The mission concluded in 2015 with the deliberate crash of the spacecraft onto the planet\'s surface. The surface of Mercury bears a strong resemblance to the Moon in its appearance, marked by thousands of craters and large basins reaching up to 1300 kilometers in diameter. Several of the brighter craters exhibit rays, akin to those seen in Tycho and Copernicus on the Moon, with many exhibiting central peaks. Additionally, Mercury features scarps, or cliffs, stretching over a kilometer in height and hundreds of kilometers in length, as well as ridges and plains. The instruments aboard MESSENGER analyzed the surface composition and documented evidence of past volcanic activity. Among its significant findings, MESSENGER confirmed the presence of water ice -- initially detected via radar -- within craters near the planet\'s poles, resembling the conditions observed on the Moon. Furthermore, the spacecraft unexpectedly discovered organic compounds rich in carbon mixed with the water ice. The majority of features on Mercury are named after artists, writers, composers, and other contributors to the arts and humanities, in contrast to the scientists honored on the Moon. Examples of named craters include Bach, Shakespeare, Tolstoy, Van Gogh, and Scott Joplin. Plate tectonics have not been observed on Mercury. Nonetheless, the planet\'s unique long scarps can sometimes be observed intersecting craters; this implies that the scarps must have formed after the craters (Figure 5.15). These extended, curved cliffs seem to originate from the slight compression of Mercury\'s crust. Evidently, at some point in its history, the planet contracted, causing the crust to wrinkle, and this contraction likely occurred after most of the surface craters had already formed. If Mercury follows the standard cratering chronology, this contraction must have happened within the past 4 billion years and not during the early period of heavy bombardment in the solar system. The Nearest Planets: An Overview -------------------------------- Mars and Venus, close neighbors in the night sky, are both bright objects. Mars is typically about 227 million kilometers from the Sun, while Venus orbits at a distance of 108 million kilometers. Venus has a nearly circular orbit and is sometimes seen as an \"evening star\" or \"morning star. \" It comes closer to Earth than any other planet, at a minimum distance of 40 million kilometers. Mars, on the other hand, is closest to Earth at about 56 million kilometers. ### Appearance Venus is bright and shows phases like the Moon when viewed through a telescope. Galileo observed its full range of phases, using them to prove that Venus orbits the Sun, not Earth. The planet\'s surface is hidden by thick, reflective clouds that block about 70% of incoming sunlight, making it difficult to study with cameras from orbit (Figure 5.16) Through a telescope (Figure 5.16), Mars appears incredibly intriguing when compared with Venus. The planet\'s distinct **red** color is now known to be a result of the presence of iron oxides in its soil. This color may explain its connection with conflict (and blood) in the stories of ancient societies. Telescopes on the ground can achieve a resolution of about 100 kilometers, similar to what can be observed on the Moon with the naked eye. At this resolution, no signs of topographic features are visible: no mountains, no valleys, and not even impact craters. However, bright polar ice caps are easily visible, along with faint surface markings that occasionally change in shape and intensity from one season to another. During the late 1800s and early 1900s, certain astronomers believed they had found signs of an advanced civilization on Mars. The debate originated in 1877 after Italian astronomer Giovanni Schiaparelli (1835--1910) reported observing long, faint, straight lines on Mars, which he referred to as canale, or channels. Due to translation errors, the term \"canale\" was misinterpreted as \"canals\" in English-speaking countries, suggesting an artificial origin. Prior to Schiaparelli\'s findings, astronomers had already noted the fluctuating size of the bright polar caps in relation to the seasons and observed changes in the dark surface features of Mars. Using their imagination, they visualized the canals as extensive fields of crops bordered by irrigation channels, bringing water from the melting polar ice to the arid deserts of the red planet. (They assumed the polar caps were primarily composed of water ice, which is not entirely accurate, as we will address later on.) Percival Lowell, a self-made American astronomer and member of the affluent Lowell family of Boston, was the most notable advocate for the existence of intelligent life on Mars until his passing in 1916. A talented writer and speaker, Lowell presented a compelling case to the public about the existence of intelligent Martians, who, according to him, had constructed the vast canals to sustain their civilization amidst a deteriorating climate (Figure 5.17). The argument for intelligent Martians was based on the existence of canals on Mars, a topic of debate among astronomers. The markings were challenging to study due to atmospheric conditions causing Mars to appear blurry in telescopes. While Lowell believed in the canals, others were skeptical and could not see them. Larger telescopes disproved the presence of canals, leading skeptics to feel validated. It is now understood that the straight lines were an optical illusion, a product of the human mind\'s pattern recognition. When observing faint surface markings, our minds tend to connect them into straight lines. ### Rotation of the Planets Astronomers have accurately determined that Mars has a rotation period of 24 hours, 37 minutes, and 23 seconds, slightly longer than that of Earth. This precision was achieved by observing the planet\'s surface markings over a long period of time. Mars has a tilt of about 25°, leading to seasons similar to Earth\'s but lasting about six months each due to its longer year. In contrast, Venus\'s rotation period of 243 days was determined using radar signals due to the inability to see surface details through its thick clouds. Surprisingly, Venus rotates in a backward or retrograde direction. This slow rotation results in a Venusian day being longer than a year, with the Sun taking 117 Earth days to return to the same position in the sky. This unique calendar system on Venus is a result of its slow rotation potentially caused by powerful collisions during the solar system\'s formation. The rotation periods and unique features of Mars and Venus provide intriguing insights into the differences between these two planets and Earth. From seasonal variations on Mars to the backward rotation of Venus, each planet\'s characteristics offer valuable information for astronomers studying planetary dynamics and formation processes. Observing and understanding these celestial bodies enhance our knowledge of the vast and diverse universe we inhabit. ### Basic Properties of Venus and Mars Comparing some of their basic properties with each other and with Earth (Table 5.4) before discussing each planet individually is essential. Venus, in many aspects, is similar to Earth, with a mass 0.82 times that of Earth and a density that is almost identical. The level of geological activity on Venus has also been relatively high, almost as high as on Earth. However, Venus\' atmosphere is vastly different from Earth\'s, with a surface pressure nearly 100 times greater than Earth\'s. Additionally, the surface of Venus is extremely hot, with temperatures reaching 730 K (over 850 °F), surpassing the self-cleaning cycle of an oven. Understanding why Venus\' atmosphere and surface environment have diverged so significantly from Earth\'s poses a major challenge. Mars, on the other hand, is relatively small, having a mass that is only 0.11 times that of Earth. However, it is larger than both the Moon and Mercury and unlike them, it still has a thin atmosphere. In the distant past, Mars was big enough to experience significant geological activity. What makes Mars most intriguing is that it likely had a dense atmosphere and liquid water bodies long ago, conditions that are linked to the potential for life development. It\'s even possible that some form of life survives today in protected areas beneath the surface of Mars. The Geology of Venus -------------------- Venus, similar in size and composition to Earth, lacks Earth\'s plate tectonics and erosion processes, resulting in a unique surface appearance. ### Spacecraft Exploration of Venus Nearly 50 spacecraft have been sent to Venus, but only about half of them succeeded. The Soviet Union led most of the missions after the 1962 US Mariner 2 flyby. In 1970, Venera 7 became the first probe to land on Venus and transmit data before succumbing to the extreme surface heat after just 23 minutes. More Venera probes followed, capturing images and studying the atmosphere and soil of the planet. Despite the cloud cover surrounding Venus, a radar instrument was used to create the first global radar map by the US Pioneer Venus orbiter in the late 1970s, followed by the Soviet Venera 15 and 16 radar orbiters in the early 1980s. The most detailed mapping of Venus was done by the US Magellan spacecraft, which provided images with 100-meter resolution. The spacecraft returned massive amounts of data, more than all previous planetary missions combined, giving us a comprehensive view of Venus\' surface. Magellan\'s radar has a resolution of 100 meters, allowing images of Venus surface features larger than a football field. This reveals diverse topographic features previously unseen. Radar images in this chapter are constructed from radar reflections, not visible-light photos. Bright areas indicate rough terrain, while dark regions show smoother surfaces. ### Probing Through the Clouds of Venus Venus\' radar maps reveal a planet that resembles Earth\'s surface before erosion and deposition changed it. With no water or ice and low wind speeds, Venus shows unique geological features from crust movements, volcanic eruptions, and impact craters. The planet\'s surface, uncovered beneath its clouds, holds the history of millions of years of geological activity. Around 75% of Venus consists of lowland lava plains that resemble Earth\'s basaltic ocean basins but were created differently without subduction zones. Venus never experienced plate tectonics, although mantle convection caused stresses in its crust. The lava plains formed similarly to the lunar maria, with widespread eruptions instead of plate movements. Two continents, Aphrodite and Ishtar, stand out on Venus. Aphrodite, about the size of Africa, is the largest, stretching along the equator. Ishtar, similar to Australia in size, contains the Maxwell Mountains, rising 11 kilometers above the lowlands. These mountains are named after James Clerk Maxwell, while other features on Venus are named after women from history or mythology. ### Craters and the Age of the Venus Surface Astronomers used Magellan high-resolution images to determine the age of Venus\' surface by counting impact craters. The age of a planetary surface indicates its geological activity, not the planet\'s age. Figure 5.20 shows what these craters look like on radar images of Venus. The surface age is determined by the density of craters, with more craters indicating an older surface. The largest crater on Venus, Mead, is 275 kilometers in diameter, larger than the largest known terrestrial crater (Chicxulub) but smaller than lunar impact basins. The thick atmosphere of Venus offers some protection from smaller projectiles, with craters less than 10 kilometers in diameter indicating that projectiles smaller than 1 kilometer were stopped. Larger projectiles, from 10 to 30 kilometers, often break apart in the atmosphere before reaching the ground, leading to distorted or multiple craters. Craters with diameters of 30 kilometers or more provide insights into Venus\' surface age, similar to using them on airless bodies like the Moon. The large, fresh craters on Venus\' plains suggest an average surface age of 300 to 600 million years, highlighting persistent geological activity unlike that of Earth\'s younger, more active ocean basins, but not as old as its less active continents. Limited erosion or sediment deposition indicates little change since large-scale volcanic activity resurfaced the venusian plains between 300 and 600 million years ago, a unique event in the planet\'s history. This suggests Venus underwent a planet-wide volcanic convulsion, leaving its surface relatively untouched since. ### Volcanoes on Venus Venus, like Earth, has experienced widespread volcanism which renews its surface through volcanic eruptions in lowland plains. Fluid lava flows destroy old craters, generating a fresh surface. Younger volcanic mountains and hot spots are also present on Venus, where mantle convection transports heat to the surface. The largest volcano, Sif Mons, is 500km wide and 3km high, with a 40km wide caldera at its top and lava flows up to 500km long on its slopes. Thousands of smaller volcanoes, including unusual shapes like \"pancake domes,\" are found on the surface. Most resemble terrestrial volcanoes, while others have unique characteristics. The Magellan images show these features, with some as small as a shopping mall parking lot. Volcanic activity occurs when lava erupts onto a planet\'s surface. However, not all upwelling lava from the planet\'s interior reaches the surface. On Earth and Venus, this upward-moving lava can gather and create protrusions in the planet\'s crust. Many of Earth\'s granite mountain ranges, like the Sierra Nevada in California, are formed from this type of underground volcanic activity. These protrusions are prevalent on Venus, where they form large circular or oval features known as coronae (plural: corona) (See Figure 5.22). ### Tectonic Activity Convection currents in Venus\' mantle cause tectonic forces that shape the planet\'s crust. These forces create tectonic features like ridges and cracks on the lowland plains, including rift valleys and coronae. The Maxwell Mountains in the Ishtar continent, with the highest elevations on Venus, are a prominent example of tectonic forces at work. Comparable to Earth\'s Tibetan Plateau and Himalayan Mountains, these features are formed by crustal compression and sustained by ongoing mantle convection. Overall, tectonic forces play a crucial role in shaping Venus\' geology, creating diverse and striking landscapes across the planet. ### On Venus' Surface Venera landers successfully explored Venus in the 1970s, facing extreme conditions with temperatures hot enough to melt lead and zinc, and a surface pressure of 90 bars. Despite this, they managed to take photos, collect samples, and analyze the chemistry before their instruments failed. The sunlight was red due to clouds, and the light was similar to a cloudy day on Earth. The probes discovered igneous rock, mainly basalts, on the planet\'s surface. The images captured show a barren landscape with various rocks, some potentially impact debris, and layered lava flows. NASA plans to launch a radar orbiter to study Venus in more detail, specifically focusing on the Aphrodite region, which may have evidence of geologic plates and past tectonic activity like Earth\'s. No further landings have been attempted since the 1970s. The Massive Atmosphere of Venus ------------------------------- Venus has a dense atmosphere that creates high surface temperatures and a perpetual red twilight. Sunlight cannot reach the surface directly through the thick clouds, but diffuse light illuminates it to a similar level as Earth under heavy overcast. The weather at the bottom of Venus\' deep atmosphere is consistently hot and dry, with calm winds. Due to the heavy cloud cover, all surface locations experience the same weather conditions on Venus. ### Composition and Structure of the Atmosphere Venus has a carbon dioxide-dominated atmosphere, making up 96% of its composition, with nitrogen as the second most abundant gas. If Earth didn\'t have carbon dioxide locked up in marine sediments, its atmosphere would also be primarily carbon dioxide. Table 10. 2 shows a comparison of the atmospheres of Venus, Mars, and Earth, revealing similar gas proportions but dramatically different total quantities. Venus\'s atmosphere is more than 10,000 times heavier than Mars\'s due to its high surface pressure of 90 bars. Venus\'s atmosphere lacks water, distinguishing it from Earth. Between 30 and 60 kilometers above the surface, there is a dense cloud layer in the upper troposphere that mainly consists of sulfuric acid droplets. Sulfuric acid (H~2~SO~4~) is created by the chemical reaction between sulfur dioxide (SO~2~) and water (H~2~O). On Earth, sulfur dioxide is a key gas released by volcanoes, but it gets diluted and removed by precipitation. However, in the dry atmosphere of Venus, this substance seems to stay stable. Below 30 kilometers, Venus\'s atmosphere is free of clouds. ### Surface Temperature on Venus In the late 1950s, radio astronomers discovered the high surface temperature of Venus, a finding that was later confirmed by the Mariner and Venera probes. Despite its proximity to the Sun being closer than Earth\'s, Venus\'s surface is several hundred degrees hotter than what would be expected from the additional sunlight it receives. This led scientists to question why Venus\'s surface was heated to temperatures exceeding 700 K, and the explanation ultimately lay in the **greenhouse effect**. Venus experiences the greenhouse effect similarly to Earth, but due to its incredibly high levels of CO2, which are almost a million times greater, the effect is much more potent. The dense CO2 acts like a blanket, making it difficult for the heat radiation from the ground to escape back into space, resulting in the heating of the surface. The only way for the energy balance to be restored is when the planet radiates as much energy as it receives from the Sun, which only occurs when the temperature of the lower atmosphere is exceptionally high. The greenhouse heating process raises Venus\' surface temperature until this energy equilibrium is attained. It is of interest to investigate whether Venus has always maintained such a massive atmosphere and high surface temperature, or if it evolved from a climate resembling Earth in the past. This question gains significance as we observe the rising CO2 levels in Earth's atmosphere. With the greenhouse effect intensifying on Earth, there is a concern about the potential of transforming our planet into an inhospitable environment akin to Venus. Let\'s attempt to reconstruct Venus\' likely evolution from an Earth-like beginning to its current state. Venus may have initially possessed a climate resembling Earth\'s, with moderate temperatures, liquid water oceans, and a significant portion of its CO2 dissolved in the oceans or chemically bonded with the surface rocks. Subsequently, with a modest increase in energy output from the Sun, we consider how Venus\' atmosphere would react to such changes. The analysis shows that even a slight increase in heat can lead to greater water evaporation from the oceans and the release of gas from the surface rocks. This process contributes to a further rise in atmospheric CO2 and H2O, gases that would enhance the greenhouse effect in Venus' atmosphere, resulting in increased heat near its surface and the release of more CO2 and H2O. Without intervention by other processes, the temperature continues to escalate. This scenario is referred to as the **runaway greenhouse effect**. The runaway greenhouse effect is more than just a large greenhouse effect; it is an evolutionary process. The atmosphere transitions from a small greenhouse effect, like on Earth, to a situation with significant greenhouse warming, like on Venus today. Once these large greenhouse conditions develop, the planet reaches a new, much hotter equilibrium near the surface. Reversing this situation is challenging due to the role of water. On Earth, most CO2 is bound in rocks or dissolved in oceans. Venus lost its oceans as it heated up, removing a crucial safety valve. The water vapor in its atmosphere can\'t last forever due to ultraviolet light from the Sun. The loss of water is irreversible once it\'s gone. Evidence suggests this happened on Venus. It\'s unknown if Earth could experience a similar runaway greenhouse effect in the future. However, Venus serves as a warning that a planet can\'t keep heating indefinitely without significant changes in its oceans and atmosphere. This highlights the importance of closely monitoring our planet\'s climate for future generations. The Geology of Mars ------------------- Mars is more appealing than Venus as it is more hospitable. Surface features and seasonal changes on Mars can be observed from Earth. While dry and cold now, evidence from spacecraft indicates Mars once had blue skies and lakes of liquid water. It is a potential destination for astronauts and could potentially support permanent bases in the future. ### Spacecraft Exploration of Mars Over 50 spacecraft have been launched towards Mars, with only about half being successful. The first one, Mariner 4 in 1965, transmitted 22 photos to Earth, revealing a barren planet with impact craters. Mariner 9 in 1971 became the first spacecraft to orbit Mars, discovering volcanic features, canyons, and evidence of water channels. The Viking missions in the 1970s were successful, with two landers conducting experiments in search of life on Mars. However, no evidence of life was found. Mars was unvisited for two decades after Viking, with two failed missions by NASA and the Russian Space Agency. Despite the setbacks, spacecraft exploration of Mars has provided valuable insights into its geological features and history, challenging the belief that Mars is a \"dead planet. \" Each mission has contributed to our understanding of Mars, from its surface to its potential for hosting life, and continues to inspire further exploration of the red planet. In the 1990s, NASA initiated a new exploration program using smaller and more cost-effective spacecraft compared to Viking. The first mission, Pathfinder, successfully landed a solar-powered rover on Mars on July 4, 1997. Following this, Mars Global Surveyor (MGS) provided detailed high-resolution images of the Martian surface over the course of a Martian year. It made a remarkable discovery of gullies believed to be formed by surface water. Subsequent missions, such as Mars Odyssey orbiter and Mars Express orbiter, further expanded exploration capabilities with high-resolution cameras and a gamma-ray spectrometer revealing subsurface hydrogen, potentially frozen water. Other orbiters like Mars Reconnaissance Orbiter, MAVEN, and India\'s Mangalayaan were launched to evaluate landing sites, study the atmosphere, and explore Mars\' thin atmosphere layers respectively. These missions also enhance communication with landers and rovers on the surface and transmit data back to Earth. In 2003, NASA launched the Mars Exploration Rovers Spirit and Opportunity, which far exceeded their initial goals. They traveled over 50 kilometers together, impressively surpassing their 600-meter target. Opportunity navigated the Victoria impact crater and successfully climbed out after encountering difficulties. Dust storms temporarily affected the rovers\' power supply, but they resumed operation once the dust was blown away. The rovers were strategically positioned on slopes to maximize solar power during winter. In 2006, Spirit faced technical issues but continued to function as a stationary research station. Additionally, Phoenix, constructed from spare parts, successfully landed near the north polar cap in 2008, directly detecting water ice in the Martian soil. In 2011, NASA launched its largest Mars mission since Viking with the 1-ton rover Curiosity, powered by plutonium. Curiosity landed in Gale crater, known for its complex geology and past water submersion. Unlike previous missions, Curiosity aimed to study climate, geology, and Mars\' habitability. In 2018, NASA\'s InSight Lander arrived on Mars with scientific instruments but no life detection tools. The mission included \"the mole\" to dig into Mars\' surface gradually. In 2020, the rover Perseverance, similar to Curiosity, landed in a former lakebed to collect samples for Earth analysis. NASA\'s helicopter drone, Ingenuity, has flown 69 missions, exceeding expectations, covering 17 kilometers and reaching altitudes of 24 meters. The drone provided essential scouting for the Perseverance mission, exploring the Martian terrain. The focus on potential life detection on Mars has been renewed through these missions, progressing scientific exploration and pushing the boundaries of space exploration. The missions have provided valuable data on Mars\' landscape, geology, and the possibility of ancient life forms, enhancing our understanding of the Red Planet. ### Global Properties of Mars The diameter of Mars is 6790 kilometers, slightly more than half the size of Earth, which results in a total surface area almost equal to the land area of our planet. With an overall density of 3.9 g/cm3, Mars is believed to be primarily composed of silicates with a small metal core. Although Mars doesn\'t have a global magnetic field, there are zones of strong surface magnetization indicating the presence of a global field billions of years ago. Currently, it seems that there is no conductive liquid material in Mars\' core. Thanks to the Mars Global Surveyor, we have successfully mapped the entire planet. The onboard laser altimeter conducted millions of precise measurements of the planetary surface, accurate to within a few meters. This precision allowed us to observe even the yearly deposition and evaporation of the polar caps. Similar to Earth, the Moon, and Venus, Mars exhibits both continental or highland regions and extensive volcanic plains. The total variation in elevation from the highest mountain (Olympus Mons) to the deepest basin (Hellas) is 31 kilometers. Roughly half of the planet is made up of heavily cratered highland terrain, mainly located in the southern hemisphere. The remaining half, mostly situated in the north, contains younger, lightly cratered volcanic plains at an average elevation approximately 5 kilometers lower than the highlands. It\'s worth noting that we\'ve observed a similar pattern on Earth, the Moon, and Venus. The geological division into older highlands and younger lowland plains appears to be a common characteristic among all terrestrial planets except Mercury. Spanning the north-south division of Mars is an uplifted continent equivalent in size to North America. This continent is the Tharsis bulge, which rises 10 kilometers high and is a volcanic region dominated by four significant volcanoes that soar even higher into the Martian sky. ### Volcanoes on Mars The Martian lowland plains bear a strong resemblance to the lunar maria and have a similar frequency of impact craters. These plains, much like the lunar maria, likely took shape between 3 and 4 billion years ago. It appears that Mars underwent widespread volcanic activity around the same period as the Moon, resulting in the formation of comparable basaltic lavas. In the Tharsis region, the largest volcanic mountains on Mars can be observed, while smaller volcanoes are scattered across much of the planet\'s surface. Olympus Mons (Mount Olympus), the most remarkable volcano on Mars, boasts a diameter exceeding 500 kilometers and a summit towering over 20 kilometers above the surrounding plains---three times taller than Earth's tallest mountain (Figure 5.27). The volume of this colossal volcano is nearly 100 times greater than that of Hawaii's Mauna Loa. If placed on Earth, Olympus Mons would cover an area larger than the entire state of Missouri. The use of images captured from space enables scientists to seek out impact craters on the sides of these volcanoes to gauge their age. A significant number of the volcanoes display numerous such craters, indicating that their activity ceased over a billion years ago. However, Olympus Mons has very few impact craters. Its current surface is likely no more than around 100 million years old, and it might even be much younger. Some of the recent-looking lava flows could have been produced a hundred years ago, or a thousand, or a million, but from a geological standpoint, they are relatively young. This suggests to geologists that Olympus Mons could still be periodically active today---something that potential future Mars developers should consider. Martian Cracks and Canyons -------------------------- The Tharsis bulge on Mars features impressive geological formations, including massive volcanoes. The bulging surface, caused by intense pressure from below, has led to extensive tectonic cracking of the planet\'s crust. One of the most remarkable tectonic features is the Valles Marineris, stretching about 5000 kilometers along the slopes of the Tharsis bulge. On Earth, this canyon system would span from Los Angeles to Washington, DC. The main canyon is around 7 kilometers deep and up to 100 kilometers wide, large enough to fit the Grand Canyon of the Colorado River comfortably within one of its side canyons. In the movie \"The Martian,\" viewers can witness a recreation of these canyonlands as the protagonist embarks on a journey through this extraordinary Martian landscape. The Valles Marineris canyons, despite being termed as such, were not formed by running water but rather by tectonic forces. The Tharsis uplift, which caused crustal tensions, also contributed to the creation of these canyons. However, water has played a role in shaping them through seepage from deep springs that undercut the cliffs, leading to landslides that widened the cracks into the valleys seen today. Erosion in the canyons is now primarily due to wind. Mars appears to have fewer tectonic structures compared to Venus, possibly due to lower geological activity on the smaller planet. Evidence of widespread faulting may have been buried by wind-deposited sediment, much like Earth conceals its geological history under layers of soil. ### The View on the Martian Surface The initial spacecraft to achieve successful landings on Mars encompassed Vikings 1 and 2 in addition to Mars Pathfinder. They all transmitted images displaying a barren yet oddly captivating scenery, featuring various angular rocks mixed with formations of dune-like, fine-grained, reddish soil (See Figure 5.29). All three landers touched down on flat, lowland terrain on Mars and discovered that the soil was composed of clays and iron oxides, consistent with the planet\'s red color. The rocks found were volcanic in origin. Later landers landed in areas that seemed to have been flooded in the past, where sedimentary rock layers formed in the presence of water are common, although most of Mars is covered in wind-blown dust. The Viking landers had weather stations that operated for several years, recording temperatures that varied significantly with the seasons due to the lack of oceans and clouds. In summer, temperatures reached 240 K at Viking 1, dropping to 190 K just before dawn. The lowest temperatures measured by Viking 2 were about 173 K. Winter temperatures at Viking 2 also revealed water frost deposits. Mars experiences mostly light winds, but intense windstorms can blanket the planet in dust, stripping loose material from the surface. Dust devils are common on Mars and play a role in lifting dust into the atmosphere, creating patterns on the ground. Wind also redistributes surface material, leading to the formation of sand dunes on top of lighter material. Material from canyons has been deposited in extensive dune fields at high latitudes on Mars. Water and Life on Mars ---------------------- Mars is believed to offer the best chance of finding evidence of past or present life within our solar system. Scientists focus on searching for signs of life by following the existence of water, a key ingredient for life as we know it on Earth. This approach guides the exploration of Mars, with the hope that uncovering liquid water will eventually lead to discovering signs of life. By studying the water on the red planet, researchers aim to unlock the mysteries of potential life on Mars. ### Atmosphere and Clouds on Mars The current atmospheric pressure on Mars is an average of only 0.007 bar, which is less than 1% of Earth\'s pressure at approximately 30 kilometers above the Earth\'s surface. The main components of the Martian air are carbon dioxide (95%), nitrogen (about 3%), and argon (approximately 2%). These proportions of gases are similar to those in Venus\'s atmosphere as shown in Table 10.2, but in the thin Martian air, there is much less of each gas. Although the winds on Mars can achieve high speeds, they apply much less pressure compared to winds of the same velocity on Earth due to the thin atmosphere. However, the wind is capable of lifting extremely fine dust particles, which can lead to planet-wide dust storms. It is this fine dust that covers almost the entire surface, giving Mars its characteristic red hue. In the absence of surface water, wind erosion plays a significant role in shaping the Martian Liquid water is not stable under current conditions on Mars, due to the low temperatures and low pressure on the planet. Even if the temperature rises above freezing on a sunny summer day, the low pressure prevents the existence of liquid water on the surface, except at the lowest elevations. At a pressure of less than 0.006 bar, water changes directly from solid to vapor without an intermediate liquid state. Salts dissolved in water lower its freezing point, allowing salty water to exist in liquid form on the martian surface under certain conditions. Various types of clouds can form in the martian atmosphere, including dust clouds, water-ice clouds, and CO2 clouds, which are unique to Mars due to the lower temperatures that allow carbon dioxide to condense at high altitudes. ### The Polar Caps The bright polar caps are the most noticeable surface features on Mars when viewed through a telescope, and they undergo seasonal changes, resembling the seasonal snow cover on Earth. While we typically don\'t consider the winter snow in northern latitudes as part of our polar caps, from space, the thin winter snow combines with Earth\'s extensive, permanent ice caps to create a visual effect similar to that observed on Mars (Figure 5.30). The Mars seasonal caps are not made of regular snow but of frozen CO2, also known as dry ice, which condenses directly from the atmosphere when the surface temperature drops below approximately 150 K. These caps form during the cold martian winters and extend down to about 50° latitude by the beginning of spring. The permanent or residual caps, present near the poles, are quite different from the thin seasonal caps of CO2. The southern permanent cap, with a diameter of 350 kilometers, is composed of frozen CO2 deposits and a significant amount of water ice. Throughout the southern summer, it remains at the freezing point of CO2, 150 K, and is thick enough to survive the summer heat intact. In contrast, the northern permanent cap is much larger, never reducing to a diameter less than 1000 kilometers, and is made up of water ice. The north\'s summer temperatures are too high for the frozen CO2 to be preserved. Measurements from the Mars Global Surveyor have revealed the precise elevations in the north polar region of Mars, showing that it is a large basin about the size of our own Arctic Ocean basin. The ice cap itself is approximately 3 kilometers thick, with a total volume of about 10 million km3, similar to Earth's Mediterranean Sea. If Mars ever had extensive liquid water, this north polar basin would have contained a shallow sea. There are indications of ancient shorelines visible, but better images are needed to confirm this suggestion. Orbit images also display a distinctive type of terrain surrounding the permanent polar caps, as shown in Figure 5.30. Above 80° latitude in both hemispheres, the surface is covered with recent layered deposits that overlay the older cratered ground below. Individual layers are usually ten to a few tens of meters thick and are distinguished by alternating light and dark bands of sediment. It is likely that the material in the polar deposits includes dust transported by wind from Mars\' equatorial regions. What do the terraced layers tell us about Mars? These layers indicate that some cyclic process is depositing dust and ice over periods of time. The time scales represented by the polar layers are tens of thousands of years. It seems that the martian climate undergoes periodic changes at intervals similar to those between ice ages on Earth. Calculations suggest that the causes are probably also similar: the gravitational pull of the other planets produces variations in Mars' orbit and tilt through the great clockwork of the solar system. ### Ancient Lakes and Glaciers The Mars rovers, including Spirit, Opportunity, Curiosity, and Perseverance, have explored the planet in search of evidence of water. While they couldn\'t reach the most intriguing sites, like steep gullies, they did investigate potential dried-out lake beds from when Mars had a warmer, thicker atmosphere that allowed for liquid water. Spirit targeted an ancient lake bed in Gusev crater but found it covered by lava flows, hindering access to sedimentary rocks. Opportunity had more success, discovering layered sedimentary rock in a small crater with chemical signs of evaporation, indicating a past shallow salty lake. The rocks also contained hematite-rich spheres, suggesting a watery environment. Curiosity landed in Gale crater and found evidence of past water erosion, including mudstones and sedimentary rocks from an ancient lakebed. Photos from orbit show glaciers beneath Mars\' surface, with some exposed in cliff faces. Perseverance is exploring Jezero crater, a former lakebed and river delta with evidence of ancient floods depositing large rocks. These findings indicate the presence of significant ice below Mars\' surface, potentially forming during warmer periods with higher atmospheric pressure. The discovery of glaciers may provide a future water source for human exploration of Mars. ### Climate Change on Mars The evidence so far indicates that ancient rivers and lakes of water existed on Mars billions of years ago, implying that the planet\'s climate must have been warmer and its atmosphere more substantial than it is today. However, what brought about such drastic changes in Mars\'s climate? One assumption is that, like Earth and Venus, Mars likely had a higher surface temperature initially due to the greenhouse effect. Nonetheless, Mars, being a smaller planet with lower gravity, would have allowed atmospheric gases to escape more easily compared to Earth and Venus. As a result, the loss of atmosphere into space led to a gradual decrease in surface temperature. Eventually, Mars became so cold that most of the water froze out of the atmosphere, further diminishing its heat retention capacity. This led to a reverse greenhouse effect, in contrast to the runaway greenhouse effect on Venus. It is plausible that the loss of atmosphere occurred within a billion years after Mars\'s formation, resulting in the cold and arid Mars we currently know. Despite this, conditions a few meters below the martian surface could be quite different, potentially supporting the persistence of liquid water, especially salty water, aided by the internal heat of Mars or insulating rock layers. Even on the surface, there might be methods to temporarily alter the Martian atmosphere. Mars is likely to undergo long-term climate cycles, possibly driven by changes in the planet\'s orbit and tilt. Periodically, one or both of the polar caps might melt, releasing a considerable amount of water vapor into the atmosphere. It is conceivable that the occasional impact of a comet could create a temporary atmosphere thick enough to permit liquid water on the surface for several weeks or months. Some have even proposed the idea that future technology might enable us to terraform Mars, altering its atmosphere and climate to make the planet more suitable for prolonged human habitation. ### The Search for Life on Mars In the past, if Mars had flowing water, there might have been life. Perhaps some form of life could still exist in the soil on Mars today. The primary goal of the Viking landers in 1976 was to test this unlikely possibility. These landers transported small biological labs to check for microorganisms in the martian soil. The spacecraft's long arm scooped up martian soil and placed it into experimental chambers to isolate and incubate it with various gases, radioactive isotopes, and nutrients. The purpose was to observe any evidence of respiration by living organisms, absorption of nutrients, and gas exchange between the soil and its surroundings. Another instrument analyzed the soil for organic material. If a Viking lander had landed on Earth (barring Antarctica), it would easily have detected life due to the sensitivity of the experiments. However, no life was found on Mars, disappointing many scientists and the public. Although some activity was observed in the soil tests, it was most likely due to chemical reactions resulting from the addition of water to the soil, rather than life. Martian soil appears to be more chemically active than terrestrial soil due to exposure to solar ultraviolet radiation. The organic chemistry experiment revealed no trace of organic material due to the sterilizing effect of ultraviolet light on the martian surface. While the possibility of life on the surface hasn\'t been ruled out, most experts consider it highly unlikely. Mars had similar surface conditions to Earth about 4 billion years ago, raising the prospect that life could have begun there at the same time as on Earth. Scientists are now focused on searching for fossilized life on Mars and whether it once supported its own life forms. Future spacecraft missions will aim to collect martian samples from sedimentary rocks at sites that may have once contained water and potentially ancient life, and the most effective searches for martian life will be conducted in laboratories on Earth. Divergent Planetary Evolution ----------------------------- The planets Venus, Mars, and Earth make up a diverse trio of worlds. Despite orbiting in a similar inner zone around the Sun and beginning with similar chemical compositions of silicates and metals, their paths of development have differed. Consequently, Venus became hot and arid, Mars became cold and arid, and only Earth acquired a hospitable climate. We have examined the runaway greenhouse effect on Venus and the runaway refrigerator effect on Mars, yet we lack a complete understanding of what initiated these distinct evolutionary paths for the two planets. Did Earth ever face a similar fate? Or could it potentially veer onto one of these paths, possibly due to atmospheric strain caused by human pollutants? Exploring Venus and Mars serves the purpose of gaining insight into these inquiries. Some individuals have proposed that a better understanding of the evolution of Mars and Venus could lead to the possibility of reversing their evolution and reinstating more Earth-like environments. While it appears improbable that humans could transform either Mars or Venus into Earth replicas, contemplating such scenarios is a valuable aspect of our broader pursuit to comprehend the delicate environmental equilibrium that sets our planet apart from its neighboring worlds. In \"Cosmic Samples and the Origin of the Solar System,\" we revisit the comparative analysis of the terrestrial planets and their divergent evolutionary chronicles.