Structure and Function of White Blood Cells and Immunity PDF
Document Details
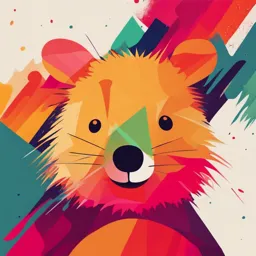
Uploaded by InstructiveTheme
BAU Medical School
Tags
Summary
This document discusses the structure and function of white blood cells and immunity, covering learning outcomes, different types of white blood cells and their roles. It also provides insights into innate and acquired immunity, various aspects like chemotaxis, phagocytosis, and the complement system.
Full Transcript
Structure and Functions of White Blood Cells & Immunity Learning Outcomes 1. Explain the types of white blood cells and their functions. 2. Explain the importance of the leukocyte formula. 3. Describe the components and functions of the immune system. 4. Differentiate innate and acquired immunity....
Structure and Functions of White Blood Cells & Immunity Learning Outcomes 1. Explain the types of white blood cells and their functions. 2. Explain the importance of the leukocyte formula. 3. Describe the components and functions of the immune system. 4. Differentiate innate and acquired immunity. 5. Identify the roles of the humoral and cellular arms of acquired immunity. 6. Describe the regulation of immunity by soluble cytokines and the complement system. 7. Define the roles of additional circulating and tissue cell types that contribute to immune response such as granulocytes, mast cells, and monocytes. • Our bodies have a special system for combating the different infectious and toxic agents. • This system is composed of blood leukocytes (white blood cells [WBCs]) and tissue cells derived from leukocytes. • These cells work together in two ways to prevent disease: (1) by actually destroying invading bacteria or viruses by phagocytosis; and, (2) by forming antibodies and sensitized lymphocytes that may destroy or inactivate the invader. White Blood Cells • White blood cells (leukocytes), are the mobile units of the body’s protective system. • They are formed partially in the bone marrow (granulocytes and monocytes and a few lymphocytes) and partially in the lymph tissue (lymphocytes and plasma cells). • After formation, they are transported in the blood to different parts of the body where they are needed. • The real value of WBCs is that most of them are specifically transported to areas of serious infection and inflammation, thereby providing a rapid and potent defense against infectious agents. Types of White Blood Cells Five types of WBCs are normally present in the blood: Neutrophils (polymorphonuclear), Lymphocytes, Monocytes, Eosinophils (polymorphonuclear), Basophils (polymorphonuclear), and, occasionally, plasma cells. • Basophils, eosinophils, and neutrophils are called granulocytes because they contain cytoplasmic inclusions that give them a granular appearance. • They are polymorphonuclear cells. • The granulocytes and monocytes protect the body against invading organisms by ingesting them (by phagocytosis) or by releasing antimicrobial or inflammatory substances that have multiple effects that aid in destroying the offending organism. • The leukocytes of the agranulocyte group include monocytes and lymphocytes. • Monocytes generally arise from red bone marrow. • Lymphocytes are formed in the organs of the lymphatic system, as well as in the red bone marrow. • The lymphocytes and plasma cells function mainly in connection with the immune system. Concentrations of Different White Blood Cells in Blood • An adult human has about 7000 WBCs per microliter of blood cell (4.000–11.000 per microliter of blood). • Of the total WBCs, the normal percentages of the different types are approximately the following: • Neutrophils: 62.0% • Eosinophils: 2.3% • Basophils: 0.4% • Monocytes: 5.3% • Lymphocytes: 30.0% Hematopoietic lineages. CFU, colony forming unit—with suffixes – Baso (basophilic), -E (erythroid), -Eo (eosinophil), -G (granulocyte), GEMM (granulocyte, erythrocyte, megakaryocyte, macrophage), -GM (granulocyte/macrophage), -M (macrophage), -Meg (megakaryocyte), -MegE (megakaryocyte/erythroid); CMP, common myeloid progenitor; CLP, common lymphoid progenitor; GMP, granulocyte-macrophage progenitor; MEP, megakaryocyteerythroid progenitor. • Pluripotent long-term hematopoietic stem cells (LT-HSCs) constitute a population of adult stem cells found in bone marrow that are multipotent and able to self-renew. • The short-term hematopoietic stem cells (STHSCs) give rise to committed stem cells or progenitors, which after proliferation can differentiate into lineages that in turn give rise to burst-forming units (BFUs) or colonyforming units (CFUs), each of which ultimately will produce one or a limited number of mature cell types: • erythrocytes, • the megakaryocytes that give rise to platelets, • eosinophils, • basophils, • neutrophils, • monocytes-macrophages/dendritic cells, and • B or T lymphocytes and natural killer cells. • Soluble factors known as cytokines guide the development of each lineage. Hematopoietic lineages. CFU, colony forming unit—with suffixes – Baso (basophilic), -E (erythroid), -Eo (eosinophil), -G (granulocyte), GEMM (granulocyte, erythrocyte, megakaryocyte, macrophage), -GM (granulocyte/macrophage), -M (macrophage), -Meg (megakaryocyte), -MegE (megakaryocyte/erythroid); CMP, common myeloid progenitor; CLP, common lymphoid progenitor; GMP, granulocyte-macrophage progenitor; MEP, megakaryocyteerythroid progenitor. A family of hematopoietic cytokines stimulates colony formation by progenitor cells, the colonystimulating factors. The main colony-stimulating factors are: • granulocyte-macrophage colonystimulating factor (GM-CSF), • granulocyte colony-stimulating factor (G-CSF), • macrophage colony-stimulating factor (M-CSF), • interleukin-3 (IL-3) and IL-5, • thrombopoietin (TPO), and • erythropoietin (EPO). • The granulocytes and monocytes are formed only in the bone marrow. • Lymphocytes and plasma cells are produced mainly in the various lymphogenous tissues— especially the lymph glands, spleen, thymus, tonsils, and various pockets of lymphoid tissue elsewhere in the body, such as in the bone marrow and in Peyer’s patches underneath the epithelium in the gut wall. • The WBCs formed in the bone marrow are stored in the marrow until they are needed in the circulatory system. Then, when the need arises, various factors cause them to be released. • Normally, about three times as many WBCs are stored in the marrow as circulate in the entire blood. • This quantity represents about a 6-day supply of these cells. • The lymphocytes are mostly stored in the various lymphoid tissues, except for a small number that are temporarily being transported in the blood. Life Span Of White Blood Cells • The life of the granulocytes after being released from the bone marrow is normally 4 to 8 hours circulating in the blood and another 4 to 5 days in tissues where they are needed. • In times of serious tissue infection, this total life span is often shortened to only a few hours because the granulocytes proceed even more rapidly to the infected area, perform their functions, and in the process, are themselves destroyed. • The monocytes also have a short transit time, 10 to 20 hours in the blood, before wandering through the capillary membranes into the tissues. • Once in the tissues, they swell to much larger sizes to become tissue macrophages and, in this form, they can live for months unless destroyed while performing phagocytic functions. These tissue macrophages are the basis of the tissue macrophage system, which provides continuing defense against infection. • Lymphocytes enter the circulatory system continually, along with drainage of lymph from the lymph nodes and other lymphoid tissue. • After a few hours, they pass out of the blood back into the tissues by diapedesis/extravasation. • Then, they re-enter the lymph and return to the blood again and again; thus, there is continual circulation of lymphocytes through the body. • Lymphocytes have life spans of weeks or months, depending on the body’s need for these cells. Neutrophils And Macrophages Defend Against Infections • It is mainly the neutrophils and tissue macrophages that attack and destroy invading bacteria, viruses, and other harmful agents. • The neutrophils are mature cells that can attack and destroy bacteria, even in the circulating blood. • Conversely, the tissue macrophages begin life as blood monocytes, which are immature cells while still in the blood and have little ability to fight infectious agents at that time. • However, once they enter the tissues, they begin to swell—sometimes increasing their diameters as much as fivefold—to as great as 60 to 80 micrometers, a size that can barely be seen with the naked eye. • These cells are now called macrophages, and they are extremely capable of combating disease agents in the tissues. White Blood Cells Enter the Tissue Spaces by Diapedesis • Neutrophils and monocytes can squeeze through gaps between endothelial cells of the blood capillaries and postcapillary venules by diapedesis. • Although the intercellular gaps are much smaller than a cell, a small portion of the cell slides through the gap at a time; the portion sliding through is momentarily constricted to the size of the gap. Movement of neutrophils by diapedesis or extravasation through capillary pores and by chemotaxis toward an area of tissue damage. • Both neutrophils and macrophages can move through the tissues by ameboid motion. https://www.youtube.com/watch?v=t2Me_z3x74A White Blood Cells Are Attracted to Inflamed Tissue Areas by Chemotaxis Many different chemical substances in the tissues cause both neutrophils and macrophages to move toward the source of the chemical. This phenomenon is known as chemotaxis. When a tissue becomes inflamed, at least a dozen different products that can cause chemotaxis toward the inflamed area are formed. They include the following: (1) some of the bacterial or viral toxins; (2) Degenerative products of the inflamed tissues; (3) several reaction products of the complement complex activated in inflamed tissues; and (4) Several reaction products caused by plasma clotting in the inflamed area, as well as other substances. The chemotaxis depends on the concentration gradient of the chemotactic substance. The concentration is greatest near the source, which directs the unidirectional movement of the WBCs. Chemotaxis is effective up to 100 micrometers away from an inflamed tissue. Therefore, because almost no tissue area is more than 50 micrometers away from a capillary, the chemotactic signal can easily move hordes of WBCs from the capillaries into the inflamed area. Movement of neutrophils by diapedesis or extravasation through capillary pores and by chemotaxis toward an area of tissue damage. Phagocytosis • A major function of the neutrophils and macrophages is phagocytosis, which means cellular ingestion of the offending agent. • Phagocytes must be selective of the material that is phagocytized; otherwise, normal cells and structures of the body might be ingested. Whether phagocytosis will occur especially depends on three selective procedures: • First, most natural structures in the tissues have smooth surfaces, which resist phagocytosis. However, if the surface is rough, the likelihood of phagocytosis is increased. • Second, most natural substances of the body have protective protein coats that repel the phagocytes. Conversely, most dead tissues and foreign particles have no protective coats, which makes them subject to phagocytosis. • Third, the immune system of the body develops antibodies against infectious agents such as bacteria. The antibodies then adhere to the bacterial membranes and thereby make the bacteria especially susceptible to phagocytosis. Phagocytosis of pathogens, such as bacteria, by a phagocytic cell, such as a macrophage. Antibodies coat the bacteria, making them more susceptible to phagocytosis by the macrophage that engulfs the bacterium, bringing it into the cell and forming a phagosome. Lysosomes then attach to the phagosome to form a phagolysosome, which digests the invading pathogen. The phagocytic cell then releases the digested products by exocytosis. Once Phagocytized, Most Particles Are Digested by Intracellular Enzymes. • Once a foreign particle has been phagocytized, lysosomes and other cytoplasmic granules in the neutrophil or macrophage immediately come into contact with the phagocytic vesicle, and their membranes fuse, thereby dumping many digestive enzymes and bactericidal agents into the vesicle. • Thus, the phagocytic vesicle now becomes a digestive vesicle, and digestion of the phagocytized particle begins immediately. • Both neutrophils and macrophages contain an abundance of lysosomes filled with proteolytic enzymes especially geared for digesting bacteria and other foreign protein matter. • The lysosomes of macrophages (but not of neutrophils) also contain large amounts of lipases, which digest the thick lipid membranes possessed by some bacteria, such as the tuberculosis bacillus. Phagocytosis of pathogens, such as bacteria, by a phagocytic cell, such as a macrophage. Antibodies coat the bacteria, making them more susceptible to phagocytosis by the macrophage that engulfs the bacterium, bringing it into the cell and forming a phagosome. Lysosomes then attach to the phagosome to form a phagolysosome, which digests the invading pathogen. The phagocytic cell then releases the digested products by exocytosis. Neutrophils and Macrophages Can Kill Bacteria • In addition to the digestion of ingested bacteria in phagosomes, neutrophils, and macrophages contain bactericidal agents that kill most bacteria, even when the lysosomal enzymes fail to digest them. • This characteristic is especially important because some bacteria have protective coats or other factors that prevent their destruction by digestive enzymes. • Much of the killing effect results from several powerful oxidizing agents formed by enzymes in the membrane of the phagosome or by a special organelle called the peroxisome. • These oxidizing agents include large quantities of superoxide (O2−), hydrogen peroxide (H2O2), and hydroxyl ions (OH−), which are lethal to most bacteria, even in small quantities. • Also, one of the lysosomal enzymes, myeloperoxidase, catalyzes the reaction between H2O2 and chloride ions to form hypochlorite, which is exceedingly bactericidal. Eosinophils • The eosinophils normally constitute about 2% of all the blood leukocytes. • Eosinophils are weak phagocytes, and they exhibit chemotaxis, but in comparison with neutrophils, it is doubtful that eosinophils are significant in protecting against the usual types of infection. • Eosinophils, however, are often produced in large numbers in people with parasitic infections, and they migrate into tissues diseased by parasites. • Although most parasites are too large to be phagocytized by eosinophils or any other phagocytic cells, eosinophils attach themselves to the parasites by way of special surface molecules and release substances that kill many of the parasites. • Eosinophils also have a special propensity to collect in tissues in which allergic reactions occur, such as in the peribronchial tissues of the lungs in people with asthma and in the skin after an allergic skin reaction. • This action is caused at least partly by the fact that many mast cells and basophils participate in allergic reactions. • The mast cells and basophils release an eosinophil chemotactic factor that causes eosinophils to migrate toward the inflamed allergic tissue. • The eosinophils are believed to detoxify some of the inflammationinducing substances released by the mast cells and basophils and probably also phagocytize and destroy allergen-antibody complexes, thus preventing excess spread of the local inflammatory process. Basophils • The basophils in the circulating blood are similar to the large tissue mast cells located immediately outside many of the capillaries in the body. • Both mast cells and basophils liberate heparin into the blood. Heparin is a substance that can prevent blood coagulation. • The mast cells and basophils also release histamine, as well as smaller quantities of bradykinin and serotonin. • It is mainly the mast cells in inflamed tissues that release these substances during inflammation. • The mast cells and basophils play an important role in some types of allergic reactions because the type of antibody that causes allergic reactions, immunoglobulin E (IgE), has a special propensity to become attached to mast cells and basophils. • Then, when the specific antigen for the specific IgE antibody subsequently reacts with the antibody, the resulting attachment of antigen to antibody causes the mast cell or basophil to release increased quantities of histamine, bradykinin, serotonin, heparin, and several lysosomal enzymes. • These substances cause local vascular and tissue reactions that mediate many, if not most, of the allergic manifestations. Leukopenia • A clinical condition known as leukopenia, in which the bone marrow produces very few WBCs, occasionally occurs. • This condition leaves the body unprotected against many bacteria and other agents that might invade the tissues. • Irradiation of the body by X-rays or gamma rays, or exposure to drugs and chemicals that contain benzene or anthracene nuclei, is likely to cause aplasia of the bone marrow. • Some common drugs such as chloramphenicol (an antibiotic), thiouracil (used to treat thyrotoxicosis), and even various barbiturate hypnotics on rare occasions cause leukopenia, thus setting off the entire infectious sequence of this disorder. Leukemias • Uncontrolled production of WBCs can be caused by cancerous mutation of a myelogenous or lymphogenous cell. • This process causes leukemia, which is usually characterized by greatly increased numbers of abnormal WBCs in the circulating blood. • There are two general types of leukemia, lymphocytic and myelogenous. • The lymphocytic leukemias are caused by the cancerous production of lymphoid cells, usually beginning in a lymph node or other lymphocytic tissue and spreading to other areas of the body. • The second type of leukemia, myelogenous leukemia, begins by cancerous production of young myelogenous cells in the bone marrow and then spreads throughout the body so that WBCs are produced in many extramedullary tissues—especially in the lymph nodes, spleen, and liver. Immunity • The human body has the ability to resist almost all types of organisms or toxins that tend to damage the tissues and organs. This capability is called immunity. • The human body can prevent the entry of pathogens or destroy them if they enter. Some mechanisms are quite general and protect against many types of pathogens, providing innate (nonspecific) immunity. • Other defense mechanisms are very precise, targeting specific pathogens and providing acquired immunity. Specialized lymphocytes that recognize foreign molecules (nonself antigens) in the body act against them. • Much of the immunity is acquired immunity that does not develop until after the body is first attacked by a bacterium, virus, or toxin; often, weeks or months are required for the immunity to develop. Innate Immunity An additional element of immunity that results from general processes, rather than from processes directed at specific disease organisms, is called innate immunity, which includes the following aspects: Mechanical Barriers Chemical Barriers Natural Killer (NK) Cells Inflammation Fever Phagocytosis Mechanical Barriers • The skin and mucous membranes lining the passageways of the respiratory, digestive, urinary, and reproductive systems create mechanical barriers that prevent the entrance of infectious agents. • As long as the skin and mucous membranes remain intact, many pathogens are unable to penetrate them. • Hair traps infectious agents associated with the skin and mucous membranes and sweat and mucus rinse away microorganisms. • These barriers provide a first line of defense. Chemical Barriers • Enzymes in body fluids provide a chemical barrier to pathogens. • Gastric juice, for example, contains the protein-splitting enzyme pepsin and has a low pH due to the presence of hydrochloric acid (HCl). • The combined effect of pepsin and HCl is lethal to many pathogens that enter the stomach. • Similarly, tears contain the enzyme lysozyme, which destroys certain bacteria in the eyes. • The accumulation of salt from perspiration also kills certain bacteria on the skin. • Lymphocytes and fibroblasts produce hormonelike peptides called interferons in response to viruses or tumor cells. • Once released from the virus-infected cell, interferon binds to receptors on uninfected cells, stimulating them to synthesize proteins that block the replication of a variety of viruses. • Thus, interferon’s effect is nonspecific. • Interferons also stimulate phagocytosis and enhance the activity of other cells that help resist infections and the growth of tumors. The Complement system is a group of proteins, in plasma and other body fluids, that interact in an expanding series of reactions or cascades. • Activation of complement stimulates inflammation, attracts phagocytes, and enhances phagocytosis. Natural Killer (NK) Cells • Natural killer (NK) cells are a small population of lymphocytes different from the lymphocytes that provide acquired defense mechanisms. • NK cells defend the body against various viruses and cancer cells by secreting cytolytic (“cell-cutting”) substances called perforins that lyse the cell membrane, destroying the infected cell. • NK cells also secrete chemicals that enhance inflammation. Inflammation • When tissue injury occurs, whether caused by bacteria, trauma, chemicals, heat, or any other phenomenon, multiple substances are released by the injured tissues and cause dramatic secondary changes in the surrounding uninjured tissues. This entire complex of tissue changes is called inflammation. Inflammation is characterized by the following: (1) vasodilation of the local blood vessels, with consequent increased local blood flow; (2) increased permeability of the capillaries, allowing leakage of large quantities of fluid into the interstitial spaces; (3) often, clotting of the fluid in the interstitial spaces because of increased amounts of fibrinogen and other proteins leaking from the capillaries; (4) migration of large numbers of granulocytes and monocytes into the tissue; and (5) swelling of the tissue cells. Some of the many tissue products that cause these reactions are histamine, bradykinin, serotonin, prostaglandins, several different reaction products of the complement system, reaction products of the blood clotting system, and multiple substances called lymphokines that are released by sensitized T cells. Inflammation produces localized redness, swelling, heat, and pain. • The redness is a result of blood vessel dilation that increases blood flow and volume in the affected tissues. • This effect, coupled with an increase in the permeability of nearby capillaries, swells tissues (edema). • The heat comes as blood enters from deeper body parts, which are generally warmer than the surface. • Pain results from stimulation of nearby pain receptors. • Local heat speeds up phagocytic activity. • In bacterial infections, the resulting mass of white blood cells, bacterial cells, and damaged tissue may form a thick fluid called pus. • Body fluids also collect in inflamed tissues. These fluids contain fibrinogen and other blood-clotting factors. Clotting forms a network of fibrin threads in the affected region. • Later, fibroblasts may arrive and secrete matrix components until the area is enclosed in a connective tissue sac. • This walling off of the infected area helps inhibit the spread of pathogens and toxins to adjacent tissues. Phagocytosis • Phagocytosis removes foreign particles from the lymph as it moves from the interstitial spaces to the bloodstream. • The blood’s most active phagocytic cells are neutrophils and monocytes. • Chemicals released from injured tissues attract these cells (chemotaxis). • Neutrophils engulf and digest smaller particles; monocytes phagocytize larger ones. • Monocytes that leave the bloodstream by diapedesis become macrophages (histiocytes), which may be free or fixed in various tissues. • The fixed macrophages, which can divide and produce new macrophages, are found in the lymph nodes, spleen, liver, and lungs. • Neutrophils, monocytes, and macrophages constitute the mononuclear phagocytic system (reticuloendothelial system). Fever • Fever is body temperature elevated above an individual’s normal temperature due to an elevated setpoint. • It is part of the innate defense because it makes the body inhospitable to certain pathogens. • Higher body temperature causes the liver and spleen to sequester iron, which reduces the level of iron in the blood. • Bacteria and fungi require iron for normal metabolism, so their growth and reproduction in a fever-ridden body slows and may cease. • Also, phagocytic cells attack more vigorously when the temperature rises. • For these reasons, low-grade fever of short duration may be a desired natural response, not a symptom to be treated aggressively with medications. Acquired (Adaptive) Immunity • In addition to its generalized innate immunity, the human body has the ability to develop extremely powerful specific immunity against individual invading agents such as lethal bacteria, viruses, toxins, and even foreign tissues from other animals. This ability is called acquired or adaptive immunity. • Acquired immunity is caused by a special immune system that forms antibodies and/or activated lymphocytes that attack and destroy the specific invading organism or toxin. • Acquired immunity can often bestow an extreme degree of protection. Lymphocyte Origins • During fetal development (before birth), red bone marrow releases unspecialized precursors to lymphocytes into the circulation. • About half of these cells reach the thymus, where they specialize into T lymphocytes or T cells. • Later, some of these T cells constitute 70–80 of the circulating lymphocytes in the blood. • Other T cells reside in lymphatic organs and are particularly abundant in the lymph nodes, thoracic duct, and white pulp of the spleen. • Other lymphocytes remain in the red bone marrow until they differentiate into B lymphocytes or B cells. • The blood distributes B cells, which constitute 20– 30%of circulating lymphocytes. • B cells settle in lymphatic organs along with T cells and are abundant in the lymph nodes, spleen, bone marrow, and intestinal lining. Basic Types Of Acquired Immunity— Humoral And Cell-mediated • Two basic but closely allied types of acquired immunity occur in the body. • B-Cell Immunity: In this type of immunity the body develops circulating antibodies, which are globulin molecules in the blood plasma capable of attacking the invading agent. (B lymphocytes produce the antibodies). • T-Cell Immunity: This type of immunity is achieved through the formation of large numbers of activated T lymphocytes, which are specifically crafted in the lymph nodes to destroy the foreign agent. • This type of immunity is called cell-mediated immunity or T-cell immunity because the activated lymphocytes are T lymphocytes. • Both the antibodies and activated lymphocytes are formed in the lymphoid tissues of the body. Antigens Initiate both Types Of Acquired Immunity • Because acquired immunity does not develop until after invasion by a foreign organism or toxin, it is clear that the body must have some mechanism for recognizing this invasion. • Each toxin or organism almost always contains one or more specific chemical compounds in its makeup that are different from all other compounds. • In general, these proteins or large polysaccharides initiate the acquired immunity; these substances are called antigens (antibody generators). Formation of antibodies and sensitized lymphocytes by a lymph node in response to antigens. This figure also shows the origin of thymic (T) and bursal (B) lymphocytes that, respectively, are responsible for the cell-mediated and humoral immune processes. T Cells and the Cellular Immune Response • A lymphocyte must be activated before it can respond to an antigen. • T cell activation requires the presence of processed fragments of antigen attached to the surface of another type of cell, called an antigenpresenting cell (accessory cell). • Macrophages, B cells, and several other cell types can be antigenpresenting cells. • T cell activation begins when a macrophage phagocytizes a bacterium, digesting it in its lysosomes. • Some bacterial antigens exit the lysosomes and move to the macrophage’s surface. • Here, they are displayed on the cell membrane near certain protein molecules that are part of a group of proteins called the major histocompatibility complex (MHC). • MHC antigens help T cells recognize that an antigen is foreign, not self. • Activated T cells interact directly with antigen-bearing cells. Such cell-tocell contact is called the cellular immune response, or cell-mediated immunity. • T cells (and some macrophages) also synthesize and secrete polypeptides called cytokines that enhance certain cellular responses to antigens. • For example, interleukin-1 and interleukin-2 stimulate the synthesis of several cytokines by other T cells. • In addition, interleukin-1 helps activate T cells, whereas interleukin-2 causes T cells to proliferate. • Other cytokines, called colony-stimulating factors (CSFs), stimulate leukocyte production in red bone marrow, and activate macrophages. • T cells may also secrete toxins that kill their antigen-bearing target cells, growth-inhibiting factors that prevent target cell growth, or interferon that inhibits the proliferation of viruses and tumor cells. Primary types of T cells: (1) T-helper cells; (2) cytotoxic T cells; and (3) regulatory T cells (also called suppressor T cells). (4) Memory T cells • A helper T cell becomes activated when its antigen receptor combines with the displayed foreign antigen. • Once activated, the helper T cell stimulates a B cell to produce antibodies that are specific for the displayed antigen. • Cytotoxic T cell recognizes and combine with nonself antigens that cancerous cells or virally infected cells display on their surfaces near certain MHC proteins. • Cytokines from helper T cells activate the cytotoxic T cell. • Next, the cytotoxic T cell proliferates, enlarging its clone of cells, which is a group of genetically identical cells that descend from a single, original cell. • Cytotoxic T cells then bind to the surfaces of antigenbearing cells, where they release perforin protein that cuts porelike openings, destroying these cells. • In this way, cytotoxic T cells continually monitor the body’s cells, recognizing and eliminating tumor cells and cells infected with viruses. • Cytotoxic T cells provide much of the immune system’s defense against HIV infection. Unfortunately, the virus eventually kills these cells. • Some T cells do not respond to a foreign antigen on first exposure but remain as memory cells. These memory cells immediately divide to yield more cytotoxic T cells and helper T cells upon subsequent exposure to the same antigen, often before symptoms arise. • Much less is known about the regulatory T cells than about the others, but they are capable of suppressing the functions of both cytotoxic and Thelper cells. • It is probable that the suppressor T-cell system plays an important role in limiting the ability of the immune system to attack a person’s own body tissues, called immune tolerance. B Cells and the Humoral Immune Response • A B cell may become activated when it encounters an antigen whose molecular shape fits the shape of its antigen receptors. • In response to the receptor antigen combination, the B cell may divide repeatedly, expanding its clone. • However, most of the time B cell activation requires T cell “help.” • When an activated helper T cell encounters a B cell already combined with a foreign antigen identical to the one that activated the helper T cell, the helper cell releases certain cytokines that stimulate the B cell to proliferate, enlarging its clone of antibody-producing cells. • The cytokines also attract macrophages and leukocytes into inflamed tissues and help keep them there. An activated B cell proliferates after stimulation by cytokines released from helper T cells. The B cell’s clone enlarges. Some cells of the clone give rise to antibody-secreting plasma cells and others to dormant memory cells. • Some members of the activated B cell’s clone differentiate further into memory cells. • Like memory T cells, these memory B cells respond rapidly to subsequent exposure to a specific antigen. • Other members of the activated B cell’s clone differentiate further into plasma cells, which produce and secrete large globular proteins called antibodies, or immunoglobulins, similar in structure to the antigen-receptor molecules on the original B cell’s surface. • Body fluids carry antibodies, which then react in various ways to destroy specific antigens or antigen-bearing particles. • This antibody-mediated immune response is called the humoral immune response (“humoral” refers to fluid). • Types of Antibodies • Antibodies (immunoglobulins) are soluble, globular proteins that constitute the gamma globulin fraction of plasma proteins. • Of the five major types of immunoglobulins, the most abundant are immunoglobulin G, immunoglobulin A, and immunoglobulin M. • Immunoglobulin G (IgG) is in plasma and tissue fluids and is particularly effective against bacteria, viruses, and toxins. It also activates complement. • Immunoglobulin A (IgA) is commonly found in exocrine gland secretions. It is in breast milk, tears, nasal fluid, gastric juice, intestinal juice, bile, and urine. • Immunoglobulin M (IgM) is a type of antibody present in plasma in response to contact with certain antigens in foods or bacteria. IgM also activates complement. • Immunoglobulin D (IgD) is found on the surfaces of most B cells, especially those of infants. IgD is important in activating B cells. • Immunoglobulin E (IgE) is in exocrine secretions with IgA. It is associated with allergic reactions. Antibody Actions In general, antibodies react to antigens in three ways: • Antibodies directly attack antigens, activate complement or stimulate localized changes (inflammation) that help prevent the spread of pathogens or cells bearing foreign antigens. • In a direct attack, antibodies combine with antigens, causing them to clump (agglutinate) or to form insoluble substances (precipitate). • Such actions make it easier for phagocytic cells to recognize and engulf the antigen-bearing agents and eliminate them. • In other instances, antibodies cover the toxic portions of antigen molecules and neutralize their effects. • However, under normal conditions, direct antibody attack is not as important as complement activation in protecting against infection. Complement System When certain IgG or IgM antibodies combine with antigens, they expose reactive sites on antibody molecules. This triggers a series of reactions, leading to activation of the complement proteins, which in turn produce a variety of effects. These include: • coating the antigen-antibody complexes (opsonization), • making the complexes more susceptible to phagocytosis; attracting macrophages and neutrophils into the region (chemotaxis); • rupturing membranes of foreign cells (lysis); • clumping antigen-bearing cells (agglutination); • and altering the molecular structure of viruses, making them harmless (neutralization) Immune Responses • Activation of B cells or T cells after first encountering the antigens for which they are specialized to react constitutes a primary immune response. During such a response, plasma cells release antibodies (IgM, followed by IgG) into the lymph. • The antibodies are transported to the blood and then throughout the body, where they help destroy antigen-bearing agents. • Production and release of antibodies continue for several weeks. • Following a primary immune response, some of the B cells produced during the proliferation of the clone remain dormant as memory cells. • If the identical antigen is encountered again, clones of these memory cells enlarge and can respond rapidly with IgG to the antigen to which they were previously sensitized. • These memory B cells, along with the memory T cells, produce a secondary immune response. • As a result of a primary immune response, detectable concentrations of antibodies usually appear in the blood plasma five to ten days after exposure to antigens. • If the identical antigen is encountered later in life, a secondary immune response may produce the same antibodies within a day or two. • Although newly formed antibodies may persist in the body for only a few months or years, memory cells live much longer. A primary immune response occurs after the first exposure to an antigen. A secondary immune response occurs after subsequent exposure(s) to the same antigen. The break in the timeline represents a time lapse between exposure(s) to the antigen, when the time to develop antibodies begins once again. Practical Classification of Immunity • Adaptive, also known as acquired, immunity can arise in response to natural events or be induced artificially by injection. Both naturally and artificially acquired immunities can be either active or passive. • Active immunity results when the person produces an immune response (including memory cells) to the antigen; it is long-lasting. Passive immunity occurs when a person receives antibodies produced by another individual. • Because the person does not produce an immune response, passive immunity is short-term, and the individual will be susceptible upon exposure to the antigen at some later date. • Naturally acquired active immunity occurs when a person exposed to a pathogen develops a disease. • Resistance to that pathogen is the result of a primary immune response. • A vaccine is a preparation that produces another type of active immunity. A vaccine might consist of bacteria or viruses that have been killed or weakened so that they cannot cause a serious infection, or only molecules unique to the pathogens. • A vaccine might also be a toxoid, which is a toxin from an infectious organism that has been chemically altered to destroy its dangerous effects. Whatever its composition, a vaccine includes the antigens that stimulate a primary immune response but do not produce the severe symptoms of the disease. A vaccine causes a person to develop artificially acquired active immunity. • A person who has been exposed to infection may require protection against a disease-causing microorganism before active immunity has had the time to develop. • An injection of antiserum (antibodies) may help. These antibodies may be obtained by separating gamma globulins from the plasma of persons who have already developed immunity against the particular disease. • A gamma globulin injection provides artificially acquired passive immunity. • During pregnancy, certain antibodies (IgG) pass from the maternal blood into the fetal bloodstream. As a result, the fetus acquires limited immunity against pathogens that the pregnant woman has developed active immunities against. The fetus thus has naturally acquired passive immunity, which may last for six months to a year after birth. Autoimmunity • The immune system can turn against itself, and become unable to distinguish a particular selfantigen from a nonself antigen, producing autoantibodies and cytotoxic T cells that attack and damage certain tissues and organs. • This reaction against self is called autoimmunity. The specific nature of an autoimmune disorder reflects the cell types that are the target of the immune attack. • Type 1 (insulin dependent) diabetes mellitus, rheumatoid arthritis, and systemic lupus erythematosus are autoimmune disorders. • About 5% of the population has an autoimmune disorder. Why might the immune system attack body tissues? • Perhaps a virus, while replicating inside a human cell, takes proteins from the host cell’s surface and incorporates them onto its own surface. When the immune system “learns” the surface of the virus to destroy it, it also learns to attack the human cells that normally bear the particular protein. • Another explanation of autoimmunity is that somehow T cells never learn to distinguish self from nonself. • A third possible route of autoimmunity is when a nonself antigen coincidentally resembles a selfantigen.