Practice Sheet: Land Sparing vs Land Sharing PDF
Document Details
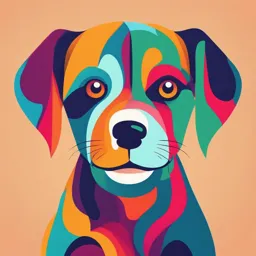
Uploaded by BeneficiaryLitotes3821
University of Bergen
Tags
Related
Summary
This practice sheet examines the concept of land sparing versus land sharing, analyzing the trade-offs between biodiversity conservation and food production. It explores different perspectives on food, including food production, food security, and food sovereignty. The practice sheet also discusses the concept of carrying capacity and the limitations of a purely biophysical approach. It encourages a more nuanced understanding of the complexities surrounding food and biodiversity.
Full Transcript
Practice sheet 1 and 2. Demography and Carrying capacity. Global scale texts here: “Land Sparing Versus Land Sharing: Moving Forward”- Joern Fischer ✅ viktige quotes: The framework on land spari...
Practice sheet 1 and 2. Demography and Carrying capacity. Global scale texts here: “Land Sparing Versus Land Sharing: Moving Forward”- Joern Fischer ✅ viktige quotes: The framework on land sparing versus land sharing is essentially an economic one because it is interested in the efficient allocation of a scarce resource, namely land Food production: technology-focused. Much literature on the written history of hunger has come at it from the perspective of “too many people, too little food,” and thus has looked at remedying famine and chronic hunger through increasing food production. Food security: states-focused. Food security can be de- fined as physical and economic access by all people in a society at all times to enough culturally and nutrition- ally appropriate food for a healthy and active lifestyle (e.g., Food and Agriculture Organization of the United Nations [FAO] 1996). This multidimensional definition was developed in response to research showing that “starvation is the characteristic of some people not hav- ing enough food to eat. Food sovereignty: people-focused. Food sovereignty de- fines “the rights of local peoples to determine their own agricultural and food policy, organize production and consumption to meet local needs, and secure access to land, water, and seed” Practice sheet 1 (Wittman 2010). It is viewed by proponents as a corrective to the inadequacies of “food security,” which “avoided discussing the social control of the food system Practice sheet 2 Indeed, many sustainability problems that in- volve both social and environmental considerations are “wicked” problems—in such problems there is no “right” solution, but “only trade-offs that appear more or less favorable depending on your perspective” (Game et al. 2014) No matter how biodiversity is defined, by focusing on one part of biodiversity, the consequences of any given land use strategy on other parts of biodiversity might be glossed over or underestimated. Management might favor, for example, species that are rare or those that provide particular ecosystem functions. While many rare species cannot survive in farmland, many species that provide important ecosystem functions can. Some of these species, in turn, provide ecosystem services that positively influence yields (Bommarco et al. 2012). In a nutshell, the framework fits into discourses on food production and land scarcity, but says nothing about food security or food sovereignty. It can help to identify trade-offs, but cannot tell us which of these trade-offs are socially desired. Its answers on biodiversity are only as good as the ways in which biodiversity is defined and measured. The framework distinguishes between two hypothetical conservation mechanisms (sparing versus sharing) but is not well- designed to Practice sheet 3 address scale issues or globalization ef- fects. Finally, the viable coexistence of humans and nature hinges on many additional variables that defy quantification. The authors identify several points of contention within this framework, one of which is the focus on food production. Much of the research on land sparing versus land sharing focuses on minimising harm to biodiversity while producing food. The paper highlights that the concept of 'food' is studied by scholars from diverse disciplines, leading to different perspectives on the land sparing versus land sharing framework. These communities include those focused on ecology, economics, and land use science, as well as those working on poverty reduction and sustainability. The authors note that the concept of “food” is often approached from three perspectives: Food production: This approach concentrates on increasing food production through technological advancements, with a historical emphasis on addressing hunger by increasing food supply. Food security: This perspective considers the availability, access, and utilisation of food, acknowledging that production alone does not guarantee food security for all. Food sovereignty: This view emphasizes local control and self-determination over food systems, promoting local production and consumption, and challenging the dominance of global market forces in shaping food systems. The paper also discusses the concept of a production possibility frontier, which shows the most efficient allocation of land for producing two goods – biodiversity and agricultural production. Trade-off analysis can help to distinguish between efficient and inefficient land allocations. However, the authors argue that trade-off analysis often oversimplifies the issue, neglecting factors like scale effects and teleconnections. For example, implementing a land sparing system in one region might result in agricultural activities being shifted to a different region through global trade dynamics, a phenomenon known as leakage. These spatial interdependencies are often overlooked in land sparing versus land sharing analyses. The authors conclude by making several recommendations for moving forward in the debate on land sparing versus land sharing: 1. Critically evaluate the use of partial trade-off analyses, acknowledging their limitations. 2. Recognise that choosing how to quantify biodiversity involves subjective decisions. 3. Address the scale effects and teleconnections that impact land-use decisions. Practice sheet 4 4. Develop alternative, more holistic frameworks to analyse the relationship between food and biodiversity. One promising approach is to view agricultural landscapes as social-ecological systems, considering the interplay of social and ecological factors. The authors encourage a more nuanced understanding of the complexities surrounding food and biodiversity, moving beyond the simplistic binary of land sparing versus land sharing. “Humanity's Fundamental Environmental Limits” Binder ✅ This paper by Binder et al. explores the concept of Earth's carrying capacity for humans, focusing on biophysical limits. The authors develop a model to estimate carrying capacity under various technological scenarios. While not explicitly discussing land sparing versus land sharing, the paper provides insights relevant to the debate: The authors emphasize that technological advancements can significantly impact carrying capacity estimates. For instance, improvements in agricultural technology can increase food production per unit of land, potentially allowing for higher population densities. The paper highlights the importance of considering multiple resource constraints simultaneously. Their model incorporates factors like sunlight, water, and essential nutrients to determine how many people Earth can sustainably support under different scenarios. They acknowledge the limitations of a purely biophysical approach, suggesting that social and cultural factors play a crucial role in defining carrying capacity in practice. The authors advocate for linking biophysical models with economic models to assess trade-offs between population, consumption, and technology. The findings of this study, while not directly addressing the specifics of land sparing or land sharing, reinforce the need to consider technological possibilities and resource limitations in conservation and land management decisions. “Does agroforestry conserve trees?” Lila Nath Sharma, Ole Reidar Vetaas ✅ viktige sitat We compared different measures of diversity; number of tree species in the sample plots (alpha diversity), total number of species in each land-use type (gamma diversity), and the change in species composition along an elevational gradient (beta diversity), which follows the classical definition of Whittaker (1972) [details in the Practice sheet 5 data analysis section]. We consider that trees in farmland are a mixture of natural forest species and introduced fodder and fruit species. The balance of the trade-offs between forest harvesting and tree retention/plantations in farmlands can contribute to conserving forest as well as trees in an agroforestry landscape. Integrating community forestry under the land-sharing strategy, therefore, seems a promising option to manage biodiversity in an agricultural landscape. However, the concept of biodiversity should go beyond ‘greenness’ or ‘forest cover’ to include different aspects of species diversity. Conservation planning must acknowledge the role of traditional as well as new agroforestry practices in species maintenance and con- servation in addition to anthropocentric provisioning ecosystem services (Manning et al. 2006; Tscharntke et al. 2011). Multipurpose trees on farmland should be promoted because they provide supplementary habitats and dispersal corridors for other species (Bhagwat et al. 2008; Harvey and Villalobos 2007; Schuepp et al. 2012; Tadesse et al. 2014) and also play a role in soil conservation through erosion control and slope stability (Young 1989). This study by Sharma and Vetaas examines tree species diversity in farmland and forest ecosystems in the central hills of Nepal, a region where agroforestry is a common practice. Agroforestry is a land sharing strategy that integrates trees into agricultural landscapes, providing a variety of ecosystem services while also potentially supporting biodiversity. The authors sought to determine if agroforestry contributes to tree species conservation in this region. They compared tree diversity in farmland to that of adjacent, sustainably managed forests. They tested three specific hypotheses: 1. Alpha diversity is higher in farmland than in forest. 2. Gamma diversity is lower in farmland than in forest, potentially due to the planting of the same tree species across farmlands, leading to homogenisation. 3. Tree species composition differs between farmland and forest due to the inclusion of both local forest trees and introduced species on farmlands. Their findings revealed that: Farmlands had consistently higher alpha diversity compared to forests. Practice sheet 6 While there were many shared species, farmland and forest ecosystems differed in composition due to varying species abundances and unique species present in each land use type. Farmland demonstrated higher gamma diversity compared to the forest, contradicting their initial hypothesis. The study highlights that agroforestry plays a significant role in maintaining tree species diversity in agricultural landscapes. The authors argue that these findings challenge the common assumption that forests generally harbour higher biodiversity than open landscapes. They suggest that agroforestry may even enhance biodiversity in certain cases. The authors emphasize the need to consider agroforestry in conservation planning. They note that existing national biodiversity policies in Nepal often overlook the role of trees on farmlands as a crucial component of agrobiodiversity. They advocate for greater recognition and support of agroforestry practices to achieve both local and regional biodiversity conservation objectives. “Population Growth and Earth's Human Carrying Capacity” - Joel E. Cohen ✅ This article by Cohen discusses the concept of human carrying capacity and its implications for sustainability. The author surveys various methods for estimating carrying capacity and examines the limitations of each approach. The paper does not explicitly address land sparing and land sharing. However, it provides a broader context for understanding the challenges of balancing human population growth with resource availability and environmental conservation. Key points relevant to the debate include: Cohen highlights the inherent uncertainty in estimating carrying capacity due to constantly changing technological, social, and environmental factors. This dynamic nature makes it difficult to define a fixed carrying capacity for humans. The article emphasizes the importance of considering multiple, interdependent factors that can constrain human population growth. These can include food, water, energy, and other resources, as well as environmental impacts like pollution and climate change. Cohen critiques deterministic and static approaches to estimating carrying capacity, advocating for models that incorporate stochastic variability and dynamic interactions between human and environmental systems. Practice sheet 7 These insights underscore the need for a nuanced and adaptive approach to managing land for both human needs and biodiversity conservation, acknowledging the uncertainties and complexities involved. Agroforestry: a refuge for tropical biodiversity? ✅ vikitge sitater: Therefore, any approach that aims to mitigate tropical deforestation and protect biodiversity should address the livelihoods and needs of local communities First, we highlight the changing concept of ‘pristine nature’ by arguing that people have played a key role in shaping many of the so-called pristine forests of today and empha- sise that future approaches to conservation need to con- sider agroforestry. Second, we recognise the so-called matrix effect on species diversity in landscape mosaics with native tree cover, and suggest that agroforestry sys- tems can provide corridors that connect distant reserves Although there is no doubt that protected areas do contribute hugely to preserving large parts of the biodiversity on Earth, such areas often fail to cover the entire diversity of ecological habitats and species and, as crucially, strict protection of such areas is often resented by local people. In a recent debate over the future of tropical forest species, it has been suggested that although some forest specialists need ‘intact’ forest habitat, the bulk of tropical species will be forced to persist in degraded and secondary habitats outside forest reserves in the future (see Ref. and references therein). There- fore, the need for biodiversity conservation in human- dominated landscapes in the tropics is greater today than ever First, many agroforestry systems are shown to be important for the protection of species and habitats outside formally protected areas. For example, Williams- Guillen and colleagues found that shade coffee plantations in Nicaragua can serve as alternative wildlife habitats and as corridors between forest fragments for mantled howling monkeys Alouatta palliata Second, agroforestry systems maintain heterogeneity at the habitat and landscape scales. For example, Parikesit and colleagues identified 12 different plant assem- blages in Kebon Tatangkalan agroforests of the Upper Citarum Watershed, West Java, Indonesia. These agrofor- ests are maintained by small households spread across the watershed and contribute to the highly heterogeneous species assemblages in the regional flora. Third, trees in agroforestry landscapes reduce pressure on formally protected forest reserves. Murniati and col- leagues studied the availability of forest resources Practice sheet 8 in mixed gardens and village forests in the northern part of the Kerinci Seblat National Park, Sumatra, Indonesia. They found that households that owned mixed gardens depended much less on national park resources than households which cultivated wetland rice fields alone. Although agroforestry systems cannot stand alone as conservation areas, they can buffer existing reserves and provide corridors for persist- ence and movement of species across landscapes (e.g. Ref. ). Such systems offer a useful means for combating species loss as a result of tropical forest fragmentation 3 The literature reviewed suggests that agroforests with less intensive management and high canopy cover have high species richness and are more similar to neighbouring forest reserves than intensively managed agroforests with open canopies. Effective management of agroforestry sys- tems, therefore, entails several aspects (see Box 1). First, we suggest an integrated approach to reserve networks that includes agroforestry systems as an important con- servation tool. Second, we recommend that farmers who maintain agroforests might, in particular circumstances, be offered compensation in the form of various incentives for biodiversity-friendly farming practices. Third, we advo- cate that awareness and education programmes are crucial to make farmers self-sufficient and to harness the potential of agroforests for biodiversity conservation This opinion piece by Bhagwat et al. explores the potential of agroforestry for biodiversity conservation in tropical regions facing deforestation and habitat loss. The authors challenge the traditional conservation focus on ‘pristine nature’ within protected areas. They argue that many seemingly pristine forests have a history of human influence, and that future conservation strategies must consider the importance of human- modified landscapes. The authors reviewed numerous studies comparing species richness and composition in agroforestry systems to neighbouring forest reserves. They found that: Agroforestry systems can support a significant proportion of the biodiversity found in forests, with average richness exceeding 60% of forest values. The similarity in species composition between agroforestry and forests varies depending on the taxa and management intensity. For instance, mobile taxa like bats, Practice sheet 9 mammals, and birds exhibited higher similarity values compared to plants. Agroforestry systems tend to have higher species richness and greater similarity to forests when: (1) conversion from forest to agroforestry occurred relatively recently; (2) management is less intensive; and (3) canopy cover of native trees is high. The authors highlight three key reasons why agroforestry systems are valuable for biodiversity conservation: 1. They provide habitat for species outside of protected areas. For example, shade coffee plantations can serve as habitat for various forest-dwelling species and act as corridors between forest fragments. 2.They maintain habitat heterogeneity at both the habitat and landscape scales. This is because farmers often manage their agroforestry plots differently, leading to a mosaic of different tree species and vegetation structures across the landscape. 3.Trees in agroforestry landscapes can reduce pressure on protected forest reserves. By providing resources like timber and fuelwood, agroforestry can alleviate the need for communities to extract these resources from protected forests. The paper discusses the concept of ‘countryside biogeography’, which emphasizes the importance of considering the surrounding landscape matrix in conservation planning.29 The authors argue that agroforestry systems can play a crucial role in connecting protected areas and facilitating species movement across fragmented landscapes. They conclude that while agroforestry systems cannot replace protected areas, they can serve as valuable complements, offering a means to conserve biodiversity within human- dominated landscapes. thr authors recommend: 1. Integrating agroforestry into conservation planning. 2. Providing incentives for farmers who engage in biodiversity-friendly agroforestry practices. 3. Raising awareness and providing education about the importance of agroforestry for conservation. Definitions and Terminologies Land Sparing: A conservation framework proposing the separation of conservation and production activities. It often involves intensifying agriculture in some areas to maximise yields, Practice sheet 10 thereby allowing other areas to be set aside for biodiversity conservation. seperate between agirculture and conservation seperation Land Sharing: A conservation framework suggesting the integration of conservation and production within the same landscape. This typically involves implementing wildlife-friendly farming practices that support biodiversity within agricultural areas. rather about integrating both, integration Land Scarcity: A concept highlighting the increasing demand for land for various purposes, including food production, housing, and infrastructure development, which can compete with biodiversity conservation goals. Food Security: A state where all people at all times have physical and economic access to sufficient, safe, and nutritious food to meet their dietary needs and preferences for an active and healthy life. Food Sovereignty: A concept emphasising people's rights to define their own agricultural and food policies, control their food systems, and prioritize local needs over global market demands. Production Possibility Frontier: A graphical representation showing all efficient combinations of producing two goods or services (e.g. biodiversity and agricultural production) given current technology. It illustrates the trade-offs between maximising one good at the expense of the other. Trade-off Analysis: A process of evaluating the costs and benefits of different land use options, considering the potential impacts on multiple goods and services, such as biodiversity, agricultural production, and ecosystem services. Agroforestry: An agricultural system that intentionally integrates trees and shrubs into crop and livestock production systems, creating a more diverse and potentially biodiverse agricultural landscape. Practice sheet 11 Alpha Diversity: A measure of species richness within a specific area or habitat. Beta Diversity: A measure of species turnover or dissimilarity in species composition between different areas or habitats. Gamma Diversity: The total species diversity within a larger region, encompassing multiple habitats or areas. Teleconnections: The interconnectedness of land use activities across different geographical locations, often through global trade networks. These connections can lead to the displacement of land use impacts and affect biodiversity in distant areas. Carrying Capacity: The maximum population size of a species that an environment can sustain indefinitely, given the available resources, environmental conditions, and the species' consumption patterns. stoichiometric stoichiometric" refers to the balance of chemical elements required for biological processes, such as the ratios of carbon, nitrogen, and phosphorus in organisms. The concept of stoichiometric constraints is discussed in the context of Earth's carrying capacity. For example, Binder et al.12 explain that even if there is sufficient energy (calories) available to support a large human population, limitations in the availability of essential nutrients, particularly phosphorus, could create a binding stoichiometric constraint on population growth. The sources also suggest that there are limitations associated with the land sparing vs. land sharing framework and that understanding scale issues and teleconnections is crucial in conservation planning. They also highlight the need for more holistic frameworks that consider social-ecological systems in addressing food security and biodiversity conservation. Additionally, they discuss the concept of "cultural carrying capacity," which takes into account not just biological needs but also social and cultural values in determining sustainable population levels. Practice sheet 12 the framwork built on the concept on land sharing and land sparing is in essencse an economic framework- how do you get enough money kinda? Beyond "Sparing" vs. "Sharing": A Deeper Look at Conservation Strategies The sources frame the debate on balancing food production and biodiversity conservation around two main strategies: land sparing and land sharing. While these terms provide a useful starting point, they also acknowledge that this dichotomy is overly simplistic and advocate for a more nuanced understanding. Land Sparing: This strategy focuses on intensifying agricultural production in specific areas, aiming to maximise yields while minimising land use. This allows for designating separate areas for conservation, such as protected areas. From an economic standpoint, land sparing aligns with the principle of efficiency: allocating land to its most productive use. By concentrating agricultural activities on land most suitable for high yields, the logic goes, we can produce more food with a smaller footprint, leaving more land for nature. However, the sources raise several economic and social critiques of this approach: Food Security vs. Food Production: Simply producing more food does not guarantee everyone has access to it. Issues of distribution, affordability, and power dynamics within food systems are crucial for achieving food security. Neglecting Food Sovereignty: This concept emphasizes local control over food systems, allowing communities to define their food needs and priorities. Land sparing, driven by global market forces, can undermine food sovereignty by prioritising export-oriented crops over local food needs. Overlooking Ecosystem Services: Focusing solely on agricultural yields disregards other valuable services provided by ecosystems, such as pollination, water regulation, and carbon sequestration. These services can be crucial for both human well-being and agricultural productivity itself. Ignoring Displacement Effects: Intensification in one area can lead to the displacement of agricultural activities to other, often more ecologically sensitive regions. This phenomenon, known as leakage, can result in deforestation and biodiversity loss elsewhere. Land Sharing: Practice sheet 13 This approach advocates for integrating conservation and production within the same landscape. This often involves promoting wildlife-friendly farming practices, such as agroforestry, organic farming, and habitat heterogeneity. Land sharing resonates with a more holistic perspective on sustainability, recognizing that agricultural landscapes can support biodiversity. However, the effectiveness of land sharing for conservation depends on several factors: Species-Specific Outcomes: While some studies show increased species richness in certain agroforestry systems compared to forests, others suggest a decline in specialist or rare species. The conservation value of land sharing varies depending on which species are prioritized and the specific management practices implemented. Management Intensity: Less intensive systems with higher canopy cover and native tree diversity tend to support more biodiversity than intensively managed monocultures. Landscape Context: The effectiveness of land sharing also depends on the surrounding landscape context. Small, isolated patches of habitat within a heavily modified matrix might not provide sufficient connectivity or resources for many species. Economic Considerations: Market Mechanisms: The success of both land sparing and land sharing depends on aligning economic incentives with conservation goals. This could involve payments for ecosystem services, certification schemes for sustainable products, or market premiums for biodiversity-friendly goods. Valuing Ecosystem Services: Incorporating the economic value of ecosystem services into decision-making processes can help justify investments in conservation and sustainable practices. This requires developing methods for quantifying and valuing these services, which can be challenging. Equity and Social Justice: Conservation strategies should address issues of equity and social justice. Who benefits from land sparing or land sharing? How do these strategies impact the livelihoods and well-being of local communities? These questions are crucial for ensuring equitable outcomes. Moving Forward: Practice sheet 14 The sources emphasize the need to move beyond the binary of "sparing" vs. "sharing" and embrace a more integrated approach to conservation and production: Context Specificity: There is no one-size-fits-all solution. Conservation strategies must be tailored to the specific ecological, social, and economic context. Multiple Scales and Connections: Conservation planning should account for global market forces, teleconnections, and the interconnectedness of landscapes. Holistic Frameworks: We need analytical approaches that consider the complex interplay of ecological, economic, and social factors within social-ecological systems. Open Dialogue and Value Articulation: Honest discussions about trade-offs, societal values, and different perspectives on the human-nature relationship are crucial for navigating these complex challenges. The sources highlight the importance of ongoing research, innovation, and collaboration to develop and implement sustainable and equitable land management practices that balance the needs of both people and nature. 3. Contested farming styles texts: “Contested farming styles” - Giller ✅ This academic paper focuses on regenerative agriculture, examining its origins, definitions, and potential to address soil and biodiversity crises. The authors note that regenerative agriculture is a relatively new term, gaining popularity in the 1980s with the work of the Rodale Institute. However, it remains poorly defined and is often conflated with other alternative agricultural approaches. The paper identifies soil health and reversal of biodiversity loss as the two major challenges regenerative agriculture seeks to address. The authors then discuss various practices associated with regenerative agriculture, such as minimizing tillage, maintaining soil cover, building soil carbon, agroforestry, relying on biological nutrient cycles, fostering plant diversity, integrating livestock, and avoiding pesticides. While acknowledging the potential benefits of these practices, the authors also emphasize the need to carefully consider their effectiveness in different contexts. They argue that a universalist approach to "fixing" agriculture may not be appropriate due to the complexity of local realities. Practice sheet 15 The authors conclude by urging research agronomists to critically evaluate the claims of regenerative agriculture and engage constructively with its proponents. They suggest focusing on five key questions: 1. What is the specific problem that Regenerative Agriculture seeks to address in a given context? 2. To what extent are these problems real, and how are they framed? 3. To what extent do the specific regenerative practices address these problems? 4. Are the purported benefits of Regenerative Agriculture demonstrably greater than those offered by other types of agriculture? 5. Are there any unintended negative consequences associated with these practices? intressante sitat Robert Rodale (1983) defined Regenerative Agriculture as ‘one that, at increasing levels of productivity, increases our land and soil biological production base. It has a high level of built-in economic and biological stability. It has minimal to no impact on the environment beyond the farm or field boundaries. It produces foodstuffs free from bio- cides. It provides for the productive contribution of increas- ingly large numbers of people during a transition to minimal reliance on non-renewable resources’ Agriculture from an agronomic perspective. By this we mean a perspective steeped in the use of plant, soil, ecological and system sciences to support the production of food, feed and fibre in a sustainable manner. Specifically, we address two questions: 1) What is the agronomic problem analysis that motivates the Regenerative Agriculture movement and what is the evidence base for this analysis? 2) What agro- nomic solutions are proposed, and how well are these sup- ported by evidence? Harwood summarizes the ‘Regenerative Agriculture Philosophy’ in 10 points (Box 1). He further states that this philosophy emphasizes: ‘1) the inter-relatedness of all parts of a farming system, including the farmer and his family; 2) the importance of the innumerable biological balances in the system; and 3) the need to maximise desired biological relationships in the system, and minimise use of materials and practices which disrupt those relationships’. 1. Agriculture should produce highly nutritional food, free from biocides, at high yields. Practice sheet 16 2.. Agriculture should increase rather than decrease soil productivity, by increasing the depth, fertility and physical characteristics of the upper soil layers. 3.. Nutrient-flow systems which fully integrate soil flora and fauna into the pattern of are more effi- cient and less destructive of the environment, and ensure better crop nutrition. Such systems accom- plish a new upward flow of nutrients in the soil profile, reducing or eliminating adverse environ- mental impact. Such a process is, by definition, a soil genesis process. 4.. Crop production should be based on biological interactions for stability, eliminating the need for synthetic biocides. 5. 5. Substances which disrupt biological structuring of the farming system (such as present-day synthetic fertilizers) should not be used. 6. 6. Regenerative agriculture requires, in its biological structuring, an intimate relationship between man- ager/participants of the system and the system itself. 7. 7. Integrated systems which are largely self-reliant in nitrogen through biological nitrogen fixation should be utilized. 8. 8. Animals in agriculture should be fed and housed in such a manner as to preclude the use of hormones and the prophylactic use of antibiotics which are then present in human food. 9. 9. Agricultural production should generate increased levels of employment. 10. 10. A Regenerative Agriculture requires national-level planning but a high degree of local and regional self-reliance to close nutrient-flow loops. To date the discussion around Regenerative Agriculture has taken little account of the wide variety of initial starting points defined by the variation in local contexts and farming systems and the scales at which they operate. For example, the problems caused by over-use of fertilizer or manure in parts of North America, Europe and China may well allow for reductions in input use and result in significant environ- mental benefits, without necessarily compromising crop yields or farmer incomes. In contrast, in many developing countries, and especially in Africa, crop productivity, and thus the food security and/or incomes of farming households, is tightly constrained by nutrient availability (i.e. because of highly weathered soils, and the limited availability of fertili- zer, manure and compostable organic matter) (e.g. Rufino et al., 2011). Under such circumstances continued cultivation inevitably leads to soil degradation, and the use of external inputs, Practice sheet 17 including fertilizer, is essential to increase crop yields, sustain soils and build soil C (Vanlauwe et al., 2014, 2015) “How sustainable is sustainable intensification?” - Joao ˜ Vasco Silva ✅ This academic paper examines the concept of sustainable intensification in the context of achieving food security and reducing the environmental impacts of agriculture. The paper analyses three contrasting farming systems—mixed crop-livestock systems in southern Ethiopia, specialized rice-based farming systems in Central Luzon (Philippines), and arable farming systems in the Netherlands—to evaluate the environmental and socio-economic sustainability of sustainable intensification at the farm level. The study found significant potential for future intensification of cereal production in southern Ethiopia. However, the current input use in these farming systems is deemed not economically and environmentally sustainable at the farm level. Similarly, in Central Luzon, while sustainable intensification can potentially narrow yield gaps and improve nitrogen use efficiency, profitability remains a challenge due to the reliance on costly hired labor. In contrast, arable farms in the Netherlands exhibit high economic performance and nitrogen use efficiency, but require further improvements in resource-use efficiency to enhance environmental sustainability. The paper highlights the importance of public investments in promoting innovation and making sustainable intensification technologies accessible and affordable to farmers. These investments are deemed crucial in ensuring that yield gaps can be narrowed while simultaneously meeting sustainability objectives at the farm level. intressante quotes Yield gaps are defined as the difference between the potential (Yp) or the water- limited yield (Yw) and the actual yield (Ya) observed in farmers’ fields under irrigated or rainfed conditions, respectively (van Ittersum et al., 2013). Decomposing yield gaps into efficiency, resource and technology yield gaps is helpful to identify the management drivers of existing yield gaps (Silva et al., 2017b). The efficiency yield gap is defined as the difference between the technically efficient yield (YTEx, the maximum yield that can be achieved for a given input Practice sheet 18 level) and Ya and captures the contribution of sub-optimal time, form and/or space of crop management practices. The resource yield gap is defined as the difference between the highest farmers’ yield (YHF) and YTEx and it is attributed to a sub- optimal amount of inputs applied. Finally, the technology yield gap is defined as the difference between Yp or Yw and YHF and it can be attributed to the use of inferior technologies (e.g., varieties or balanced nutrition) in farmers’ fields than those needed to reach Yp or Yw. Sustainable intensification involves trade-offs between ‘sustainabil ity’ and ‘intensification’ (Struik et al., 2014). Such trade-offs are likely to occur at the farm level given resource constraints and the timing of activities; some objectives are then prioritized over others. Few studies have paid attention to this in the past. Hence it remains unclear how sustainable intensification of crops (at field level) works out at farm level, given constraints of land, labour and capital availability and farmers’ decisions on resource allocation coupled with their prioritiza tion of crop management activities. Farmers’ decisions can be classified as strategic, tactical and operational in terms of long, intermediate and immediate time scales (de Koeijer et al., 1999). Their decisions deter mine actual resource-use efficiencies and the extent to which growth-defining, -limiting and -reducing factors are optimised for a specific crop in the biophysical environment of the farm (Giller et al., 2011). In turn, management decisions are strongly conditional on the socio- economic environment of the farm and the farmers’ personal priorities. “Divergent understandings of agroecology in the era of the African Green Revolution” - Imogen Bellwood-Howard ✅ Understandings of agroecology within mainstream development and how these relate to different agricultural development narratives. Using a "knowledge politics" approach, the authors identify five understandings of agroecology: 1. No knowledge of agroecology: This pertains to individuals or institutions unfamiliar with the concept. 2. Descriptive science: Agroecology as the scientific study of interactions between livestock, crops, and the environment. 3. Science for sustainability: Agroecology as a science geared towards designing sustainable agricultural systems, encompassing socio-economic and environmental considerations. Practice sheet 19 4. Environmentally sustainable farming practices: Agroecology as a set of practices aimed at minimizing external inputs and promoting environmental sustainability. 5. Political agroecology: Agroecology as a movement advocating for human and environmental well-being, food sovereignty, and social justice. The paper further identifies four agricultural development "regimes," each associated with specific understandings of agroecology: 1. African Green Revolution: Focused on increasing agricultural productivity through modern technologies and inputs. 2. 21st-Century Agroecology: Promoting a transition towards agroecological food systems based on principles of ecological sustainability, social justice, and food sovereignty. 3. Large-scale agriculture: Emphasizing large-scale agricultural production, often for export markets. 4. Smallholder agriculture: Characterized by small, diverse, low-input, low-output farms, primarily focused on subsistence farming. The authors argue that proponents of these different regimes often emphasize the distinctions between their favored understandings of agroecology, while neglecting to acknowledge the historical evolution and diverse framing of agroecological science.32 This tendency obscures the political nature of scientific knowledge construction and how different interpretations of agroecology can support contrasting development paradigms. intressante sitat Many contemporary agroecological publications consider social or political science concerns such as food sover- eignty, community empowerment and environmental jus- tice (e.g. de Molina, 2013), rather than interactions between crops, livestock and their physical environment Especially since the 1990s, varied understandings of agroe- cology have interacted with proposed regimes of agricul- tural development. Recognition of either a more political or a more technical understanding can be one factor contribut- ing to dominance of a given development paradigm. As different development trajectories can have far-reaching economic, environmental and cultural implications, the definition of agroecology is more than just an academic preoccupation. Although different actors may not recog- nize the discursive power exercised by promoting various definitions, groups with different perspectives on agroecol- ogy have Practice sheet 20 occasionally done discursive work on the term’s definition. An absence of such debate in some contexts also has a bearing, albeit likely unintentional, on the trajectory of agricultural development. Both paradigms also have a common root in agroecolo- gical science. Thus, Altieri’s (1995) book, published as the political understanding of agroecology was taking shape, is called ‘Agroecology: The Science of Sustainable Agricul- ture’. The 21st- century agroecology regime in particular relies on the definition of agroecology as a science being used alongside the political agroecology definition. With- out this, there is no basis for claiming that certain practices are environmentally desirable, and therefore that political and economic systems should be managed to maintain them. Simultaneously, Conway’s (1997) book, the ‘Doubly Green Revolution: Food for all in the 21st Century’, which can be seen as a precursor to the AGR, draws on a deep understanding of the ecology of agricultural systems to advocate extensive use of advanced technologies to pro- mote food security The least political approach takes it for granted that the attainment of yield is the primary objective of agriculture and agronomy, so agroecological science is understood as mobilizing the understanding of ecological processes towards that end. Simultaneously, since its earli- est mention, there has been a perspective that agroecology is about using ecological understanding to ensure agricul- ture is environmentally and socially ‘harmonious’: Hanson (1939, p.113) proposed ‘the need for natural areas as checks, or standards, by which the values and effects of tillage, irrigation, drainage, grazing, lumbering, and other uses may be measured’. Much later, Gliessman (2015) pre- sented the same ecological processes and ecosystem com- ponents in such a way as to explicitly promote a specific vision of sustainable agricultural development Navigating the Complexities of Sustainable Agriculture: A Synthesis of Key Concepts The sources explore different farming styles and approaches aimed at achieving sustainable food production amidst a growing global population. They highlight the complexities and challenges inherent in balancing productivity, environmental sustainability, and social equity. A core theme that runs throughout the sources is that no single solution fits all contexts; local realities must be carefully considered when evaluating the applicability and Practice sheet 21 effectiveness of different agricultural practices. Let's examine some of the key concepts discussed: Sustainable Intensification: This concept emphasises increasing agricultural output on existing farmland while minimising environmental impact. It involves closing yield gaps, which are the differences between potential and actual crop yields. Sustainable intensification seeks to achieve "more output with less input". However, this is often easier said than done, as illustrated by the case studies presented by Silva et al. In southern Ethiopia, for example, intensification is needed to improve food security, but current practices are not economically or environmentally sustainable. This highlights the need to tailor intensification strategies to local conditions and consider social and economic factors alongside environmental concerns. Regenerative Agriculture: This relatively new concept focuses on restoring and enhancing natural systems within agricultural landscapes. It emphasizes soil health, biodiversity conservation, and carbon sequestration as key outcomes. Proponents of regenerative agriculture advocate for practices such as minimizing tillage, maintaining soil cover, and integrating livestock to improve soil fertility and biodiversity. However, Giller et al. caution against a universalist approach to regenerative agriculture, arguing that the effectiveness of specific practices can vary significantly depending on the local context. They urge researchers to critically evaluate the claims of regenerative agriculture and consider potential unintended consequences. Agroecology: This concept is particularly contested due to its various interpretations and the political dimensions associated with it. It encompasses a range of perspectives, from a purely scientific approach to a political movement advocating for social justice and food sovereignty. Bellwood-Howard and Ripoll identify five distinct understandings of agroecology: descriptive science, science for sustainability, environmentally sustainable farming practices, political agroecology, and a lack of awareness of the concept altogether. These diverse interpretations often lead to confusion and disagreement over what constitutes "true" agroecology. The authors argue that acknowledging the historical evolution of agroecology and understanding the different ways it has been framed can help clarify these debates. The sources also touch upon other important aspects of sustainable agriculture, such as: Yield Gaps and Their Drivers: Practice sheet 22 The concept of yield gaps is central to understanding the potential for sustainable intensification. Yield gaps can be decomposed into efficiency, resource, and technology gaps, helping to identify the specific factors limiting agricultural productivity. These factors can include sub-optimal management practices, insufficient input use, and the adoption of inferior technologies. Farm Size and Labour Use: The scale of agricultural operations and the availability of labor are crucial factors influencing sustainable intensification strategies. The Silva et al. study highlights the contrasts between smallholder farming systems in Ethiopia and the Philippines, where labor is abundant and capital is scarce, and large-scale farming systems in the Netherlands, characterized by high levels of mechanisation and capital investment. Economic and Social Sustainability: Sustainable agriculture requires considering not only environmental impacts but also economic viability and social equity. Ensuring that farmers can make a living from sustainable practices is crucial for their adoption and long-term success. This may involve supporting innovation, making technologies accessible and affordable, and promoting fair market access for smallholder farmers. In summary, the sources paint a complex picture of the challenges and opportunities associated with sustainable agriculture. They emphasize the need for context-specific solutions, critical evaluation of different approaches, and consideration of both environmental and socio-economic factors. The sources provide a valuable framework for understanding the key concepts and debates surrounding sustainable food production and offer insights that can inform the development of more effective and equitable agricultural practices. Terminologies and Definitions Agroecology: A multifaceted concept with various interpretations, encompassing scientific study, social movement, and farming practices. It can be understood as: Descriptive science: Studying ecological interactions within agricultural systems. Science for sustainability: Applying ecological knowledge to design sustainable agricultural systems, often considering socio-political aspects like food sovereignty and environmental justice. Environmentally sustainable farming practices: Low-external-input practices promoting environmental sustainability, like composting, intercropping, and crop Practice sheet 23 rotations. Political agroecology: Advocating for food sovereignty, social justice, and transformative change in food systems. Agronomic practices: Specific methods and techniques used in agricultural production. The sources discuss various practices associated with regenerative agriculture, including: Zero tillage/Reduced tillage/Conservation agriculture: Minimizing soil disturbance to preserve soil structure and organic matter. Cover cropping: Growing non-cash crops to protect and improve soil health. Mulching: Applying organic or inorganic materials to the soil surface for moisture retention, weed suppression, and temperature regulation. Biochar: Charred organic matter added to soil to improve fertility and carbon sequestration. Compost/Green manures/Animal manures: Organic amendments to enhance soil fertility and nutrient cycling. Agroforestry/Silvopasture/Tree crops: Integrating trees into agricultural systems for various benefits, including carbon sequestration and biodiversity enhancement. Permaculture: A design system aiming to create sustainable human settlements by mimicking natural ecosystems. Biodiversity: The variety of life in a particular habitat or ecosystem. Regenerative agriculture often claims to reverse biodiversity loss. Efficiency yield gap: The difference between the technically efficient yield (achievable with optimal management for given inputs) and the actual yield, reflecting sub-optimal timing, form, or placement of crop management practices. Good Agricultural Practices: Established guidelines for environmentally and socially responsible farming, encompassing various practices. Green Revolution: A period of significant agricultural productivity increases in the mid-20th century, driven by technological advancements like high-yielding varieties and synthetic fertilizers. Practice sheet 24 African Green Revolution: Similar aims to the Green Revolution, but focused on increasing agricultural productivity in Africa. Integrated Pest Management (IPM): An ecosystem-based strategy emphasizing pest prevention and minimizing pesticide use through various methods. Nitrogen surplus: The excess nitrogen input into agricultural systems beyond what crops can uptake, potentially leading to environmental pollution. Nitrogen use efficiency (NUE): A measure of how effectively plants utilize nitrogen fertilizer. Organic agriculture: A farming system emphasizing ecological principles and minimizing synthetic inputs. Regenerative agriculture: An approach aiming to regenerate and enhance agricultural ecosystems, often focusing on soil health, biodiversity, and ecosystem services. Regenerative organic agriculture: A subset of regenerative agriculture that adheres to organic farming principles. Resource yield gap: The difference between the highest farmer's yield (achievable with best practices and available resources) and the technically efficient yield, indicating limitations from insufficient inputs. Soil health: A complex concept often lacking precise definition, referring to the capacity of soil to function as a living ecosystem supporting plant growth and other ecosystem services. Sustainable intensification: Increasing agricultural productivity while minimizing environmental impact and promoting social equity. Technology yield gap: The difference between the potential yield (achievable with optimal conditions and technologies) and the highest farmer's yield, indicating limitations from using inferior technologies. Practice sheet 25 Yield gap: The difference between the potential yield (or water-limited yield in rainfed conditions) and the actual yield achieved by farmers, indicating the potential for improvement. Yield gap decomposition: Analyzing the yield gap by breaking it down into different components, such as efficiency, resource, and technology yield gaps. 4. Resilience and Food Systems texts: Food System Resilience: Concepts, Issues, and Challenges ✅ intressante sitater We present four questions to frame food system resilience (Resilience of what? Resilience to what? Resilience from whose perspective? Resilience for how long?) and three approaches to enhancing resilience (robustness, recovery, and reorientation—the three “Rs”) Shocks are abrupt events of differing probability of occurrence and severity of impact, and may even be wholly unimagined: a surprise (32). Analysis of a half- century of drivers of shocks for both land- and marine-based food systems around the world identified extreme weather events, geopo- litical events, financial market crashes, and short-term cost spiraling of fertilizer and other inputs, disease outbreaks, and conflict as major shocks (33), often compounded by policy change and mis- management. Food safety scares can also significantly “shock” the system (34). The impacts of a combination of shocks, such as extreme weather and disease outbreak, can be particularly signifi- cant, as exemplified in the sidebar titled Interacting Shocks to Banana Production in Colombia Compared with shocks, food system stresses are longer-term drivers or conditions that are more easily perceived. Examples of stresses include gradual changes in landscape-level land use and agrochemical use, dietary shifts, climate change, demographic change, regulatory alterations, trends in commodity prices, and functional biodiversity loss (35–39). Practice sheet 26 Fan et al. (30) refer to risks as decision-making situations in which the likelihoods of potential outcomes are known to the decision-maker (whereas in uncertain situations, they are not). Risks can vary in detectability, the likelihood of an adverse current or future occurrence, and the severity of their impact (49). They can be examined through, for example, foresight and scenario analysis (48). However, risk percep- tion is often heavily dependent on contextual factors (50). Hazards that provoke a particular sense of dread, are unfairly distributed, or are unmitigable may prompt heightened risk perceptions (50, 51). Social processes may amplify risk perception through media (52), which can produce a knock-on effect, such as the panic buying witnessed during the COVID-19 pandemic (53, 54) Holling (59) coined the now common ecological systems definition of resilience as a measure of the persistence of systems and their ability to absorb change and disturbance and still maintain the same relationships between populations or state variables. This equates to our concept of the functioning of the system. Thresholds are central to this definition of resilience. Severe perturba- tions can potentially trigger numerous reactions across spatial or temporal scales that can bring the system over a threshold, causing it to shift to a new state. Similarly, small shifts in system func- tioning, which are not visible, can move system functioning toward a precipice, where additional perturbation creates a systemic change in ecosystem functioning (60). Resilience recognizes that a system can have multiple stable states and can maintain function as a result of internal reorga- nization, i.e., its adaptive capacity (61). Béné et al. (62) suggested three important components to consider: the abilities of a system (a) to absorb losses due to disturbances, (b) to adapt through learning and incremental adjustments, or even (c) to transform through radical changes in the face of stresses and shocks Helfgott (13) identified four key framing questions (the four “Qs”), answers to which help to define the boundaries of the systemic resilience sought; they point to the inclusion, exclusion, and marginalization of certain stakeholders and the issues that concern them (87). 1. Resilience of what? Is it the soil, the crop, the farm enterprise, the local market as an in- stitution, food supply, or the food system more generally? We can consider the food system activities (the functioning), the outcomes of these activities (the function), or both. Although certain individuals may have a particular interest in specific activities (e.g., farmers in farm- ing, caterers in catering), we argue that from a societal-level viewpoint, the interest lies in the resilience of the overall food system outcomes (Figure 1) rather than in the Practice sheet 27 individual activities per se; food security is one of the four highly valued features in a society (88). 2. Resilience to what? We need to understand the nature of the individual shocks and stresses that affect the food system and how they may interact to amplify the overall impact. Re- silience depends largely on the severity and frequency of the shock or stress to which the system is exposed. The shock or stress can be external to the food system (e.g., an extreme weather event or demographic change) or internal (e.g., a food safety outbreak or dietary change). 3. Resilience from whose perspective? Is it from the perspective of a given actor in the sys- tem (e.g., a farmer or a retailer) or from that of a policymaker, or company CEO, or society at large? We need to know which features of the system need to be preserved, which can change, and what constitutes desirable change (improvement) for whom, and from whose perspective. This question is important for understanding power, justice, and equity, as well as trade-offs between food system outcomes, relative to different system actors. 4. For what time period do we need to build resilience? It is important to distinguish short-term interruptions due to shocks (e.g., bad weather or an IT malfunction interrupting just-in-time fresh grocery deliveries) from disruptions due to stresses that affect the longer term (e.g., changing dietary preferences, shifting cropping regions). It is also important to understand the interactions between the two and the notion of temporal mismatches (89). In the context of dynamically shifting risk environments, strategies to enhance resilience over a shorter timescale may deplete resilience over the longer term, necessitating specification of the time frame over which resilience is being considered (90). Resilience-building measures need to account for temporal dimensions Practice sheet 28 This article, authored by Martin Zurek and colleagues, explores the multidimensional nature of food system resilience, drawing on concepts from both food system studies and resilience research. Food Systems as Social-Ecological Systems: The authors emphasize the understanding of food systems as complex social-ecological systems (SES) that operate across multiple scales—spatial, temporal, and jurisdictional. These systems involve various actors driven by diverse forces, resulting in a range of outcomes, including food security, environmental impacts, and socio-economic effects. Shocks and Stresses: The article distinguishes between shocks, which are sudden, disruptive events (e.g., extreme weather, pandemics), and stresses, which are longer- term, gradual pressures (e.g., climate change, demographic shifts). Both shocks and stresses can significantly affect food system outcomes and require different resilience-building approaches. Framing Resilience: The authors identify four key questions (referred to as the four "Qs") that are essential for framing food system resilience. These questions help define the boundaries of the system and guide the development of appropriate strategies: Resilience of what? (the activities/functions, outcomes/functions, or both?) Resilience to what? (specific shocks and stresses) Resilience from whose perspective? (farmers, policymakers, society, etc.?) Resilience for how long? (short-term, long-term) The Three "Rs" of Resilience: The article proposes three main approaches to enhancing food system resilience: Robustness: This involves maintaining the status quo of desired food system outcomes by preventing shocks and stresses from disrupting them. It often focuses on absorbing the impacts of disruptions and requires adapting activities to withstand them. For example, a retailer may adapt their supply chain to maintain stock even if one source is disrupted. Recovery: This approach focuses on returning the system to its original state after being disrupted. It emphasizes bouncing back from shocks and stresses and requires adapting activities to facilitate recovery. For example, a farmer might implement better seed storage to recover from a poor harvest. Practice sheet 29 Reorientation: This involves rejecting the status quo and accepting alternative food system outcomes, potentially before or after a disruption occurs. It requires adapting activities to achieve the desired transformed outcomes. For instance, this could involve shifting to more sustainable farming practices or promoting local food production. The authors acknowledge that operationalizing these strategies involves normative choices and negotiating trade-offs between different food system actors and their desired outcomes. Adaptation and Transformation: The article differentiates between adaptation as changing food system activities and transformation as changing food system outcomes. It argues that transforming an outcome from state A to state B requires actors to adapt their activities, highlighting the need for changing drivers like policies and economic incentives to stimulate those adaptations. Assessing Resilience: The authors highlight the challenges in assessing food system resilience, noting that most efforts have focused on specific contexts, like development or food security, with limited consideration of broader components and objectives. They call for the development of more comprehensive metrics and indexes to accurately assess resilience across multiple dimensions. Questions and Issues: The article concludes by raising several questions and issues that need further exploration: To what degree is food system resilience an emergent property influenced by the shape and structure of the system? How do we balance the resilience of individual actors with the resilience of the system as a whole? How do we assess food system resilience effectively, considering the complexity of relationships between multiple variables? How do we manage potential trade-offs between resilience and sustainability aims? Addressing these questions is crucial for developing more resilient food systems that can withstand future shocks and stresses. An Indicator Framework for Assessing Agroecosystem Resilience ✅ intressante sitater Essentially, resilience is measured in three ways: (1) the amount of change the system can undergo and still retain the same controls on function and structure; (2) Practice sheet 30 the degree to which the system is capable of self- organization; and (3) the ability to build and increase the capacity for learning and adaptation We use intentionality to bound the agroecosystem (Bland and Bell 2007); as such, the agroecosystem can be defined as an ecosystem managed with the intention of producing, distributing, and consuming food, fuel, and fiber. Its boundaries encompass the physical space dedicated to production, as well as the resources, infrastructure, markets, institutions, and people that are dedicated to bringing food to the plate, fiber to the factory, and fuel to the hearth. The agroecosystem operates simultaneously at multiple nested scales and hierarchies, from the field to the globe. Here, we are concerned with a scale greater than the individual farmer and his or her farm, but a scale small enough that an individual’s voice can still make a difference. This is because no farmer operates in a vacuum, and the decisions he or she makes are based in large part on outside influences (Darnhofer et al. 2010b). However, those outside influences should still be subject to feedback from those who are affected by them. This article, written by Joshua F. Cabell and Myles Oelofse, focuses on applying resilience theory to agroecosystems, which are ecosystems managed for food, fuel, and fibre production. The authors acknowledge the complexity of these systems and argue that resilience cannot be directly measured. Instead, they advocate for using behaviour- based indicators to assess resilience, drawing on the concept of biotic indicators used in ecosystem monitoring. Challenges in Measuring Resilience: Cabell and Oelofse agree with Darnhofer et al. (2010a) that the complexity and variability of agroecosystems make precise measurement of resilience impractical. They highlight the challenges in defining the “resilience of what to what” due to the nested nature of these systems. Behavior-Based Indicators: Instead of direct measurement, the authors propose using a set of behaviour-based indicators, which are observable characteristics that suggest resilience when present. They compiled 13 such indicators from resilience literature, including: Socially self-organized: This refers to communities having the capacity to manage their own resources and adapt to change without excessive external control. Practice sheet 31 Ecologically self-regulated: This indicates that the agroecosystem can regulate essential processes, like nutrient cycling and pest control, with minimal human intervention. Appropriately connected: This highlights the importance of diverse and flexible relationships between different components of the system, as opposed to rigid dependencies. High degree of functional and response diversity: This includes having a variety of species and farming practices that contribute different functions and respond differently to disturbances, increasing the system’s adaptability. Optimally redundant: This refers to having “backups” or alternative options within the system to compensate for potential failures. While redundancy may reduce efficiency, it can be crucial for resilience during shocks and stresses. Spatial and temporal heterogeneity: This relates to the diversity of landscape features and farming practices across space and time, contributing to greater ecological and social resilience. Manages slow variables and feedbacks: This emphasizes the importance of managing key processes that change slowly over time, such as soil health, water cycles, and biodiversity. Responsibly coupled with local natural capital: This indicator suggests that the agroecosystem operates within the limits of its local resource base and minimizes reliance on external inputs. Reflective and shared learning: This highlights the importance of learning from past experiences and sharing knowledge within the community to enhance adaptive capacity. Embraces innovation: This refers to the willingness to adopt new technologies and practices that can contribute to resilience, while also considering their potential risks. Maintains human capital: This involves investing in the skills, knowledge, and well-being of the people involved in the agroecosystem. Adequate financial capital: This emphasizes the importance of having access to financial resources to invest in resilience-building measures and cope with unexpected events. Polycentric governance: This suggests a system of governance where multiple actors and institutions share power and responsibility, contributing to greater Practice sheet 32 flexibility and responsiveness. The presence of these indicators can help identify resilient agroecosystems, while their absence reveals vulnerabilities that require attention. This approach provides a practical framework for assessing and enhancing the resilience of farming and food systems to ensure long-term sustainability. Do you bend or break? System dynamics in resilience planning for food security - Hugo Jose Herrera de Leon* and Birgit Kopainsky ✅ intressante quotes Food systems are a particular type of social–ecological system (SES) man- aged with the primary purpose of producing food and, more specifically, of providing food security to their stakeholders (Ericksen, 2008a). FAO (2002, p. 50) defines food security as follows: “all people, at all times, have physical and economic access to sufficient, safe and nutritious food to meet their die- tary needs and food preferences for an active and healthy life” In their 2018 report, the Food and Agriculture Organization of the United Nations (FAO, 2018, p. 1) reported, for example, that “70–80 percent of severely food insecure people worldwide rely on agriculture-based livelihoods”. All these households are on the verge of disaster as climate becomes unpredictable and extreme events such as floods and droughts are more common Resilience, at a basic level, can be understood as the ability of a system to cope with, and adapt to, changes (Holling, 1996; Folke, 2006; Marshall and Marshall, 2007). Resilience is used in both theoretical and practical settings as a means to characterise systems but also as a framework to improve its adaptive capacity (Folke et al., 2004; Walker et al., 2006). In this paper we focus on the latter, and particularly on the way resilience is applied as a framework to study how systems can withstand bigger and/or more frequent changes. We look at resilience as an outcome of the planning and risk man- agement processes (Berkes and Folke, 1998; Chapin et al., 2009) and empha- sise those attributes that can help a system to live with, learn from and adapt to change In the past, case study research has been used to identify system attributes and characteristics that contribute to resilience. By studying systems that have been identified as resilient, researchers have proposed that attributes such as redundancy, connectivity or polycentrism contribute to enhance systems’ resilience. Practice sheet 33 “Hardness” indicates the threshold between stability and adaptation, i.e. the degree of disturbance needed to perturb the outcome’s stability (see Figure 2). Similarly, the measure “elasticity” indicates the threshold between adaptation and transformation, and the “index of resil- ience” indicates the probability of reaching this threshold (see Figure 2). Practice sheet 34 summary This article, written by Hugo Jose Herrera de Leon and Birgit Kopainsky, explores the use of system dynamics in creating resilience plans for food security in Guatemalan communities facing climate pressures. The authors criticize the traditional deductive approach to resilience planning, which relies on broadly accepted factors like redundancy, connectivity, and polycentrism. They argue that this approach is often too simplistic and advocate for a more nuanced understanding of the structure-behaviour relationship in complex dynamic systems. What is Resilience? The authors define resilience as a system's ability to cope with and adapt to change. They emphasize the importance of planning and risk management in achieving resilience. System Dynamics in Resilience Planning: The paper advocates for using system dynamics (SD) to explore the complex mechanisms behind resilience. They highlight the success of SD in areas like water management and CO2 emissions reduction. SD focuses on how stocks, flows, and feedback structures influence a system’s behaviour, which makes it particularly well-suited for resilience planning. Case Study in Guatemala: The authors used SD to study resilience planning for food security in subsistence food systems in Guatemala. They focused on two districts, Huehuetenango and Jutiapa, and developed a general model to understand the behaviour and processes of maize-dominated agriculture systems. The model was based on publicly available data and validated through continuous engagement with stakeholders. Feedback Loops and Policies: The model identified key feedback loops influencing food security resilience, including: Commercial agriculture: Increased maize revenue allows for more spending on farming, leading to better yields and further revenue increases. Poverty trap: Dedicating a larger proportion of maize to self-consumption reduces sales and may lead to a cycle of lower revenues. Market’s invisible hand: High production reduces demand and lowers prices, while low production increases demand and raises prices. The study also tested the resilience of food consumption to drought intensification, using historical data and simulating various drought scenarios. The authors facilitated discussions with stakeholders to propose policies for enhancing resilience, including Practice sheet 35 promoting home gardens, increasing poultry farming, improving market infrastructure, and supporting irrigation systems. Policy 2, increasing poultry farming, was found to be most effective in increasing system elasticity, particularly in Huehuetenango. The authors stress that resilience planning requires understanding the dynamic complexity of the systems involved and that policy recommendations should vary from case to case. Briefing Doc: Food System Resilience This document provides an overview of food system resilience, drawing on three key sources. It explores the concept of resilience, its application to food systems, frameworks for understanding and enhancing resilience, and the challenges in assessing it. What is Resilience? Resilience is broadly defined as the ability of a system to cope with and adapt to change. This concept is particularly relevant for food systems, which face various shocks and stresses, such as climate change, extreme weather events, economic fluctuations, and pandemics. Food Systems as Complex Adaptive Systems Food systems are viewed as complex adaptive systems, operating across multiple scales and involving various actors, activities, and outcomes. These systems are characterized by: Interconnectedness: Different components of the food system, such as production, processing, distribution, and consumption, are interconnected and influence each other. Feedback loops: Actions within the food system can create feedback loops that either reinforce or dampen change. Non-linearity: The relationships between different components are often non-linear, meaning small changes can lead to significant and unpredictable outcomes. Framing Food System Resilience Before discussing resilience strategies, it's crucial to establish a clear understanding of what we want to make more resilient and to what threats. This involves answering four key questions (the four "Qs"): 1. Resilience of what? Are we aiming for the resilience of: Specific activities or functions within the food system (e.g., farming prac