DNA & RNA Structure Lecture Notes PDF
Document Details
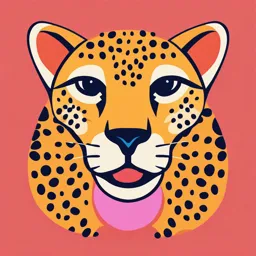
Uploaded by sonia
Cihan University
2024
Tags
Summary
These lecture notes cover the structure and function of DNA and RNA. They detail primary, secondary, and tertiary structures, along with the various types of RNA and their roles.
Full Transcript
DNA &RNA STRUCTURE 2nd Lecture 14/9/2024 Overview: Define nucleic acids and its types. Clarify the structure of DNA and RNA, primary structure, secondary and tertiary structure. Explain the types of RNA in cells with their functions. Nucleic acids Nucleic acids are l...
DNA &RNA STRUCTURE 2nd Lecture 14/9/2024 Overview: Define nucleic acids and its types. Clarify the structure of DNA and RNA, primary structure, secondary and tertiary structure. Explain the types of RNA in cells with their functions. Nucleic acids Nucleic acids are long-chain polymeric molecules, the monomer (the repeating unit) is known as nucleotides and hence sometimes nucleic acids are referred to as polynucleotides. There are two types of nucleic acids: 1. Deoxyribonucleic acid (DNA) 2. Ribonucleic acid (RNA) DNA Deoxyribonucleic acid DNA - a polymer of deoxyribonucleotides. Usually, double stranded. And have double-helix structure. Found in chromosomes, mitochondria and chloroplasts. It acts as the genetic material in most of the organisms. Carries the genetic information DNA Structure DNA structure is often divided into four different levels primary, secondary, tertiary and quaternary The primary DNA structure mainly refers to the linear nucleotide sequences that are held together by strong phosphodiester bonds. Nucleotides has three main components: 1. Deoxyribose (a pentose sugar) 2. Base (there are four different types) 3. Phosphate Primary structure 1. Five-carbon sugars Nucleotide subunits of DNA contain the sugar deoxyribose. The rings are not only made of carbon The sugars differ only in the presence or absence (“deoxy”) of an oxygen in the 2′ position 2. Bases They are nitrogen-containing molecules having the chemical properties of a base. Two groups of the bases: A) purines ⎯ Have a double carbon–nitrogen ring structure ⎯ Including Adenine (A) and Guanine (G) B) pyrimidines ⎯ Have a single ring structure ⎯ Including: thymine (T), cytosine (C), and uracil (U) ⎯ Thymine is found in DNA only, while uridine is specific for RNA. Chargaff’s Rule The number of A residues in all DNA samples equal to the number of T residues; i.e. [A] = [T], the number of G residues equals that of C, [G] = [C]. Finally, the amount of the purine bases equals that of the pyrimidine bases: [A] + [G] = [T] + [C]. The G + C content can vary from 22 to 73%, depending on the organism. 3. The phosphate Functional Group The phosphate functional group (PO4) gives DNA and RNA the property of an acid. The linking bonds that are formed from phosphates are esters that have the additional property of being stable. The negatively charged phosphates are extremely insoluble in lipids, which ensures the retention of nucleic acids within the cell or nuclear membrane. Nucleosides and nucleotides A DNA or RNA chain is formed in a series of three steps: 1. In the first reaction, each base is chemically linked to one molecule of sugar at the 1′-carbon of the sugar, forming a compound called a nucleoside. 2. In the second reaction, a phosphate group is also attached to the 5′-carbon of the same sugar, the nucleoside becomes a nucleotide. 3. Finally, nucleotides are joined (polymerized) by condensation reactions to form a chain by phosphodiester bond. ⎯ Base + sugar = nucleoside Example: Adenine + ribose = Adenosine ⎯ Base + sugar + phosphate(s) =nucleotide Example: Deoxyadenosine monophosphate (dAMP) Significance of 5′ and 3′ The two ends are designated by the symbols 5′ and 3′, with different chemical properties. The symbol 5′ refers to the carbon 5 in the sugar that a phosphate (PO4) functional group is attached. The symbol 3′ refers to the carbon 3 in the sugar ring to which a hydroxyl (OH) functional group is attached. By convention, a DNA sequence is written with the 5′ end to the left, and the 3′ end to the right. This is also the direction of synthesis. Secondary structure of DNA Hydrogen bonds form between the bases: Thermodynamically stable hydrogen bonds form between the nitrogenous bases on opposite strands of the DNA chains. Hydrogen bonds are weak bonds. The hydrogen bonds provide one type of force holding the strands together and give structural stability. It occurs in a way that adenine (A) pairs with thymine (T) by two hydrogen bonds, and guanine (G) pairs with cytosine (C) by three hydrogen bonds. Secondary structure of DNA Base stacking: Stacking is the hydrophobic interaction between the flat surfaces of adjacent bases. It makes a significant contribution to the stability of a nucleic acid in solution. Helps to minimize contact with water. Nitrogenous bases are nonpolar and thus hydrophobic (“water hating”) Also involves combinations of Van der waals force ( weak electrostatic force) between the bases. Secondary structure of DNA Major and minor grooves: The two bonds that attach a base pair to its deoxyribose sugar rings are not directly opposite. Therefore, the sugar–phosphate backbone is not equally spaced. This results in what are called the major and minor grooves of DNA. The major groove has a significant role in sequence-specific DNA–protein interactions. The minor groove of DNA is less informative. most transcription factors (proteins involved in regulating gene expression) bind DNA in the major groove. Alternative double-helical structures of DNA Studies have revealed the basic structure of three fundamental types of double helix: B-, A-, and Z-DNA. 1. B-DNA (Watson–Crick DNA) B-DNA is a right-handed helix. The bases are stacked almost exactly perpendicular to the main axis with 10.5 bases per turn. The major groove is wide and of moderate depth, while the minor groove is of moderate depth but is much narrower. B-DNA occurs under conditions of high humidity (95%) and relatively low salt. The predominant form in vivo is B-DNA. 2. A-DNA If the water content is decreased and the salt concentration increased during crystal formation, the A form of DNA (A-DNA) will occur. It is right-handed helix and there are 11 bases per turn. The major groove of A-DNA is deep and narrow, while the minor groove is shallow. 3. Z DNA It is left -handed helix and has 12 base pairs per turn. The minor groove is very deep and narrow. In contrast, the major groove is shallow to the point of being virtually nonexistent. ZDNA forms under conditions of high salt, presence of alcohol, and when methyl groups are added to the cytosines. Unusual DNA secondary structures 1. Slipped structures Slipped structures have been postulated to occur at tandem repeats. A tandem repeat in DNA is two or more adjacent, approximate copies of a pattern of nucleotides, arranged in a head to tail fashion. For example, the sequence 5′-TACGTACGTACGTACG-3′ contains four tandem repeats of “TACG”. Unusual DNA secondary structures 2. Cruciform structures The formation of short bubbles of unpaired single-stranded DNA. Occur in the presence of inverted repeats that are base sequences of identical composition on the complementary strands. They read exactly the same from 5′ → 3′ on each strand. Unusual DNA secondary structures 3. Triple helix DNA In a triple helix, a third strand of DNA joins the first two to form triplex DNA. Triple helix DNA occurs at purine–pyrimidine stretches in DNA and is favored by sequences containing a mirror repeat symmetry. Supercoiled DNA Many naturally occurring DNA molecules are circular, with no free 5′ or 3′ end. The 5′ end of one strand can only join its own 3′ end to covalently close a circle. This DNA can then become supercoiled (under torsional stress). Supercoils are a twisted, three-dimensional structure which is more favorable energetically. Supercoiling occurs both in vivo and in vitro. The supercoiled state is inherently less stable than relaxed DNA Topoisomerases relax supercoiled DNA Topoisomerases are highly conserved enzymes that convert (isomerize) one topoisomer of DNA to another. DNA topoisomerases fall into two major categories, type I and type II. 1. Type I topoisomerases are proficient at relaxing supercoiled DNA. They do not require the energy of ATP. They act by forming a transient single-stranded break in the DNA, while winding the broken ends, pass the other strand through the break. 2. Type II topoisomerases are usually ATP-dependent. They form transient double-stranded breaks in the double helix and pass another double helix through the temporary gap or DNA-linked “protein gate.” RNA structure RNA is typically single stranded. Is made of ribonucleotides that are linked by phosphodiester bonds. A ribonucleotide in the RNA chain contains ribose (the pentose sugar), one of the four nitrogenous bases (A, U, G, and C), and a phosphate group. The subtle structural difference between the sugars gives DNA added stability, making DNA more suitable for storage of genetic information, whereas the relative instability of RNA makes it more suitable for its more short-term functions. Secondary and Tertiary RNA structure: Double helical characteristics of RNA Stem and loop structure Internal loops Bulges Junctions Types of RNA In both prokaryotes and eukaryotes, there are three main types of RNA: 1. Messenger RNA (mRNA) mRNA accounts for just 5% of the total RNA in the cell. is the most heterogeneous types of RNA in terms of both base sequence and size. It carries complimentary genetic code copied in the form of triplets of nucleotides called codons. Each codon specifies a particular amino acid. In eukaryotes, the 5’ end of mRNA is capped with a guanosine triphosphate nucleotide. The 3’ end of an mRNA has a poly-A tail or multiple adenylate residues added to it, which prevents enzymatic degradation of mRNA. Both the 5’ and 3’ end of an mRNA imparts stability to the mRNA. 2. Ribosomal RNA (rRNA) rRNAs found in the ribosomes and account for 80% of the total RNA present in the cell. Ribosomes are composed of a large subunit called the 50S and a small subunit called the 30S, each of which is made up of its own specific rRNA molecules. They interact with tRNAs and other molecules that are crucial to protein synthesis. The structure and function of ribosomes is largely similar across all species. 3. Transfer RNA (tRNA) tRNA is the smallest of the 3 types of RNA, possessing around 75-95 nucleotides. tRNAs are an essential component of translation, where their main function is the transfer of amino acids during protein synthesis. Each of the 20 amino acids has a specific tRNA that binds with it and transfers it to the growing polypeptide chain. Other types of RNA Beyond the primary role of RNA in protein synthesis, several varieties of RNA exist that are involved in post-transcriptional modification, DNA replication, and gene regulation. Some forms of RNA are only found in particular forms of life, such as in eukaryotes or bacteria. Such as snRNA, regulatory RNAs and mtRNA. 1. Small Nuclear RNA (snRNA) They are very short, with an average length of only 150 nucleotides. snRNA is involved in the processing of pre-messenger RNA (pre-mRNA) into mature mRNA. 2. Regulatory RNAs A number of types of RNA are involved in regulation of gene expression, including micro RNA (miRNA), small interfering RNA (siRNA) and antisense RNA (aRNA). miRNA (21-22 nt) is found in eukaryotes, miRNA can break down mRNA with the aid of enzymes. This can block the mRNA from being translated or accelerate its degradation. siRNA (20-25 nt) are often produced by breakdown of viral RNA. They act similarly to miRNA. 3. Transfer-messenger RNA (tmRNA) Found in many bacteria and plastids. tmRNA tag the proteins encoded by mRNAs that lack stop codons for degradation, And prevents the ribosome from stalling due to the missing stop