Lecture 2: Microbial Genetics PDF
Document Details
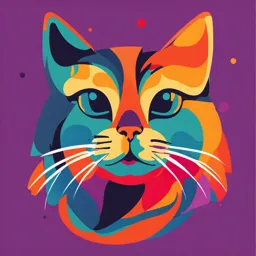
Uploaded by ViewableQuasar2637
Damietta University
Dr. Manal Mohamed Zaater
Tags
Related
- VL12 Biotechnology and genetic engineering PDF
- U.S. Army Medical Center of Excellence Interservice Physician Assistant Program Block 2-1 Microbial Genetics PDF
- BIOL2010 Week 5 Microbial Genetics PDF
- Lecture 1: Introduction to Agricultural Biotechnology PDF
- Microbial Biotechnology Lecture Notes PDF
- Microbial Genetics Lecture 4 (BI 302) PDF
Summary
This lecture covers microbial genetics, its role in genetic research, biotechnology, and applications in the study of human biology. It discusses essential concepts like rapid growth and reproduction of microorganisms, model organisms, genetic manipulation tools, gene transfer mechanisms, and biotechnological applications.
Full Transcript
وراثة الطفرات والكائنات الدقيقة Dr. Manal Mohamed Zaater Lecturer of Genetics Fac. of Agri., Damietta University Microorganisms play a crucial role in genetic studies for several reasons, primarily due to their unique characteristics and the advantages they offer in research and biotechnolog...
وراثة الطفرات والكائنات الدقيقة Dr. Manal Mohamed Zaater Lecturer of Genetics Fac. of Agri., Damietta University Microorganisms play a crucial role in genetic studies for several reasons, primarily due to their unique characteristics and the advantages they offer in research and biotechnology. The reasons for using microorganisms in genetic studies: Rapid Growth and Reproduction Microorganisms, especially bacteria, have rapid growth rates and short generation times, allowing scientists to observe genetic changes and evolution over relatively short periods. This rapid reproduction facilitates the study of genetic variations and evolutionary processes more efficiently than larger organisms https://en.wikipedia.org/wiki/Microbial_genetics Model Organisms Bacteria serve as excellent model organisms in genetic research. Their relatively simple genomes and well-understood genetics make them ideal for studying fundamental biological processes. For instance, Escherichia coli has been extensively used to elucidate basic genetic mechanisms, leading to significant discoveries in molecular biology, such as the one gene-one enzyme hypothesis https://en.wikipedia.org/wiki/Microbial_genetics https://en.wikipedia.org/wiki/One_gene%E2%80%93one_enzyme_hypothesis Genetic Manipulation Tools Microorganisms are essential sources of genetic tools that have revolutionized genetic engineering. Techniques such as CRISPR-Cas9, which originated from bacterial immune systems, allow precise editing of genomes. Additionally, enzymes like DNA polymerases from thermophilic bacteria are crucial for techniques such as PCR (Polymerase Chain Reaction), enabling the amplification of DNA for various applications https://www.ncbi.nlm.nih.gov/pmc/articles/PMC10982607/ https://en.wikipedia.org/wiki/Microbial_genetics https://bio.libretexts.org/Bookshelves/Microbiology/Microbiology_(OpenStax)/12:_Modern_Applications_of_Microbial_Genetics Gene Transfer Microbes can exchange genetic material through processes like conjugation, transduction, and transformation, which enhance genetic diversity. This ability allows researchers to study gene transfer mechanisms and their implications for evolution, antibiotic resistance, and pathogenicity. Understanding these processes is vital for addressing public health challenges, such as the spread of antibiotic resistance. https://www.ncbi.nlm.nih.gov/books/NBK7908/ https://academic.oup.com/femsre/article/24/1/1/525624?login=false Biotechnological Applications Microbial genetics has significant implications in biotechnology. Microorganisms are utilized to produce a variety of products, including antibiotics, vaccines, and enzymes. For example, insulin production has been industrialized using genetically modified bacteria. The manipulation of microbial genetics not only aids in the production of therapeutic agents but also contributes to advancements in sustainable practices, such as biodegradable plastics https://www.ncbi.nlm.nih.gov/pmc/articles/PMC10982607/ https://bio.libretexts.org/Bookshelves/Microbiology/Microbiology_(OpenStax)/12:_Modern_Applications_of_Microbial_Genetics Human Biology Studies Studying microorganisms can provide insights into human health and disease mechanisms. Many microbial pathways are analogous to those in humans, making them valuable for understanding drug metabolism and disease processes. Research into the human microbiome has revealed its critical role in health and disease, further emphasizing the importance of microbial genetics. https://www.ncbi.nlm.nih.gov/pmc/articles/PMC10982607/ https://en.wikipedia.org/wiki/Microbial_genetics DNA: STRUCTURE, REPLICATION,AND ORGANIZATION Knowledge of Nucleic Acid Chemistry Is Essential to the Understanding of DNA Structure Having established the critical importance of DNA and RNA in genetic processes, we will now take a brief look at the chemical structures of these molecules. As we shall see, the structural components of DNA and RNA are very similar. This chemical similarity is important in the coordinated functions played by these molecules during gene expression. Like the other major groups of organic biomolecules (proteins, carbohydrates, and lipids), nucleic acid chemistry is based on a variety of similar building blocks that are polymerized into chains of varying lengths. Nucleotides: Building Blocks of Nucleic Acids DNA is a nucleic acid, and nucleotides are the building blocks of all nucleic acid molecules. Sometimes called mononucleotides, these structural units consist of three essential components: a nitrogenous base, a pentose sugar (a 5-carbon sugar), and a phosphate group. There are two kinds of nitrogenous bases: the nine-member double-ring purines and the six-member single-ring pyrimidines. Two types of purines and three types of pyrimidines are commonly found in nucleic acids. The two purines are guanine and adenine, abbreviated G and A. The three pyrimidines are cytosine , thymine, and uracil, abbreviated C, T, and U, respectively. The chemical structures of A, G, C, T, and U are shown in the Next Figure. Both DNA and RNA contain A, C, and G, but only DNA contains the base T and only RNA contains the base U. Each nitrogen or carbon atom of the ring structures of purines and pyrimidines is designated by an unprimed number. The pentose sugars found in nucleic acids give them their names. Ribonucleic acids (RNA) contain ribose, while deoxyribonucleic acids (DNA) contain deoxyribose. If a molecule is composed of a purine or pyrimidine base and a ribose or deoxyribose sugar, the chemical unit is called a nucleoside. If a phosphate group is added to the nucleoside, the molecule is now called a nucleotide. Nucleosides and nucleotides are named according to the specific nitrogenous base (A, T, G, C, or U) that is part of the molecule. Nucleoside Diphosphates and Triphosphates Nucleotides are also described by the term nucleoside monophosphate (NMP). The addition of one or two phosphate groups results in nucleoside diphosphates (NDPs) and triphosphates (NTPs), In addition, adenosine triphosphate (ATP) and guanosine triphosphate (GTP) are important in cell bioenergetics because of the large amount of energy involved in adding or removing the terminal phosphate group. The hydrolysis of ATP or GTP to ADP or GDP and inorganic phosphate (Pi) is accompanied by the release of a large amount of energy in the cell. When these chemical conversions are coupled to other reactions, the energy produced is used to drive the reactions. As a result, both ATP and GTP are involved in many cellular activities, including numerous genetic events. DNA Replication in Prokaryotes DNA replication has been extremely well studied in prokaryotes primarily because of the small size of the genome and the mutants that are available. E. coli has 4.6 million base pairs in a single circular chromosome and all of it gets replicated in approximately 42 minutes, starting from a single origin of replication and proceeding around the circle in both directions. This means that approximately 1000 nucleotides are added per second. The process is quite rapid and occurs without many mistakes. DNA replication employs a large number of proteins and enzymes, each of which plays a critical role during the process. One of the key players is the enzyme DNA polymerase, also known as DNA pol, which adds nucleotides one by one to the growing DNA chain that are complementary to the template strand. The addition of nucleotides requires energy; this energy is obtained from the nucleotides that have three phosphates attached to them, similar to ATP which has three phosphate groups attached. When the bond between the phosphates is broken, the energy released is used to form the phosphodiester bond between the incoming nucleotide and the growing chain In prokaryotes, three main types of polymerases are known: DNA pol I, DNA pol II, and DNA pol III. It is now known that DNA pol III is the enzyme required for DNA synthesis; DNA pol I and DNA pol II are primarily required for repair. How does the replication machinery know where to begin? It turns out that there are specific nucleotide sequences called origins of replication where replication begins. In E. coli, which has a single origin of replication on its one chromosome (as do most prokaryotes), it is approximately 245 base pairs long and is rich in AT sequences. The origin of replication is recognized by certain proteins that bind to this site. An enzyme called helicase unwinds the DNA by breaking the hydrogen bonds between the nitrogenous base pairs. ATP hydrolysis is required for this process. As the DNA opens up, Y-shaped structures called replication forks are formed. Two replication forks are formed at the origin of replication, and these get extended bi- directionally as replication proceeds. Single-strand binding proteins coat the single strands of DNA near the replication fork to prevent the single-stranded DNA from winding back into a double helix. DNA polymerase is able to add nucleotides only in the 5' to 3' direction (a new DNA strand can be only extended in this direction). It also requires a free 3'-OH group to which it can add nucleotides by forming a phosphodiester bond between the 3'-OH end and the 5' phosphate of the next nucleotide. This essentially means that it cannot add nucleotides if a free 3'-OH group is not available. Then how does it add the first nucleotide? The problem is solved with the help of a primer that provides the free 3'-OH end. Another enzyme, RNA primase, synthesizes an RNA primer that is about five to ten nucleotides long and complementary to the DNA. Because this sequence primes the DNA synthesis, it is appropriately called the primer. DNA polymerase can now extend this RNA primer, adding nucleotides one by one that are complementary to the template strand What is Topoisomerase? Topoisomerase is an enzyme that allows DNA to change its topology, which is its shape. In bacteria, DNA exists in a single circular chromosome. However, in multicellular life it is bundled in X-shaped chromosomes. When double-stranded DNA is packaged in this way it must be coiled onto itself, creating what is called a supercoil. Supercoiled DNA resembles the cord on a landline telephone. When tension is created in one area of the coil, other areas of the coil become more or less relaxed in response. The Function of Topoisomerase The function of topoisomerase is to unwind the chromosomes and DNA double-helix by creating small, reversible cuts in the DNA. Topoisomerase enzymes can be thought of as tiny surgeons who wield molecular scissors. They cut at places that hold too much tension and then repair the cut during the process of DNA replication. DNA replication steps 1.DNA unwinds at the origin of replication. 2.Helicase opens up the DNA-forming replication forks; these are extended bidirectionally. 3.Single-strand binding proteins coat the DNA around the replication fork to prevent rewinding of the DNA. 4.Topoisomerase binds at the region ahead of the replication fork to prevent supercoiling. 5.Primase synthesizes RNA primers complementary to the DNA strand. 6.DNA polymerase starts adding nucleotides to the 3'-OH end of the primer. 7.Elongation of both the lagging and the leading strand continues. 8.RNA primers are removed by exonuclease activity. 9.Gaps are filled by DNA pol by adding dNTPs. 10.The gap between the two DNA fragments is sealed by DNA ligase, which helps in the formation of phosphodiester bonds. Enzyme/protein Specific Function DNA pol I Exonuclease activity removes RNA primer and replaces with newly synthesized DNA DNA pol II Repair function DNA pol III Main enzyme that adds nucleotides in the 5'-3' direction Helicase Opens the DNA helix by breaking hydrogen bonds between the nitrogenous bases Ligase Seals the gaps between the Okazaki fragments to create one continuous DNA strand Primase Synthesizes RNA primers needed to start replication Sliding Clamp Helps to hold the DNA polymerase in place when nucleotides are being added Topoisomerase Helps relieve the stress on DNA when unwinding by causing breaks and then resealing the DNA Single-strand binding Binds to single-stranded DNA to avoid DNA rewinding back. proteins (SSB) The sliding clamp is a ring-shaped protein that encircles duplex DNA, binds to the DNA polymerase and tethers it to the DNA template, preventing its dissociation and providing high processivity.