4MBBS101 Lecture 5 Properties of Enzymes - Enzyme kinetics PDF
Document Details
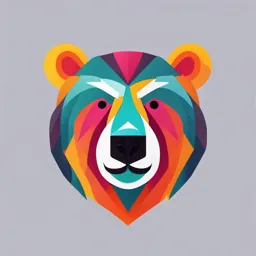
Uploaded by StableEpilogue
King's College London
Dr Gian De Nicola
Tags
Summary
These are lecture notes from King's College London on the properties of enzymes and enzyme kinetics. The document covers learning outcomes, enzyme structure, definitions and properties of enzymes. It discusses different types of enzyme inhibition and regulation mechanisms.
Full Transcript
4MBBS101– 5: Properties of Enzymes; Enzyme kinetics Dr Gian De Nicola [email protected] Learning outcomes Review basics of enzymology: concept of catalysis, sub...
4MBBS101– 5: Properties of Enzymes; Enzyme kinetics Dr Gian De Nicola [email protected] Learning outcomes Review basics of enzymology: concept of catalysis, substrate specificity; effects of pH and temperature on the rate of an enzyme catalysed reaction Describe how the rate of an enzyme reaction depends on the concentration of the substrate(s) and how this is described by the Michaelis-Menten equation Define the terms: initial velocity, Km, Vmax and explain how these values may be determined experimentally Explain the different effects of competitive and non-competitive inhibitors on enzyme kinetic parameters Explain with examples the terms reversible inhibition and irreversible inhibition Explain the concepts of cooperativity and positive and negative allosteric modulation Summarise some of the important mechanisms for regulation of enzyme activity Enzyme structure Enzymes are proteins i.e. they are composed of one or more polypeptide chains folded into a complex 3-dimensional shape. Enzyme structure is stabilized by many weak bonds e.g. H-bonds, electrostatic salt bridges, Van der Waals and hydrophobic interactions. Catalase has four identical subunits, each with catalytic activity Definition/properties Enzymes are: Biological catalysts that speed up the rate of a reaction, without altering the final equilibrium between reactants and products. Extremely efficient, e.g. the enzyme catalase catalyses the breakdown of its substrate H2 O2 to water at a rate 1014 times faster than the uncatalysed reaction at 30C. 4 Effect of enzyme on activation energy of a reaction 5 Enzymes active site: Lock and key theory How do enzymes lower the activation energy? Emil Fisher proposed (1884) that enzymes are complementary to their substrate, like a lock and key, to explain the high specificity of enzymes This concept influenced greatly the development of biochemistry but it is misleading Why? What are the implications of the usefulness of the lock and key theory? Induced fit theory Daniel Koshland, in 1958, suggested some modification to the lock and key theory Enzymes will undergo conformational changes upon substrate binding, induced by weak interactions with the substrate itself These changes can affect residues in the active site The “induced fit” serves to bring specific functional groups within the enzyme in the proper position to catalyse the reaction The new enzyme conformation has enhanced catalytic properties What is a transition state? An unstable, high-energy intermediate in a chemical reaction: stabilising the transition state is one way that enzymes can speed up a reaction. Why complementarity of enzymes to transition state is necessary? Let’s consider a hypothetical “Stickase” which catalyzes a cleavage of a metal stick a) In the uncatalyzed reaction the bar must bend first to a transition state before being broken. High activation energy reaction proceeds slowly b) When the enzyme is complementary to the bar the rate would not improve as the enzyme stabilizes the structure of the stick. ES complex will be in an energetic minimum difficult to escape c) The enzyme is complementary to the transition state B Substrate Specificity (1) Enzymes will usually: – Catalyse only one type of reaction (e.g. alcohol dehydrogenase will oxidise primary alcohols to aldehydes) – And will act on only a few related molecules (‘group specificity’ e.g. alcohol dehydrogenase will oxidise methanol, ethanol and propanol). A few enzymes are so specific they will act on one substrate. Substrate Specificity (2) If a natural compound can exist in two stereoisomer forms e.g. D-glucose and L-glucose, the enzyme will usually act only on one isomer. Specificity is determined by a groove or cleft of defined shape - the ‘active site’ into which only the substrate of the correct shape and charge can fit. Enzyme Specificity - classification Specificity of enzymes has led to a systematic classification scheme, established by the I.U.B. Commission on Enzymes, Enzymes are divided into 6 main classes according to the type of reaction they catalyse – e.g. Class 1, oxidoreductases, contains the enzymes catalase and alcohol dehydrogenase. Six classes are then further divided into subgroups according to their substrate or source. Each enzyme is identified by its own individual 4-digit number Classification of enzymes E.g. tripeptide aminopeptidase Cleaves amino terminal amino acid from a polypeptide hydrolases 3. 4. 11. 4 Cleaves amino terminal amino acid from a tripeptide hydrolases that act on peptide bonds Classification of enzymes CLASS TYPE OF REACTION EXAMPLE 1. Oxidoreductases To catalyze oxidation/reduction reactions; transfer of lactate dehydrogenase H and O atoms or electrons from one substance to another 2. Transferases Transfer of a functional group from one substance to Alanine amino transferase another. The group may be methyl-, acyl-, amino- or phosphate group 3. Hydrolases Formation of two products from a substrate Trypsin by hydrolysis 4. Lyases Non-hydrolytic addition or removal of groups from Aldolase substrates. C-C, C-N, C-O or C-S bonds may be cleaved 5. Isomerases Intramolecule rearrangement, Phosphoglucose isomerase i.e. isomerization changes within a single molecule 6. Ligases Join together two molecules by synthesis of new C- DNA ligase O, C-S, C-N or C-C bonds with simultaneous breakdown of ATP Oxidoreductases catalyze oxidation/reduction reactions; transfer of H and O atoms or electrons from one substance to another Example - Lactate Dehydrogenase O O- O O- C lactate C dehydrogenase C O + NADH + H+ HO CH + NAD + CH3 CH3 pyruvate L-lactate Transferases catalyze the transfer of functional groups from one substance to another Example - Alanine aminotransferase O O- O O- C C H2N CH O O- O O- C O C alanine C CH2 aminotransferase C O CH2 + H2 N CH + CH2 CH2 CH3 CH3 C pyruvate L-alanine C - O O O O- glutamate -ketoglutarate (2 oxoglutarate) Hydrolases catalyze formation of two products from a substrate by hydrolysis (splitting using water) Example - Trypsin NH 2(CH 2) 4 H O H N C C O - C N C H O NH 2(CH 2) 4 CH 3 H H H O H H O H trypsin Gl y - L y s C C N C C N C N C C N C + H2 O + H H O O CH 3 H H H CH(CH ) H 32 O + C Gl y - L y s - V a l - A l a H 3N C C N C H O H CH(CH ) 32 V al- Ala Lyases catalyze non-hydrolytic addition or removal of groups from substrates. C-C, C-N, C-O or C-S bonds may be cleaved Example - aldolase Isomerases catalyze isomerization changes within a single molecule Example – Phosphoglucose isomerase (phosphohexose isomerase) Ligases join together two molecules by synthesis of new C-O, C-S, C-N or C-C bonds with simultaneous breakdown of ATP Example - DNA ligase Properties of enzymes: effects of temperature Enzyme structure is stabilized by many weak bonds e.g. H-bonds, electrostatic salt bridges, Van der Waals and hydrophobic interactions. These weak bonds are easily broken e.g. by heating the protein, giving rise to a disorganised or tangled structure in which the enzyme no longer has any catalytic activity (inactive, ‘denatured’ state). This property makes enzymes very sensitive to changes in their environment. Effect of pH on enzyme-catalysed reactions Changes in pH can have a number of effects on the rate of enzyme-catalysed reactions: Direct effects [H+] or [OH-] appear in the rate equations Substrate effects Changes in ionisation state of the substrate leading to additional acid/base catalysis. Changes leading to altered binding to the enzyme and hence a change in Km. Effects on the enzyme Unfolding of the enzyme leading to complete inactivation. Changes in ionization state of active site residues leading to change in Km (substrate binding) or Vmax (involved in reaction). Effect of pH on enzyme reactions (1) Typical enzyme (2) Pepsin – functions in stomach (acidic) Enzyme Kinetics The study of the rate of an enzyme catalysed reaction – how that rate varies with different substrate concentrations – effects of inhibitors Reaction rate (v) D[product]/D time = rate D[substrate]/D time = rate [Sustrate] [Product] Time The reaction rate is the increase in the amount of product formed per unit time. Alternatively, the reaction rate can be measured as decrease in the amount of substrate per unit time Many enzymes display saturation ‘hyperbolic’ kinetics At low substrate concentration, the reaction Reaction Rate Enzyme catalyzed reaction rate is directly proportional to the substrate concentration At high substrate concentration, the reaction rate is independent of substrate concentration. [Substrate] Michaelis-Menten reaction model k1 k2 E + S ⇌ ES → E + P k-1 Where: S = substrate E = enzyme ES = enzyme-substrate complex k1, k-1 and k2 = rate constants Three assumptions [S] >> [E] so that the amount of substrate bound by enzyme at any one time is small. Initial velocities used, concentration of product small and back reaction of P to S can be ignored k1 k2 E + S ⇌ ES ⇌ E + P X k-1 [ES] does not change with time (steady-state); formation of ES is = to breakdown of ES (to E+ S and E+ P). d ES = 0 = k1 E S − k −1 ES − k 2 ES dt Michaelis-Menten equation 𝑉 max[ 𝑆] Leonor Maud 𝑉0 = Michaelis Menten 𝐾𝑚 + [𝑆] Vmax V0 = initial reaction velocity, measured as soon as enzyme and substrate are mixed. Reaction velocity (V0) Vmax = maximal velocity of an enzyme Vmax/2 catalysed reaction i.e. when all the enzyme active sites are fully saturated with substrate. Km = Michaelis constant Km = (k-1 + k2)/k1 Substrate concentration [S] [S] = substrate concentration Special relationship between Km and [S] when V0= 0.5Vmax 𝑉 max.[ 𝑆] 𝑉0 = 𝐾𝑚 + [𝑆] 𝑉 max.[ 𝑆] If V0=0.5 Vmax 0.5 𝑉 max = 𝐾𝑚 + [𝑆] Multiply by Km+[S] 0.5 𝑉 max. 𝐾𝑚 + [𝑆] = 𝑉 max.[ 𝑆] 𝑉 max. [ 𝑆] Divide by 0.5 Vmax 𝐾𝑚 + [𝑆] = 0.5 𝑉max Simplify Vmax/0.5 Vmax 𝐾𝑚 + [𝑆] = 2. [𝑆] Subtract [S] from both sides 𝑲𝒎 = [𝑺] Km and enzyme substrate affinity k1 k2 E + S ⇌ ES → E + P k-1 𝑘2 + 𝑘 − 1 𝐾𝑚 = 𝑘1 𝑘−1 Where k2