Cell Injury 1: Cell Responses to Stress and Biochemical Mechanisms of Cell Injury PDF
Document Details
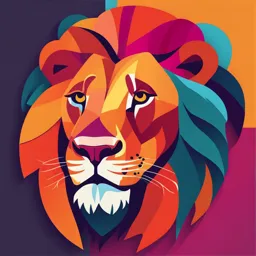
Uploaded by HonorableTsavorite
MUSC
Debra Hazen-Martin, PhD
Tags
Summary
This document outlines cell injury, covering cellular adaptations, mechanisms of stress and injury, including causes and key biochemical events. It also details the role of calcium homeostasis and free radicals in cell injury.
Full Transcript
Cell Injury 1 Debra Hazen-Martin, PhD Office: 792-2906 Email: [email protected] Cell Injury 1: Cell Responses to Stress and Biochemical Mechanisms of Cell Injury Outline: I. Overview A. Introduction to Cell Injury and Adaptive Changes B. Terms C. Causes of Cell Stress II. Cellular Adaptations A....
Cell Injury 1 Debra Hazen-Martin, PhD Office: 792-2906 Email: [email protected] Cell Injury 1: Cell Responses to Stress and Biochemical Mechanisms of Cell Injury Outline: I. Overview A. Introduction to Cell Injury and Adaptive Changes B. Terms C. Causes of Cell Stress II. Cellular Adaptations A. Changes in Cell Type, Number and Morphology B. Mechanisms and Examples C. Intracellular Accumulations III. Mechanisms of Cell Injury A. General Principles and Cellular Targets B. 3 Key Biochemical Events Suggested Reading: Robbins Basic Pathology by Kumar, Abbas, and Aster Chapter 2, pages 31-33, 48-52 Objectives: 1) Define and apply the terms etiology, pathogenesis, and morphologic change. 2) Describe adaptive changes in cell number, size, and/or type as it applies to atrophy, hypertrophy, hyperplasia, and metaplasia. 3) Recognize abnormal accumulations of endogenous and/or exogenous substances in cells and begin to appreciate the cellular mechanisms and processes that account for their presence. 4) List the possible causes and/or conditions that lead to cell stress and injury. 5) Describe an initiator and mechanisms of atrophy, hypertrophy, hyperplasia, and metaplasia. 6) Describe 4 general principles (presented in the lecture) that underlie our understanding of the steps in cell injury and pathogenesis. 7) Describe 3 key biochemical events observed during most instances of cell injury. 8) Describe the downstream effects of dysregulation of intracellular Ca++ levels that lead to additional injury of various cellular components. 9) Describe mechanisms for the generation of ATP (aerobic/anaerobic). 1 Cell Injury 1 10) Describe how oxygen-derived free radicals are formed and controlled in health. 11) Describe the targets and mechanisms of free radical injury in cells. 12) Describe factors that might lead to accumulation of proteins, lipids, and/or glycogen in stressed cells. I. Overview: A. Introduction: Cells must be able to make structural and functional adjustments in response to the constant stress of their environment. Their adaptive success determines whether stress leads to reversible or irreversible injury and, in some cases, death. In this lecture you will learn the causes of cell stress that lead to adaptive cellular changes and the mechanisms that cause injury. Key questions that you should hope to answer after Cell Injury lectures 1/2 are the following: 2 Cell Injury 1 B. Terms: As you begin your study of pathology you should become familiar with the following terms: C. Causes of Cell Stress: 1. Hypoxia – Oxygen deficiency, caused by ischemic events (stroke), inability to oxygenate blood (respiratory failure), loss of ability to carry oxygen in blood (anemia). 2. Chemicals/drugs – by direct injury of a cell compartment or metabolic event or by exposure of toxic metabolites or byproducts of the agent. 3. Microbiologic agents – Infection or exposure to pathogens or their products. 4. Immunologic events – immune reactions (anaphylaxis) or complexes (autoimmune response). 5. Genetic defects/damage – abnormal chromosome (trisomy 21) affecting many systems or a single point mutation that may alter a single protein. 6. Nutritional – dietary deficiencies and/or excesses. 7. Aging – genetically programmed or progressive wear and tear to cells. 3 Cell Injury 1 II. Cellular Adaptations A. Changes in Cell Type, Number, and Morphology: Cells may respond to pathological or physiological stresses by changing size, mitotic rate, or state of differentiation to avoid injury. Physiologic adaptations are usually mediated by changes in hormones, endogenous chemical mediators, or neurotransmitter stimulation. Pathological adaptations result from exposure to stress or agents that would injure the cell. The cell modulates it’s form and function to allow survival. While we consider hypertrophy, atrophy, and hyperplasia at the single cell level, it is with the understanding that the processes can impact organ size as well. Atrophy is the decrease in cell size. This response can lead to a reduction in organ size if enough cells atrophy. Fig Hypertrophy is the increase in cell size. This response can lead to an increase in organ size if enough cells of an organ are affected. Hyperplasia is due to an increase in mitosis resulting in a greater number of cells. Hyperplasia can also contribute to an increased organ size. Metaplasia is not shown on this diagram. 4 Cell Injury 1 B. Mechanism and Examples: 1. Atrophy may be caused by a decreased work load, decreased blood supply to an organ, alteration in nutritional status, loss of hormonal stimulation, loss of nerve supply or aging in general. Example: With age there is atrophy of the cortical cells of the brain leading to gross shrinkage of the gyri with wider sulci. At the cellular level, a reduction in size is caused by decreased synthetic mechanisms coupled with increased degradation. Ubiquitin ligases are activated and, in turn, initiate the ubiquitin-proteasome pathway leading to targeting of proteins for degradation. It is important to remember that these cells are not dead. Fig 2. Hypertrophy may be due to increased physical or mechanical demand or hormonal stimulation. At the cell level there is activation of synthetic pathways. Examples: Pathologic hypertrophy of the heart is illustrated by the increased cardiac muscle cell size in the left ventricle of the heart in hypertension. Both mechanical stress and trophic factors result in activation of genes that result in production of structural proteins (myofilaments) that increase cardiac muscle fiber size. The physiologic hypertrophy seen in the uterus during pregnancy also illustrates hormone induced increases in the size of smooth muscle cells. It is important to acknowledge that the estrogen causing cellular hypertrophy will also cause smooth muscle cell hyperplasia in the gravid uterus. 5 Cell Injury 1 3. Hyperplasia may result from growth factor stimulation or altered hormone levels in cell populations capable of reentering the cell cycle. Examples: Compensatory hyperplasia occurs in the remaining donor kidney following unilateral nephrectomy and in the remaining liver tissue following partial hepatectomy. Pathologic hyperplasia may also result from a change or increase in hormonal stimulation as in benign prostatic hyperplasia in the aging male. It is important to note that the presence of hyperplasia in the periurethral areas of the prostate (nodular hyperplasia) leads to the overall increase in the size of the organ (hypertrophy) and urinary retention. 4. Metaplasia is a change in the state of differentiation of a cell in response to a stress, most commonly an irritatant. In response to a potentially damaging irritant, growth factors signal to reserve stem cells to produce a population of cells better suited to handle the stress. Example: Respiratory epithelium that is chronically irritated by chemicals in tobacco smoke may respond by metaplastic change to a stratified squamous type of cell. With the change, comes loss of function. Particle-trapping mucous is not transported up and out of the respiratory airways. 6 Cell Injury 1 Metaplastic change can also involve a conversion from squamous epithelium to columnar epithelium. Example: Esophageal reflux disease may lead to replacement of normal stratified squamous epithelium of the lower esophagus with a columnar epithelial cell type with characteristics of stomach and possibly intestinal mucosa. ER: SSE -> CE This disease process is termed Barrett’s esophagus. Metaplasia may be a precursor to neoplasia. The same factors that stimulate stem cells to initiate metaplasia may also stimulate malignant transformation of epithelial cells. Barret's : SSE -> ICE 7 Cell Injury 1 C. Intercellular Accumulations: In response to stress, cells may accumulate substances that may be normal constituents of the cell (endogenous) or abnormal substances (either endogenous or exogenous). The substance may be harmless or may result in injury. Accumulations of normal endogenous material may occur when that substance is produced by the cell at normal or greater than normal rates where the rate of removal is inadequate to prevent accumulation. Fatty change of the liver and protein accumulations in proximal tubule cells of the kidney are examples of this situation. Both normal and abnormal endogenous substances may accumulate if the cell has defects in the metabolic pathway necessary to process the substance as in the lysosomal storage diseases. Certain exogenous substances accumulate in cells because there simply is no biological mechanism for their removal. This is the case of carbon in the lung and associated lymph nodes. 8 Cell Injury 1 Accumulations of lipids: Fatty change of the liver involves accumulation of triglycerides in hepatocytes due to blockage of any of the steps in triglyceride metabolism. xter(4) Insults that accomplish this include starvation, anoxia, chemicals, and alcohol. Lipids accumulate as small vesicles surrounding the nucleus and then coalesce to form large intracellular lipid vacuoles that displace the hepatocyte nucleus and may cause rupture of the cell. Fats may accumulate in any cell that metabolizes triglycerides if any step in the metabolic pathway is interrupted. Anoxia and alcohol may prevent oxidation of fatty acids to ketone bodies. Starvation liberates fatty acids from peripheral tissues causes greater concentration in the liver. A toxin such as carbon tetrachloride causes reduction in apoprotein synthesis necessary to form lipoproteins for transport out of the liver. All of the above cause triglycerides to accumulate. Cholesterol - In the intimal thickenings of vessels, macrophages and smooth muscle cells filled with lipid (foam cells) accumulate to contribute to mature atherosclerotic plaques . When the lipid containing cells die, the lipid collects in the extracellular space to form the cholesterol core of the atheroma. Needle-like profiles in fixed histological sections indicate the presence of lipid crystals. 9 Cell Injury 1 Accumulations of Protein –Proteins accumulate less commonly in cells. One example includes the proximal tubule cell of the kidney in patients with nephrotic syndrome. In this disease, greater than normal amounts of proteins escape in the glomerular filtrate which flows past the proximal tubule cells. Proximal tubule cells normally function to retrieve small proteins from the urine. In this disease they fill with eosinophilic droplets as they attempt to recapture the excess protein by reabsorption. Glycogen accumulation - Glycogen may accumulate in a number of cell types in diabetic individuals due to altered glucose or metabolism. In addition there are specific genetic defects resulting in a defective enzyme involved in glucose or glycogen metabolism. A defect in glucose6-phosphatase results in accumulation of glycogen in the liver and hepatomegaly. 10 Cell Injury 1 Pigment accumulation – Pigments derived from both exogenous and endogenous sources may accumulate in cells and tissues. Two types The endogenous pigments may be derived from hemoglobin by-products. Breakdown products of lipid peroxidation collects in normal cells results in collection of residue. One example of accumulation of an exogenous pigment is the accumulation of carbon from environmental pollutants in the lung. Macrophages transport carbon to tracheobronchial lymph nodes via lymphatic vessels. The carbon remains at these sites and is called anthracosis leading to various lung diseases. (I promise this really is from a text book!) Another exogenous pigment that may be stored is tattoo ink which is injected into the dermis. There is no biological mechanism for the removal of this substance leading to the permanent nature of tattoos. 11 Cell Injury 1 Two endogenous pigments derived from the normal breakdown of hemoglobin from aging or damaged red blood cells can accumulate in cells and tissues. Bilirubin is the pigmented end product of the degradation of hemoglobin from red blood cells. Bilirubin is taken up by the liver and is processed to bile and then excreted by the liver. If the liver fails to take up, process, or transport bilirubin or its products, the bilirubin will collect in tissues and remain in circulation. When concentrations of bilirubin in the blood exceed 2.0 mg/dL the condition of jaundice is noticed due to bilirubin’s ability to discolor tissues including skin and the sclera of the eyeball. Elevations in bilirubin are due to liver dysfunction or severe hemolysis. Dubin-Johnson disease involves a defect in the hepatic excretion of bilirubin. Bilirubin accumulates in the hepatocytes of these individuals. Hemosiderin is a hemoglobin-derived, ironcontaining pigment. The brown granules that accumulate in macrophages and parenchymal cells are the ferritin micelles. Local excesses of hemosiderin are found in focal hemorrhage in a bruise or in the congested lungs of heart failure patients. Systemic excess in multiple organs are due to massive doses of dietary iron or impaired iron usage may cause hemosiderosis and extreme iron overload is found in hemochromatosis along with organ dysfunction. Prussian blue is a histochemical stain that combines with iron to form a deep blue color. It can be used in gross or histological specimens to indicate iron accumulation. The pigment is normally golden brown in color. 12 Cell Injury 1 Lipofuscin is known as the “wear and tear” pigment that accumulates with age or atrophy. This pigment is the insoluble product of the cell’s lifetime of free radical injury and other insults. The granules of pigment are lipids and phospholipids complexed to protein. These accumulations are not injurious to the cell. III. Mechanisms of Cell Injury: Continued stress may exceed a cell’s adaptive capacity resulting in cell injury that leads to altered function. Cell injury may be reversible or irreversible. Identifying the precise mechanisms involved in cell injury is not always as easy as explaining that a particular substance such as cyanide inactivates a specific enzyme to stop ATP production. However, even after acknowledging the complicated factors listed in the above slide, some generalizations may be made. 13 Cell Injury 1 A. 4 General Principles 1. Example: Thermal injury would affect many cell and tissue types. Yet, the extent of the injury would be determined by the actual temperature and long the cell was exposed. 2. Example: Identical conditions such as loss of oxygen to a tissue for 5 minutes may have very different impacts on tissue of the central nervous system versus skeletal muscle of the lower limb. This points to different biochemical mechanisms in different tissues. 3. Specific agents may initially target a particular subcellular compartment most directly. Loss of function within that compartment tends to lead to damage in additional compartments. 4. Each of the 3 key biochemical events may lead to damage in one or multiple compartments of the cell. Determining the initial (primary) point of injury and loss of function can be difficult due to secondary effects. 14 Cell Injury 1 B. Key Biochemical Events 1. Loss of calcium homeostasis: The cell utilizes Ca++, Mg++-ATPase to maintain intracellular calcium levels that are much lower (0.1uM) than that of the extracellular environment (1.3mM). Mitochondrial and endoplasmic reticulum calcium stores also help to stabilize the lower cytosolic calcium levels. When membranes are damaged, extracellular calcium enters the cytosol resulting in release of both the mitochondrial and ER stores of calcium as well. The elevated cytosolic calcium then activates a number of enzymes that damage both protein and lipid membrane components, damage DNA, and deplete cellular ATP. Depletion of ATP then shuts down the membrane Na+, K+- ATPase that drives transport of sodium from the cell. With increased concentrations of intracellular sodium, water enters the cell and selective membrane permeability is lost. The entry of water is referred to as hydropic change. 2. Loss of ATP: ATP is required for an array of normal cellular functions including protein synthesis, membrane transport and lipid turnover. ATP may be depleted at abnormally rapid rates by increased ATPase activity in the presence of high cytosolic calcium levels (described above). In addition, loss of oxygen will lead to lowered ATP production in mitochondria by the process of oxidative phosphorylation of ADP. Anaerobic production of ATP may occur in the absence of oxygen by glycolytic pathways in cells that store glycogen or have continued access to circulating glucose. 15 Cell Injury 1 Aerobic production of ATP: The mitochondria receive pyruvate (a product of the breakdown of glucose in the cytosol). Pyruvate is oxidized to acetyl CoA and finally to CO2 and H2O by the citric acid cycle. This also produces FADH2 and NADH which at the inner mitochondrial membrane provide the protons that are pumped via the electron transport chain. The proton gradient formed provides the energy for the generation of ATP (by ATP synthase). This system, with normal oxygen levels, provides the optimal amount of ATP (36). Any event that decreases oxygen, substrate (glucose), integrity of the mitochondrial membrane and/or activity of the electron transport chain proteins will adversely impact ATP production. 1. Decrease O2 substrate(glu) 2. Mit membrane integrity 3. ETC activity Anaerobic production of ATP by glycolysis: Cells with glycogen stores or sustained access to circulating glucose can revert to temporary dependance on the glycolytic pathway of ATP production under anaerobic conditions. Elevated AMP and low oxygen increase the activity of phosphofructokinase and lactate dehydrogenase to convert glucose to pyruvate and pyruvate to lactic acid. This provides only 2 ATP’s (better than nothing). But, it does have the liability of decreasing cellular pH as lactic acid accumulates. The lowered pH can also damage cell components and enzyme activity. 16 Cell Injury 1 3. Free radical formation and accumulation: Free radicals are atoms or molecules with a single unpaired electron in the outer orbit. Frequently derived from oxygen, they are unstable and very reactive and will combine readily with both inorganic and organic molecules. Free radicals are formed in the following ways: a) Normal oxidation- reduction reactions in the cell (respiration): The addition of 4 electrons to molecular oxygen reduces it to water. If the electron additions occur one at a time, toxic intermediate species (superoxide anion, hydrogen peroxide, and hydroxyl radical) are formed. Hydroxyl radical is the most toxic of these free radical species. Cells are normally protected from free radicals by the following enzymes: SOD converts SO to hydrogen peroxide. Catalase converts hydrogen peroxide to water and oxygen. Glutathione peroxidase coverts hydrogen peroxide to water. Antioxidants (vitamin E, A, C, beta-carotene) also block formation of free radicals within membranes. b) Intracellular oxidases can generate free radicals in the presence of transition metals (copper and iron). In the presence of superoxide anion, ferric iron (Fe+++) will accept an electron to form molecular oxygen and ferrous iron (Fe++). The reduced ferrous iron and hydrogen peroxide produce the hydroxyl radical, hydroxyl ion and ferric iron. This activity is contained/controlled by normal binding of the metals to transport proteins (transferrin, ferritin, ex.) 17 Cell Injury 1 c) Additional mechanisms for free radical formation There are 3 cellular targets for free radical injury: 1) Single strand breaks in DNA may contribute to aging, malignant transformation or cell death. 2) Protein cross-linking results in conformational changes that alter enzyme activity and enhance protein degradation. 3) Lipid peroxidation of membranes (most important). Free radicals attack double bonds in polyunsaturated fatty acids of membrane lipids (123°angles) by removing H from the carbon adjacent to the double bond. This straightens the 123° bend and breakage occurs. The breakage products are peroxides and act as free radicals as well. 18