Chemical Bonds Chemistry PDF
Document Details
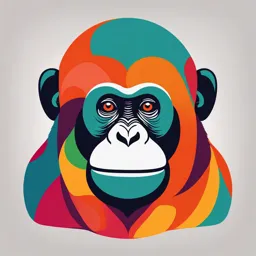
Uploaded by AdoredExponential
University of Science and Technology Sana'a
Dr. Saeed Mohammed Ismaeel
Tags
Summary
This document explains chemical bonds, including the octet rule, ionic bonding, covalent bonding, and electronegativity. It provides examples and solutions to illustrate the concepts. The material is suitable for an undergraduate chemistry course.
Full Transcript
Chemical Bonds Chemistry Dr. Saeed Mohammed Ismaeel CHEMICAL BONDS Octet rule When undergoing chemical reaction, atoms of Group 1A–7A elements tend to gain, lose, or share sufficient electrons to achieve an electi...
Chemical Bonds Chemistry Dr. Saeed Mohammed Ismaeel CHEMICAL BONDS Octet rule When undergoing chemical reaction, atoms of Group 1A–7A elements tend to gain, lose, or share sufficient electrons to achieve an election configuration having eight valence electrons Anion : An ion with a negative electric charge. Cation : An ion with a positive electric charge E X A M P L E :1 Show how the following chemical changes obey the octet rule: (a) A sodium atom loses an electron to form a sodium ion, Na +. (b) A chlorine atom gains an electron to form a chloride ion, Cl–. Solution To see how each chemical change follows the octet rule, first write the condensed ground- state electron configuration of the atom involved in the chemical change and the ion it forms, and then compare them. (a) The condensed ground-state electron configurations for Na and Na+ are A sodium atom has one electron in its valence shell. The loss of this one valence electron changes the sodium atom to a sodium ion, Na+, which has a complete octet of electrons in its valence shell and the same electron configuration as neon, the noble gas nearest to it in atomic number. We can write this chemical change using Lewis dot structures: (b) The condensed ground-state electron configurations for Cl and Cl– are as: 1 1 / 96 Chemical Bonds Chemistry Dr. Saeed Mohammed Ismaeel A chlorine atom has seven electrons in its valence shell. The gain of one electron changes the chlorine atom to a chloride ion, Cl–, which has a complete octet of electrons in its valence shell and the same electron configuration as argon, the noble gas nearest to it in atomic number. We can write this chemical change using Lewis dot structures: The octet rule gives us a good way to understand why Group 1A–7A elements form the ions that they do. It is not perfect, however, for the following two reasons: 1. Ions of period 1 and 2 elements with charges greater than 12 are unstable. Boron, for example, has three valence electrons. If it lost these three electrons, it would become B3+ and have a complete outer shell like that of helium. It seems, however, that this is far too large a charge for an ion of this period 2 element; consequently, this ion is not found in stable compounds. By the same reasoning, carbon does not lose its four valence electrons to become C4+, nor does it gain four valence electrons to become C4-. Either of these changes would place too great a charge on this period 2 element. 2. The octet rule does not apply to Group 1B–7B elements (the transition elements), most of which form ions with two or more different positive charges. Copper, for example, can lose one valence electron to form Cu+;alternatively, it can lose two valence electrons to form Cu2+. It is important to understand that there are enormous differences between the properties of an atom and those of its ion(s). Atoms and their ions are completely different chemical species and have completely different chemical and physical properties. Consider, for example, sodium and chlorine. Sodium is a soft metal made of sodium atoms that react violently with water. Chlorine atoms are very unstable and even more reactive than sodium atoms. Both sodium and chlorine are poisonous. NaCl, common table salt, is made up of sodium ions and chloride ions. These two ions are quite stable and unreactive. Neither sodium ions nor chloride ions react with water at all. Because atoms and their ions are different chemical species, we must be careful to distinguish one from the other. Consider the drug commonly known as “lithium,” which is used to treat manic depression. The element lithium, like sodium, is a soft metal that reacts with water. The drug used to treat manic depression is not lithium atoms, Li, but rather lithium ions, Li+, usually given in the form of lithium carbonate, Li2CO3. Another example comes from the fluoridation of drinking water, and of toothpastes and dental gels. The element fluorine, F- , is an extremely poisonous and corrosive gas; it is not what is used for this purpose. Instead, 2 2 / 96 Chemical Bonds Chemistry Dr. Saeed Mohammed Ismaeel fluoridation uses fluoride ions, F-, in the form of sodium fluoride, NaF, a compound that is unreactive and nonpoisonous in the concentrations used. (a) Sodium chloride (b) Sodium (c) Chlorine How Do We Name Anions and Cations ?: A. Naming Monatomic Cations A monatomic (containing only one atom) cation forms when a metal loses one or more valence electrons. Elements of Groups 1A, 2A, and 3A form only one type of cation. For ions from these metals, the name of the cation is the name of the metal followed by the word “ion” (Table 1). There is no need to specify the charge on these cations, because only one charge is possible. For example, Na+ is sodium ion and Ca2+ is calcium ion. Most transition and inner transition elements form more than one type of cation and, therefore, the name of the cation must show its charge. To show the charge we write a Roman numeral immediately following (with no space) the name of the metal (Table 2). For example, Cu+ is copper(I) ion and Cu2+ is copper(II) ion. Copper(I) oxide and copper(II) oxide. The different copper ion charges result in different colors. 3 3 / 96 Chemical Bonds Chemistry Dr. Saeed Mohammed Ismaeel B. Naming Monatomic Anions A monatomic anion is named by adding -ide to the stem part of the name. Table 3 gives the names of the monatomic anions we deal with most often. C. Naming Polyatomic Ions A polyatomic ion contains more than one atom. Examples are the hydroxide ion, OH-, and the phosphate ion, PO43–. We will not be concerned with how these ions are formed, only that they exist and are present in the materials around us. While rules for naming polyatomic ions have been developed, the simplest thing to do is just to memorize them. The preferred system for naming polyatomic ions that differ in the number of hydrogen atoms is to use the prefixes di-, tri-, and so forth, to show the presence of more than one hydrogen. For example, HPO42– is hydrogen phosphate ion, and H2PO4– is dihydrogen phosphate ion. Table 4 lists some of the important polyatomic ions. Because several hydrogen-containing polyatomic anions have common names that are still widely used, you should memorize 4 4 / 96 Chemical Bonds Chemistry Dr. Saeed Mohammed Ismaeel them as well. In these common names, the prefix bi is used to show the presence of one hydrogen. Coral Chemistry and Broken Bonds: Bone is a highly structured matrix consisting of both inorganic and organic materials. The inorganic material is chiefly hydroxyapatite, Ca5(PO4)3OH, which makes up about 70% of bone by dry weight. By comparison, the enamel of teeth consists almost entirely of hydroxyapatite. Chief among the organic components of bone are collagen fibers, which thread their way through the inorganic matrix, providing extra strength and allowing bone to flex under stress. Also weaving through the hydroxyapatite–collagen framework are blood vessels that supply nutrients. A problem faced by orthopedic surgeons is how to repair bone damage. For a minor fracture, usually a few weeks in a cast suffice for the normal process of bone growth to repair the damaged area. For severe fractures, especially those involving bone loss, a bone graft may be needed. An alternative to a bone graft is an implant of synthetic bone material. One such material, called Pro Osteon, is derived by heating coral with ammonium hydrogen phosphate to form a hydroxyapatite similar to that of bone. Throughout the heating process, the porous structure of the coral, which resembles that of bone, is retained. The surgeon can shape a piece of this material to match the bone void, implant it, stabilize the area by inserting metal plates and/or screws, and let new bone tissue grow into the pores of the implant. In an alternative process, a dry mixture of calcium dihydrogen phosphate monohydrate, Ca(H2PO4)2 H2O, calcium phosphate, Ca3(PO4)2, and calcium carbonate, CaCO3, is prepared. Just before the surgical implant occurs, these chemicals are mixed with a solution of sodium phosphate to form a paste that is then injected into the bony area to be repaired. In this way, the fractured bony area is held in the desired position by the synthetic material while the natural process of bone rebuilding replaces the implant with living bone tissue. 5 5 / 96 Chemical Bonds Chemistry Dr. Saeed Mohammed Ismaeel Types of Chemical Bonds: A. Ionic and Covalent Bonds: According to the Lewis model of chemical bonding, atoms bond together in such a way that each atom participating in a bond acquires a valence-shell electron configuration matching that of the noble gas nearest to it in atomic number. Atoms acquire completed valence shells in two ways: 1. An atom may lose or gain enough electrons to acquire a filled valence shell, becoming an ion as it does so. An ionic bond results from the force of attraction between a cation and an anion. 2. An atom may share electrons with one or more other atoms to acquire a filled valence shell. A covalent bond results from the sharing of electrons between two atoms. We can now ask how to determine whether two atoms in a compound are bonded by an ionic bond or a covalent bond. One way to do so is to consider the relative positions of the two atoms in the Periodic Table. Ionic bonds usually form between a metal and a nonmetal. An example of an ionic bond is that formed between the metal sodium and the nonmetal chlorine in the compound sodium chloride, Na+Cl-. When two nonmetals or a metalloid and a nonmetal combine, the bond between them is usually covalent. Examples of compounds containing covalent bonds between nonmetals include Cl2, H2O, CH4, and NH3. Examples of compounds containing covalent bonds between a metalloid and a nonmetal include BF3, SiCl4, and AsH3. Another way to determine the bond type is to compare the electronegativities of the atoms involved. B. Electronegativity and Chemical Bonds: Electronegativity is a measure of an atom’s attraction for the electrons it shares in a chemical bond with another atom. The most widely used scale of electronegativities (Table 5) was devised by Linus Pauling in the 1930s. On the Pauling scale, fluorine, the most electronegative element, is assigned an electronegativity of 4.0, and all other elements are assigned values relative to fluorine. As you study the electronegativity values in Table 5, note that they generally increase from left to right across a row of the Periodic Table and from bottom to top within a column. Values increase from left to right because of the increasing positive charge on the nucleus, which leads to a stronger attraction for electrons in the valence shell. Values increase going 6 6 / 96 Chemical Bonds Chemistry Dr. Saeed Mohammed Ismaeel up a column because of the decreasing distance of the valence electrons from the nucleus, which leads to stronger attraction between a nucleus and its valence electrons. You might compare these trends in electronegativity with the trends in ionization energy. Each illustrates the periodic nature of elements within the Periodic Table. Ionization energy measures the amount of energy necessary to remove an electron from an atom. Electronegativity measures how tightly an atom holds the electrons that it shares with another atom. Notice that for periods within the Periodic Table, both electronegativity and ionization potential generally increase from left to right across a row of the Periodic Table from Columns 1A to 7A. In addition, both electronegativity and ionization potential increase in going up a column. Forming Ionic Bonds: According to the Lewis model of bonding, an ionic bond forms by the transfer of one or more valence-shell electrons from an atom of lower electronegativity to the valence shell of an atom of higher electronegativity. The more electronegative atom gains one or more valence electrons and becomes an anion; the less electronegative atom loses one or more valence electrons and becomes a cation. The compound formed by the combination of positive and negative ions is called an ionic compound. As a guideline, we say that this type of electron transfer to form an ionic compound is most likely to occur if the difference in electronegativity between two atoms is approximately 1.9 or greater. A bond is more likely to be covalent if this difference is less than 1.9. You 7 7 / 96 Chemical Bonds Chemistry Dr. Saeed Mohammed Ismaeel should be aware that the value of 1.9 for the formation of an ionic bond is somewhat arbitrary. Some chemists prefer a slightly larger value, others a slightly smaller value. The essential point is that the value of 1.9 gives us a guidepost against which to decide if a bond is more likely to be ionic or more likely to be covalent. An example of an ionic compound is that formed between the metal sodium (electronegativity 0.9) and the nonmetal chlorine (electronegativity 3.0). The difference in electronegativity between these two elements is 2.1. In forming the ionic compound NaCl, the single 3s valence electron of a sodium atom is transferred to the partially filled valence shell of a chlorine atom. The ionic bond in solid sodium chloride results from the force of attraction between positive sodium ions and negative chloride ions. In its solid (crystalline) form, sodium chloride consists of a three-dimensional array of Na+ and Cl- ions. Although ionic compounds do not consist of molecules, they do have a definite ratio of one kind of ion to another; their formulas give this ratio. For example, NaCl represents the simplest ratio of sodium ions to chloride ions—namely, 1:1. Predicting Formulas of Ionic Compounds: Ions are charged particles, but the matter we see all around us and deal with every day is electrically neutral (uncharged). If ions are present in any sample of matter, the total number of positive charges must equal the total number of negative charges. Therefore, we cannot have a sample containing only Na+ ions. Any sample that contains Na+ ions must also contain negative ions, such as Cl-, Br-, or S2-, and the sum of the positive charges must equal the sum of the negative charges. Remember that the subscripts in the formulas for ionic compounds represent the ratio of the ions. Thus a crystal of BaI2 has twice as many iodide ions as barium ions. For ionic compounds, when both charges are 2, as in the compound formed from Ba2+ and O2-, we must “reduce to lowest terms.” That is, barium oxide is BaO, not Ba2O2. The reason is that we are looking at ratios only, and the ratio of ions in barium oxide is 1:1. How Do We Name Ionic Compounds: To name an ionic compound, we give the name of the cation first followed by the name of the anion. A. Binary Ionic Compounds of Metals That Form Only One Positive Ion: A binary compound contains only two elements. In a binary ionic compound, both of the elements are present as ions. The name of the compound consists of the name of the metal 8 8 / 96 Chemical Bonds Chemistry Dr. Saeed Mohammed Ismaeel from which the cation (positive ion) was formed, followed by the name of the anion (negative ion). We ignore subscripts in naming binary ionic compounds. For example, AlCl3 is named aluminum chloride. We know this compound contains three chloride ions because the positive and negative charges must match—that is, one Al3+ ion must combine with three Cl- ions to balance the charges. B. Binary Ionic Compounds of Metals That Form More Than One Positive Ion: Table 2 shows that many transition metals form more than one positive ion. For example, copper forms both Cu+ and Cu2+ ions. For systematic names, we use Roman numerals in the name to show the charge. For common names we use the –ous /-ic system. C. Ionic Compounds That Contain Polyatomic Ions: To name ionic compounds containing polyatomic ions, name the positive ion first and then the negative ion, each as a separate word. Formation of a Covalent Bond: A covalent bond forms when electron pairs are shared between two atoms whose difference in electronegativity is less than 1.9. As we have already mentioned, the most common covalent bonds occur between two nonmetals or between a nonmetal and a metalloid. 9 9 / 96 Chemical Bonds Chemistry Dr. Saeed Mohammed Ismaeel According to the Lewis model, a pair of electrons in a covalent bond functions in two ways simultaneously: It is shared by two atoms and it fills the valence shell of each atom. The simplest example of a covalent bond is that in a hydrogen molecule, H2. When two hydrogen atoms bond, the single electrons from each atom combine to form an electron pair. A bond formed by sharing a pair of electrons is called a single bond and is represented by a single line between the two atoms. The electron pair shared between the two hydrogen atoms in H2 completes the valence shell of each hydrogen. Thus, in H2, each hydrogen has two electrons in its valence shell and an electron configuration like that of helium, the noble gas nearest to it in atomic number. H+H → H—H Nonpolar and Polar Covalent Bonds: Although all covalent bonds involve the sharing of electrons, they differ widely in the degree of sharing. We classify covalent bonds into two categories, nonpolar covalent and polar covalent, depending on the difference in electronegativity between the bonded atoms. In a nonpolar covalent bond, electrons are shared equally. In a polar covalent bond, they are shared unequally. It is important to realize that no sharp line divides these two categories, nor, for that matter, does a sharp line divide polar covalent bonds and ionic bonds. Nonpolar covalent bond: A covalent bond between two atoms whose difference in electronegativity is less than 0.5. Polar covalent bond: A covalent bond between two atoms whose difference in electronegativity is between 0.5 and 1.9. An example of a polar covalent bond is that in H–Cl, in which the difference in electronegativity between the bonded atoms is 3.0 – 2.1 = 0.9. A covalent bond between carbon and hydrogen, for example, is classified as nonpolar covalent because the difference in electronegativity between these two atoms is only 2.5 – 2.1 = 0.4. You should be aware, however, that there is some slight polarity to a C – H bond but, because it is quite small, we arbitrarily say that a C – H bond is nonpolar. 10 10 / 96 Chemical Bonds Chemistry Dr. Saeed Mohammed Ismaeel An important consequence of the unequal sharing of electrons in a polar covalent bond is that the more electronegative atom gains a greater fraction of the shared electrons and acquires a partial negative charge, indicated by the symbol δ- (read “delta minus”). The less electronegative atom has a lesser fraction of the shared electrons and acquires a partial positive charge, indicated by the symbol δ+ (read “delta plus”). This separation of charge produces a dipole (two poles). We commonly show the presence of a bond dipole by an arrow, with the head of the arrow near the negative end of the dipole and a cross on the tail of the arrow near the positive end (Figure 2). Figure 2 HCl is a polar covalent molecule. In the electron density model of HCl, re indicates a region of high electron density, and blue indicates aregion of low electron density. Bonding electrons Valence electrons involved in forming a covalent bond; that is, shared electrons. Nonbonding electrons Valence electrons not involved in forming covalent bonds; that is, unshared electrons. Single bond A bond formed by sharing one pair of electrons and represented by a single line between two atoms. Lewis Structure A formula for a molecule or ion showing all pairs of bonding electrons as single, double, or triple bonds, and all non-bonded electrons as pairs of Lewis dots. Structural formula A formula showing how atoms in a molecule or ion are bonded to each other; similar to a Lewis structure except that a structural formula typically shows only bonding pairs of electrons. Double bond A bond formed by sharing two pairs of electrons and represented by two lines between the two bonded atoms. Triple bond A bond formed by sharing three pairs of electrons and represented by three lines between the two bonded atoms. 11 11 / 96 Chemical Bonds Chemistry Dr. Saeed Mohammed Ismaeel Draw Lewis Structures: 1. Determine the number of valence electrons in the molecule. Add up the number of valence electrons contributed by each atom. To determine the number of valence electrons, you simply need to know the number of each kind of atom in the molecule. You don’t have to know anything about how the atoms are bonded to each other. Example: The Lewis structure for formaldehyde, CH2O, must show 12 valence electrons: 2. Determine the connectivity of the atoms (which atoms are bonded to each other) and connect bonded atoms by single bonds. Drawing a Lewis structure is often the most challenging task. For some molecules we give you as examples or problems, we ask you to propose a connectivity. For most, however, we give you the experimentally determined connectivity and ask you to complete the Lewis structure. Example: The atoms in formaldehyde are bonded in the following order. Note that we do not attempt at this point to show bond angles or the three-dimensional shape of the molecule; we just show what is bonded to what. This partial structure shows six valence electrons in the three single bonds. In it we have accounted for six of the 12 valence electrons. 3. Arrange the remaining electrons so that each atom has a complete outer shell. Each hydrogen atom must be surrounded by two electrons. Each carbon, nitrogen, oxygen, and halogen atom must be surrounded by eight valence electrons (to satisfy the octet rule). The remaining valence electrons may be shared between atoms in bonds or may be unshared pairs on a single atom. A pair of electrons involved in a covalent bond (bonding electrons) is shown as a single line; an unshared pair of electrons (nonbonding electrons) is shown as a pair of Lewis dots. By placing two pairs of bonding electrons between C and O, we give carbon a complete octet. By placing the remaining four electrons on oxygen as two Lewis dot pairs, we give oxygen eight valence electrons and a complete octet (octet rule). Note that we placed the two pairs of bonding electrons between C and O before we assigned the unshared pairs of electrons on the oxygen. As a check on this structure, verify (1) that each atom has a complete valence shell (which each does) and (2) that the Lewis structure has the correct number of valence electrons (12, which it does). 4. In a double bond, two atoms share two pairs of electrons; we represent a double bond by two lines between the bonded atoms. Double bonds are most common between atoms of C, N, O, and S. In the organic and biochemistry chapters in particular, we shall see many examples of C = C, C = N, and C = O double bonds. 5. In a triple bond, two atoms share three pairs of electrons; we show a triple bond by three lines between the bonded atoms. Triple bonds are most common between atoms of C and N—for example, C ≡ C and C ≡ N triple bonds. 12 12 / 96 Chemical Bonds Chemistry Dr. Saeed Mohammed Ismaeel Table 7 gives Lewis structures and names for several small molecules. Notice that each hydrogen is surrounded by two valence electrons, and that each carbon, nitrogen, oxygen, and chlorine is surrounded by eight valence electrons. Furthermore, each carbon has four bonds, each nitrogen has three bonds and one unshared pair of electrons, each oxygen has two bonds and two unshared pairs of electrons, and chlorine (as well as other halogens) has one bond and three unshared pairs of electrons. D. Exceptions to the Octet Rule: The Lewis model of covalent bonding focuses on valence electrons and the necessity for each atom other than hydrogen to have a completed valence shell containing eight electrons. Although most molecules formed by main-group elements (Groups 1A–7A) have structures that satisfy the octet rule, some important exceptions exist. One exception involves molecules that contain an atom with more than eight electrons in its valence shell. Atoms of period 2 elements use one 2s and three 2p orbitals for bonding; these four orbitals can contain only eight valence electrons—hence the octet rule. Atoms of period 3 elements, however, have one 3 s orbital, three 3p orbitals, and five 3d orbitals; they can accommodate more than eight electrons in their valence shells. In 13 13 / 96 Chemical Bonds Chemistry Dr. Saeed Mohammed Ismaeel phosphine, PH3, phosphorus has eight electrons in its valence shell and obeys the octet rule. The phosphorus atoms in phosphorus pentachloride, PCl5, and phosphoric acid, H3PO4, have ten electrons in their valence shells and, therefore, are exceptions to the octet rule. Sulfur, another period 3 element, forms compounds in which it has 8, 10, and even 12 electrons in its valence shell. The sulfur atom in H2S has 8 electrons in its valence shell and obeys the octet rule. The sulfur atoms in SO2 and H2SO4 have 10 and 12 electrons in their valence shells and are exceptions to the octet rule. How Do We Name Binary Covalent Compounds ?: A binary covalent compound is a binary (two-element) compound in which all bonds are covalent. In naming a binary covalent compound: 1. First name the less electronegative element (see Table 5). Note that the less electronegative element is also generally written first in the formula. 2. Then name the more electronegative element. To name it, add -ide to the stem name of the element. Chlorine, for example, becomes chloride, and oxygen becomes oxide. 3. Use the prefixes di-, tri-, tetra-, and so on to show the number of atoms of each element. The prefix mono- is omitted when it refers to the first atom named, and it is rarely used with the second atom. An exception to this rule is CO, which is named carbon monoxide. The name is then written as two words. 14 14 / 96 Chemical Bonds Chemistry Dr. Saeed Mohammed Ismaeel What is Resonance ?: As chemists developed a deeper understanding of covalent bonding in organic and inorganic compounds, it became obvious that, for a great many molecules and ions, no single Lewis structure provides a truly accurate representation. For example, Figure 3 shows three Lewis structures for the carbonate ion, CO32 ־. In each structure, carbon is bonded to three oxygen atoms by a combination of one double bond and two single bonds. Each Lewis structure implies that one carbon-oxygen bond is different from the other two. However, this is not the case. It has been determined experimentally that all three carbon-oxygen bonds are identical. The problem for chemists, then, is how to describe the structure of molecules and ions for which no single Lewis structure is adequate and yet still retain Lewis structures. As an answer to this problem, Linus Pauling proposed the theory of resonance. Figure 3 (a–c ) Three Lewis structures for the carbonate ion. A. Theory of Resonance: The theory of resonance, developed primarily by Pauling in the 1930s, provides an explanation. According to this theory, many molecules and ions are best described by writing two or more Lewis structures and considering the real molecule or ion to be a hybrid of these structures. Individual Lewis structures are called contributing structures (or sometimes resonance structures or resonance contributors). We show that the real molecule or ion is a resonance hybrid of the various contributing structures by interconnecting them with double-headed arrows. Do not confuse the double-headed arrow with the double arrow used to show chemical equilibrium. As we explain shortly, resonance structures are not in equilibrium with each other. Figure 4 shows three contributing structures for the carbonate ion. These contributing structures are said to be equivalent; all three have identical patterns of covalent bonding. 15 15 / 96 Chemical Bonds Chemistry Dr. Saeed Mohammed Ismaeel How To Draw Curved Arrows and Pushed Electrons ?: Notice in Figure 4 that the only difference among contributing structures (a), (b), and (c) is the position of the valence electrons. To generate one resonance structure from another, chemists use a curved arrow. The arrow indicates where a pair of electrons originates (the tail of the arrow) and where it is repositioned in the next structure (the head of the arrow). A curved arrow is nothing more than a bookkeeping symbol for keeping track of electron pairs or, as some call it, electron pushing. Do not be misled by its simplicity. Electron pushing will help you see the relationship among contributing structures. Following are contributing structures for the nitrite and acetate ions. Curved arrows show how the contributing structures are interconverted. For each ion, the contributing structures are equivalent: They have the same bonding pattern. A common mistake is to use curved arrows to indicate the movement of atoms or positive charges. This is never correct. Curved arrows are used only to show the repositioning of electron pairs, when a new resonance hybrid is generated. Figure 4 (a–c ) The carbonate ion represented as a hybrid of three equivalent contributing structures. Curved arrows (in red) show how electron pairs are redistributed from one contributing structure to the next. Resonance : A theory that many molecules and ions are best described as a hybrid of two or more Lewis contributing structures. Contributing structure: Representations of a molecule or ion that differ only in the distribution of valence electrons. 16 16 / 96 Chemical Bonds Chemistry Dr. Saeed Mohammed Ismaeel Resonance hybrid: A molecule or ion described as a composite or hybrid of a number of contributing structures. Double-headed arrow: A symbol used to show that the structures on either side of it are resonance contributing structures. The use of the term “resonance” for this theory of covalent bonding might appear to suggest that bonds and electron pairs are constantly changing back and forth from one position to another over time. This notion is not at all correct. The carbonate ion, for example, has one— and only one—real structure. The problem is ours: How do we draw that one real structure? The resonance method offers a way to describe the real structure while simultaneously retaining Lewis structures with electron-pair bonds and showing all nonbonding pairs of electrons. Thus, although we realize that the carbonate ion is not accurately represented by any one contributing structure shown in Figure 4, we continue to represent it by one of these for convenience. We understand, of course, that we are referring to the resonance hybrid. One consequence of resonance is that it is a stabilizing factor when it exists—that is, a resonance hybrid is more stable than chemists would estimate or calculate compared to any one of its contributing structures. We will see three particularly striking illustrations of the stability of resonance hybrids when we consider the unusual chemical properties of benzene and aromatic hydrocarbons, the acidity of carboxylic acids, and the geometry of the amide bonds in proteins. B. Writing Acceptable Contributing Structures: Certain rules must be followed to write acceptable contributing structures: 1. All contributing structures must have the same number of valence electrons. 2. All contributing structures must obey the rules of covalent bonding. In particular, no contributing structure may have more than two electrons in the valence shell of hydrogen or more than eight electrons in the valence shell of a second-period element. Third-period elements, such as phosphorus and sulfur, may have up to 12 electrons in their valence shells. 3. The positions of all nuclei must be the same in all resonance structures; that is, contributing structures must differ only in the distribution of valence electrons. 4. All contributing structures must have the same number of paired and unpaired electrons. 17 17 / 96 Chemical Bonds Chemistry Dr. Saeed Mohammed Ismaeel A final note: Do not confuse resonance contributing structures with equilibration among different species. A molecule described as a resonance hybrid is not equilibrating among the individual electron configurations of the contributing structures. Rather, the molecule has only one structure, which is best described as a hybrid of its various contributing structures. The colors on the color wheel provide a good analogy. Purple is not a primary color; the primary colors of blue and red are mixed to make purple. You can think of molecules represented by resonance hybrids as being purple. Purple is not sometimes blue and sometimes red: Purple is purple. In an analogous way, a molecule described as a resonance hybrid is not sometimes one contributing structure and sometimes another: It is a single structure all the time. How Do We Predict Bond Angles in Covalent Molecules ?: We can predict bond angles in molecules by using the valence-shell electron-pair repulsion (VSEPR) model. According to this model, the valence electrons of an atom may be involved in the formation of single, double, or triple bonds, or they may be unshared. Each combination creates a negatively charged region of electron density around the nucleus. Because like charges repel each other, the various regions of electron density around an atom spread out so that each is as far away as possible from the others. We use the VSEPR model and the balloon model analogy in the following way to predict the shape of a molecule of methane, CH4. The Lewis structure for CH4 shows a carbon atom surrounded by four regions of electron density. Each region contains a pair of electrons forming a single covalent bond to a hydrogen atom. According to the VSEPR model, the four regions radiate from carbon so that they are as far away from one another as possible. The maximum separation occurs when the angle between any two regions of electron density is 109.5°. Therefore, we predict all H – C – H bond angles to be 109.5°, and the shape of the molecule to be tetrahedral (Figure 6). The H – C – H bond angles in methane have been measured experimentally and found to be 109.5°. Thus the bond angles and shape of methane predicted by the VSEPR model are identical to those observed experimentally. We can predict the shape of an ammonia molecule, NH3, in the same way. The Lewis structure of NH3 shows nitrogen surrounded by four regions of electron density. Three 18 18 / 96 Chemical Bonds Chemistry Dr. Saeed Mohammed Ismaeel Figure 5 Balloon models to predict bond angles. (a) Two balloons assume a linear shape with a bond angle of 180° about the tie point. (b) Three balloons assume a trigonal planar shape with bond angles of 120° about the tie point. (c) Four balloons assume a tetrahedral shape with bond angles of 109.5° about the tie point. regions contain single pairs of electrons that form covalent bonds with hydrogen atoms. The fourth region contains an unshared pair of electrons [Figure 7(a)]. Using the VSEPR model, we predict that the four regions are arranged in a tetrahedral manner and that the three H – N – H bond angles in this molecule are 109.5°. The observed bond angles are 107.3°. We can explain this small difference between the predicted and the observed angles by proposing that the unshared pair of electrons on nitrogen repels adjacent electron pairs more strongly than the bonding pairs repel one another. Figure 6 The shape of a methane molecule, Figure 7 The shape of an ammonia molecule, CH4. (a) Lewis structure and (b) ball-and-stick NH3. (a) Lewis structure and (b) ball-and-stick model. The hydrogens occupy the four corners model. The H - N - H bond angles are 107.3°, of a regular tetrahedron, and all H-C-H bond slightly smaller than the H - C- H bond angles angles are 109.5°. of methane. 19 19 / 96 Chemical Bonds Chemistry Dr. Saeed Mohammed Ismaeel The geometry of an ammonia molecule is described as pyramidal; that is, the molecule is shaped like a triangular-based pyramid with the three hydrogens located at the base and the single nitrogen located at the apex. Figure 8 shows a Lewis structure and a ball-and-stick model of a water molecule. In H2O, oxygen is surrounded by four regions of electron density. Two of these regions contain pairs of electrons used to form single covalent bonds to hydrogens; the remaining two regions contain unshared electron pairs. Using the VSEPR model, we predict that the four regions of electron density around oxygen are arranged in a tetrahedral manner and that the H – O – H bond angle is 109.5°. Experimental measurements show that the actual H – O – H bond angle in a water molecule is 104.5°, a value smaller than that predicted. We can explain this difference between the predicted and the observed bond angle by proposing, as we did for NH3, that unshared pairs of electrons repel adjacent pairs more strongly than bonding pairs do. Note that the distortion from 109.5° is greater in H2O, which has two unshared pairs of electrons, than it is in NH3, which has only one unshared pair. Figure 8 The shape of a water molecule, H2O. (a) Lewis structure and (b) ball-and-stick model. A general prediction emerges from this discussion. If a Lewis structure shows four regions of electron density around an atom, the VSEPR model predicts a tetrahedral distribution of electron density and bond angles of approximately 109.5°. In many of the molecules we will encounter, an atom is surrounded by three regions of electron density. Figure 9 shows Lewis structures and ball- and- stick models for molecules of formaldehyde, CH2O, and ethylene, C2H4. 20 20 / 96 Chemical Bonds Chemistry Dr. Saeed Mohammed Ismaeel Figure 9 The shapes of formaldehyde, CH2O, and ethylene, C2H4. In the VSEPR model, we treat a double bond as a single region of electron density. In formaldehyde, carbon is surrounded by three regions of electron density. Two regions contain single pairs of electrons, each of which forms a single bond to a hydrogen; the third region contains two pairs of electrons, which form a double bond to oxygen. In ethylene, each carbon atom is also surrounded by three regions of electron density; two contain single pairs of electrons, and the third contains two pairs of electrons. Three regions of electron density about an atom are farthest apart when they lie in a plane and make angles of 120° with one another. Thus the predicted H – C – H and H – C – O bond angles in formaldehyde and the H – C – H and H – C – C bond angles in ethylene are all 120°. Furthermore, all atoms in each molecule lie in a plane. Thus, both formaldehyde and ethylene are planar molecules. The geometry about an atom surrounded by three regions of electron density, as in formaldehyde and ethylene, is described as trigonal planar. In still other types of molecules, a central atom is surrounded by two regions of electron density. Figure 10 shows Lewis structures and balland- stick models of molecules of carbon dioxide, CO2, and acetylene, C2H2. In carbon dioxide, carbon is surrounded by two regions of electron density; each contains two pairs of electrons and forms a double bond to an oxygen atom. In acetylene, 21 21 / 96 Chemical Bonds Chemistry Dr. Saeed Mohammed Ismaeel each carbon is also surrounded by two regions of electron density; one contains a single pair of electrons and forms a single bond to a hydrogen atom, and the other contains three pairs of electrons and forms a triple bond to a carbon atom. In each case, the two regions of electron density are farthest apart if they form a straight line through the central atom and create an angle of 180°. Both carbon dioxide and acetylene are linear molecules. Table 8 summarizes the predictions of the VSEPR model. In this table, three- dimensional shapes are shown using a solid wedge to represent a bond coming toward you, out of the plane of the paper. A broken wedge represents a bond going away from you, behind the plane of the paper. A single line represents a bond in the plane of the paper. How Do We Determine If a Molecule Is Polar ?: We used the terms “polar” and “dipole” to describe a covalent bond in which one atom bears a partial positive charge and the other bears a partial negative charge. We also saw that we can use the difference in electronegativity between bonded atoms to determine the polarity of a covalent bond and the direction of its dipole. We can now combine our understanding of bond polarity and molecular geometry to predict the polarity of molecules. To discuss the physical and chemical properties of a molecule, it is essential to have an understanding of polarity. 22 22 / 96 Chemical Bonds Chemistry Dr. Saeed Mohammed Ismaeel Many chemical reactions, for example, are driven by the interaction of the positive part of one molecule with the negative part of another molecule. A molecule will be polar if (1) it has polar bonds and (2) its centers of partial positive charge and partial negative charge lie at different places within the molecule. Consider first carbon dioxide, CO2, a molecule with two polar carbon-oxygen double bonds. The oxygen on the left pulls electrons of the O = C bond toward it, giving it a partial negative charge. Similarly, the oxygen on the right pulls electrons of the C = O bond toward it by the same amount, giving it the same partial negative charge as the oxygen on the left. Carbon bears a partial positive charge. We can show the polarity of these bonds by using the symbols δ+ and δ-. Alternatively, we can show that each carbon-oxygen bond has a dipole by using an arrow, where the head of the arrow points to the negative end of the dipole and the crossed tail is positioned at the positive end of the dipole. Because carbon dioxide is a linear molecule, its centers of negative and positive partial charge coincide. Therefore, CO2 is a nonpolar molecule; that is, it has no dipole. In a water molecule, each O – H bond is polar. Oxygen, the more electronegative atom, bears a partial negative charge and each hydrogen bears a partial positive charge. The center of partial positive charge in a water molecule is located halfway between the two hydrogen atoms, and the center of partial negative charge is on the oxygen atom. Thus a water molecule has polar bonds and, because of its geometry, is a polar molecule. 23 23 / 96 Chemical Bonds Chemistry Dr. Saeed Mohammed Ismaeel Ammonia has three polar N – H bonds. Because of its geometry, the centers of partial positive and partial negative charges are found at different places within the molecule. Thus ammonia has polar bonds and, because of its geometry, is a polar molecule. 24 24 / 96 Chemical Reactions Chemistry Dr. Saeed Mohammed Ismaeel CHEMICAL REACTIONS In a chemical change, also called a chemical reaction, one or more reactants (starting materials) are converted into one or more products. Chemical reactions occur all around us. They fuel and keep alive the cells of living tissues; they occur when we light a match, cook dinner, start a car, listen to a portable radio, or watch television. Most of the world’s manufacturing processes involve chemical reactions; they include petroleum refining and food processing as well as the manufacture of drugs, plastics, synthetic fibers, explosives, and many other materials. In this chapter we discuss four aspects of chemical reactions: (1) how to write and balance chemical equations, (2) mass relationships in chemical reactions, (3) types of chemical reactions, and (4) heat gains and losses. What are Molecular Weights and Formula Weights, And How They Differ?: The formula weight (FW) of any substance is the sum of the atomic weights in atomic mass units (amu) of all the atoms in the compound’s formula. The term “formula weight” can be used for both ionic and molecular compounds and tells nothing about whether the compound is ionic or molecular. Table 1 gives formula weights for two ionic compounds and two covalent compounds. Another term, molecular weight (MW), is strictly correct only when used for covalent compounds. In this book, we use “formula weight” for both ionic and covalent compounds and “molecular weight” only for covalent compounds. 1 25 / 96 Chemical Reactions Chemistry Dr. Saeed Mohammed Ismaeel What Is a Mole And How Do We Use It To Calculate Mass Relationship's?: Mole (mol) The formula weight of a substance expressed in grams. The number of formula units in a mole is called Avogadro’s number after the Italian physicist, Amadeo Avogadro (1776–1856), who first proposed the concept of a mole, but was not able to determine the number experimentally. Note that Avogadro’s number is not a defined value, but rather a value that must be determined experimentally. Its value is now known to nine significant figures. Avogadro’s number = 6.02214199 х 1023 formula unite per mole For most calculations in this text, we round this number to three significant figures to 6.02 х 1023 formula units per mole. A mole of hydrogen atoms is 6.02 х 1023 hydrogen atoms, a mole of sucrose (table sugar) molecules is 6.02 х 1023 sugar molecules, a mole of apples is 6.02 х 1023 apples, and a mole of sodium ions is 6.02 х 1023 sodium ions. We call 6.02 х 1023 of anything a mole. The molar mass of any substance (the mass of one mole of any substance) is the formula weight of the substance expressed in grams. For instance, the formula weight of glucose, C6H12O6 (Example 1) is 180 amu; therefore, 180 g of glucose is one mole of glucose. Likewise, the formula weight of urea, (NH2)2CO, is 60.0 amu and, therefore, 60.0 g of (NH2)2CO is one mole of urea. For atoms, one mole is the atomic weight expressed in grams: 12.0 g of carbon is one mole of carbon atoms; 32.1 g of sulfur is one mole of sulfur atoms; and so on. Suppose we want to know the number of moles of water in a graduated cylinder that contains 36.0 g of water. We know that the molar mass of water is 18.0 g/mol. If 18.0 g of water is one mole of water, then 36.0 g must be two moles of water. Molar mass can also be used to convert from moles to grams. Suppose you have a beaker that contains 0.753 mol of sodium chloride and you are asked to calculate the number of grams of sodium chloride in the beaker. As a conversion factor, use the fact that the molar mass of NaCl is 58.5 g/mol. 2 26 / 96 Chemical Reactions Chemistry Dr. Saeed Mohammed Ismaeel Balance a Chemical Equation: To balance an equation, we place numbers in front of the formulas until the number of each kind of atom in the products is the same as the number in the starting materials. These numbers are called coefficients. As an example, let us balance our propane equation: To balance an equation: 1. Begin with atoms that appear in only one compound on the left and only one compound on the right. In the equation for the reaction of propane and oxygen, begin with either carbon or hydrogen. 2. If an atom occurs as a free element—as, for example, O2 does in the reaction of propane with oxygen—balance this element last. 3. You can change only coefficients in balancing an equation; you cannot change chemical formulas. For example, if you have H2O on the left side of an equation but need two oxygens, you can add the coefficient “2” to read 2H2O. You cannot, however, get two oxygens by changing the formula to H2O2. You can’t do this because the reactant is water, H2O, and not hydrogen peroxide, H2O2. For the equation for the reaction of propane with oxygen, we can begin with carbon. Three carbon atoms appear on the left and one on the right. If we put a 3 in front of the CO2 (indicating that three CO2 molecules are formed), three carbons will appear on each side, and the carbons will be balanced: Next we look at the hydrogens. There are eight on the left and two on the right. If we put a 4 in front of the H2O, there will be eight hydrogens on each side and the hydrogens will be balanced: The only atom still unbalanced is oxygen. Notice that we saved this reactant for last (rule 2). There are two oxygen atoms on the left and ten on the right. If we put a 5 in front of the O2 on the left, we both balance the oxygen atoms and arrive at the balanced equation: At this point, the equation ought to be balanced, but we should always check, just to make sure. In a balanced equation, there must be the same number of atoms of each element on both sides. A check of our work shows three C, ten O, and eight H atoms on each side. The equation is, indeed, balanced. 3 27 / 96 Chemical Reactions Chemistry Dr. Saeed Mohammed Ismaeel How Can We Predict if Ions In Aqueous Solution Will React With Each Other ?: Many ionic compounds are soluble in water. Ionic compounds always consist of both positive and negative ions. When they dissolve in water, the positive and negative ions become separated from each other by water molecules. We call such a separation a dissociation. For example, What happens when we mix aqueous solutions of two different ionic compounds? Does a reaction take place between the ions? The answer depends on the ions. If any of the negative and positive ions come together to form a water-insoluble compound, then a reaction takes place and a precipitate forms; otherwise, no reaction occurs. As an example, suppose we prepare one solution by dissolving sodium chloride, NaCl, in water and a second solution by dissolving silver nitrate, AgNO3, in water. If we now mix the two solutions, four ions are present in the solution: Ag +, Na+, Cl-, and NO. Two of these ions, Ag+ and Cl-, react to form the compound AgCl (silver chloride), which is insoluble in water. A reaction therefore takes place, forming a white precipitate of AgCl that slowly sinks to the bottom of the container (Figure 1). We write this reaction as follows: Notice that the Na1 and NO ions do not participate in the reaction, but merely remain dissolved in the water. Ions that do not participate in a reaction are called spectator ions (Spectator ion An ion that appears unchanged on both sides of a chemical equation). We can simplify the equation for the formation of silver chloride by omitting all spectator ions: This kind of equation that we write for ions in solution is called a net ionic equation. Like all other chemical equations, net ionic equations must be balanced. We balance them in the same way as we do other equations, except now we must make sure that charges balance as well as atoms. 4 28 / 96 Chemical Reactions Chemistry Dr. Saeed Mohammed Ismaeel Figure 1 Adding Cl‾ ions to a solution of Ag+ ions produces a white precipitate of silver chloride, AgCl. Net ionic equations show only the ions that react—no spectator ions are shown. For example, the net ionic equation for the precipitation of arsenic(III) sulfide from aqueous solution is Not only are there two arsenic atoms and three sulfur atoms on each side, but the total charge on the left side is the same as the total charge on the right side; they are both zero. In general, ions in solution react with each other only when one of these four things can happen: 1. Two ions form a solid that is insoluble in water. AgCl is one example. 2. Two ions form a gas that escapes from the reaction mixture as bubbles. An example is the reaction of sodium bicarbonate, NaHCO 3, with HCl to form gaseous carbon dioxide, CO2. The net ionic equation for this reaction is 3. An acid neutralizes a base. Acid–base reactions. 4. One of the ions can oxidize another. In many cases, no reaction takes place when we mix solutions of ionic compounds because none of these situations holds. For example, if we mix solutions of copper(II) nitrate, Cu(NO3)2, and potassium sulfate, K2SO4, we merely have a mixture containing Cu2+, K+, NO3‾ , and SO42 ‾ ions dissolved in water. 5 29 / 96 Chemical Reactions Chemistry Dr. Saeed Mohammed Ismaeel Solubility and Tooth Decay: The outermost protective layer of a tooth is the enamel, which is composed of approximately 95% hydroxyapatite, Ca10(PO4)6(OH)2, and 5% collagen. Like most other phosphates and hydroxides, hydroxyapatite is insoluble in water. In acidic media, however, it dissolves to a slight extent, yielding Ca2+, PO43‾ , and OH‾ ions. This loss of enamel creates pits and cavities in the tooth. Acidity in the mouth is produced by bacterial fermentation of remnants of food, especially carbohydrates. Once pits and cavities form in the enamel, bacteria can hide there and cause further damage in the underlying softer material called dentin. The fluoridation of water brings F‾ ions to the hydroxyapatite. There, F‾ ions take the place of OH‾ ions, forming the considerably less acid-soluble fluoroapatite, Ca10(PO4)6F2. Fluoride-containing toothpastes enhance this exchange process and provide further protection against tooth decay. Of the four ways for ions to react in water, one of the most common is the formation of an insoluble compound. We can predict when this result will happen if we know the solubilities of the ionic compounds. There is no simple way to remember which ionic solids are soluble in water and which are not, but we can make some useful generalizations. 1. All compounds containing Na+, K+, or NH4+ ions are soluble in water. 2. All nitrates (NO3‾ ) and acetates (CH3COO‾ ) are soluble in water. 3. Most chlorides (Cl2) and sulfates (SO42‾ ) are soluble in water. Important exceptions include silver chloride (AgCl), lead(II) chloride (PbCl2), mercury(I) chloride (Hg2Cl2), barium sulfate (BaSO4), and lead sulfate (PbSO4), which are insoluble in water. 4. Most carbonates (CO32‾ ), phosphates (PO43‾ ), sulfides (S2‾), and hydroxides (OH‾) are insoluble in water. Important exceptions include LiOH, NaOH, KOH, and aqueous NH3, which are soluble in water. What Are Oxidation and Reduction, and Why Do They Always Occurs Together: One of the most important and most common types of reaction is oxidation–reduction. Oxidation (The loss of electrons; the gain of oxygen atoms or the loss of hydrogen atoms). Reduction (The gain of electrons; the loss of oxygen atoms or the gain of hydrogen atoms ). An oxidation–reduction reaction (often called a redox reaction) involves the transfer of electrons from one species to another. An example is the oxidation of zinc by copper ions, the net ionic equation for which is When we put a piece of zinc metal into a beaker containing copper(II) ions in aqueous solution, three things happen: 6 30 / 96 Chemical Reactions Chemistry Dr. Saeed Mohammed Ismaeel 1. Some of the zinc metal dissolves and goes into solution as Zn2+. 2. Copper metal is deposited on the zinc metal. 3. The blue color of the Cu2+ ions gradually disappears. The zinc metal loses electrons to the copper ions: At the same time, the Cu2+ ions gain electrons from the zinc. The copper ions are reduced: Oxidation and reduction are not independent reactions. In other words, no oxidation can occur without an accompanying reduction, and vice versa. In the preceding reaction, Cu2+ is oxidizing Zn. We call Cu2+ an oxidizing agent. Similarly, Zn is reducing Cu2+, and we call Zn a reducing agent. We summarize these oxidation–reduction relationships for the reaction of zinc metal with Cu(II) ion in the following way: Although the definitions we have given for oxidation (loss of electrons) and reduction (gain of electrons) are easy to apply in many redox reactions, they are not so easy to apply in other cases. For example, another redox reaction is the combustion (burning) of methane, CH4, in which CH4 is oxidized to CO2 while O2 is reduced to CO2 and H2O: It is not easy to see the electron loss and gain in such a reaction, so chemists developed another definition of oxidation and reduction, one that is easier to apply in many cases, especially where organic compounds are involved: Oxidation The gain of oxygen and/or the loss of hydrogen Reduction The loss of oxygen and/or the gain of hydrogen 7 31 / 96 Chemical Reactions Chemistry Dr. Saeed Mohammed Ismaeel Applying these alternative definitions to the reaction of methane with oxygen, we find the following: We have said that redox reactions are extremely common. Here are some important categories: 1. Combustion All combustion (burning) reactions are redox reactions in which the compounds or mixtures that are burned are oxidized by oxygen, O2. They include the burning of gasoline, diesel oil, fuel oil, natural gas, coal, wood, and paper. All these materials contain carbon, and all except coal also contain hydrogen. If the combustion is complete, carbon is oxidized to CO2 and hydrogen is oxidized to H2O. In an incomplete combustion, these elements are oxidized to other compounds, many of which cause air pollution. Unfortunately, much of today’s combustion that takes place in gasoline and diesel engines and in furnaces is incomplete, so it contributes to air pollution. In the incomplete combustion of methane, for example, carbon is oxidized to carbon monoxide, CO, due to a lack of sufficient oxygen to complete its oxidation to CO2: 2. Respiration Humans and animals get their energy by respiration. The oxygen in the air we breathe oxidizes carbon-containing compounds in our cells to produce CO2 and H2O. In fact, this oxidation reaction is really the same as combustion, although it takes place more slowly and at a much lower temperature. The important product of these reactions is not CO2 (which the body eliminates) or H2O, but energy. 3. Rusting When iron or steel objects are left out in the open air, they eventually rust (steel is mostly iron but contains certain other elements, too). In rusting, iron is oxidized to a mixture of iron oxides. We can represent the main reaction by the following equation: 4Fe(s) + 3O2(g) → 2Fe2O3(s) 4. Bleaching Most bleaching involves oxidation, and common bleaches are oxidizing agents. The colored compounds being bleached are usually organic compounds; oxidation converts them to colorless compounds. 8 32 / 96 Chemical Reactions Chemistry Dr. Saeed Mohammed Ismaeel 5. Batteries A voltaic cell is a device in which electricity is generated from a chemical reaction. Such cells are often called batteries. We are all familiar with batteries in our cars and in such portable devices as radios, flashlights, cell phones, and computers. In all cases, the reaction taking place in the battery is a redox reaction. What Is Heat of Reaction ?: In almost all chemical reactions, not only are starting materials converted to products, but heat is also either given off or absorbed. For example, when one mole of carbon is oxidized by oxygen to produce one mole of CO2, 94.0 kilocalories of heat is given off: C(s) + O2(g) ➙ CO2(g) + 94.0 kcal The heat given off or gained in a reaction is called the heat of reaction. A reaction that gives off heat is exothermic; a reaction that absorbs heat is endothermic. The amount of heat given off or absorbed is proportional to the amount of material. For example, when 2 mol of carbon is oxidized by oxygen to give carbon dioxide, 2 х 94.0 = 188 kcal of heat is given off. The energy changes accompanying a chemical reaction are not limited to heat. In some reactions, such as in voltaic cells , the energy given off takes the form of electricity. In other reactions, such as photosynthesis (the reaction whereby plants convert water and carbon dioxide to sugar and oxygen), the energy absorbed is in the form of light. An example of an endothermic reaction is the decomposition of mercury(II) oxide: This equation tells us that, if we want to decompose 2 mol of mercury(II) oxide into the elements Hg(l) and O2(g), we must add 43.4 kcal of energy to HgO. Incidentally, the law of conservation of energy tells us that the reverse reaction, the oxidation of mercury, must give off exactly the same amount of heat: 2Hg(l) + O2(g) ➙ 2HgO(s) + 43.4 kcal Especially important are the heats of reaction for combustion reactions. Combustion reactions are the most important heat-producing reactions, because most of the energy required for modern society to function is derived from them. All combustions are exothermic. The heat given off in a combustion reaction is called the heat of combustion. 9 33 / 96 Reaction Rates & Chemical Equilibrium Chemistry Dr. Saeed Mohammed Ismaeel REACTION RATES & CHEMICAL EQUILIBRIUM How Do We Measure Reaction Rates?: Some chemical reactions take place rapidly; others are very slow. For example, glucose and oxygen gas react with each other to form water and carbon dioxide: This reaction is extremely slow, however. A sample of glucose exposed to O 2 in the air shows no measurable change even after many years. In contrast, consider what happens when you take one or two aspirin tablets for a slight headache. Very often, the pain disappears in half an hour or so. Thus the aspirin must have reacted with compounds in the body within that time. Many reactions occur even faster. For example, if we add a solution of silver nitrate to a solution of sodium chloride (NaCl), a precipitate of silver chloride (AgCl) forms almost instantaneously. Net ionic equation: Ag +(aq) + Cl ‾(aq) ➙ AgCl(s) The precipitation ofAgCl is essentially complete in considerably less than 1 s. The study of reaction rates is called chemical kinetics. The rate of a reaction is the change in concentration of a reactant (or product) per unit time. Every reaction has its own rate, which must be measured in the laboratory. Consider the following reaction carried out in the solvent acetone: To determine the reaction rate, we can measure the concentration of the product, iodomethane, in the acetone at periodic time intervals—say, every 10 min. For example, the concentration might increase from 0 to 0.12 mol/L over a period of 30 min. The rate of the reaction is the change in the concentration of iodomethane divided by the time interval: This unit is read “0.0040 mole per liter per minute.” During each minute of the reaction, an average of 0.0040 mol of chloromethane is converted to iodomethane for each liter of solution. 1 34 / 96 Reaction Rates & Chemical Equilibrium Chemistry Dr. Saeed Mohammed Ismaeel Why Do Some Molecular Collisions Result In Reaction Whereas Others Do Not ?: Why are some collisions effective whereas others are not? There are two main reasons: 1. In most cases, for a reaction to take place between A and B, one or more covalent bonds must be broken in A or B or both, and energy is required for this to happen. The energy comes from the collision between A and B. If the energy of the collision is large enough, bonds will break and a reaction will take place. If the collision energy is too low, the molecules will bounce apart without reacting. The minimum energy necessary for a reaction to occur is called the activation energy. The energy of any collision depends on the relative speeds (that is, on the relative kinetic energies) of the colliding objects and on their angle of approach. Much greater damage is done in a head-on collision of two cars both going 40 mi/h than in a collision in which a car going 20 mi/h sideswipes one going 10 mi/h. The same consideration applies with molecules, as Figure 1 shows. Figure 2 The energy of molecular collisions varies. (a) Two fast-moving molecules colliding head-on have a higher collision energy than (b) two slower-moving molecules colliding at an angle. 2. Even if two molecules collide with an energy greater than the activation energy, a reaction may not take place if the molecules are not oriented properly when they collide. Consider, for example, the reaction between H2O and HCl: H2O(l) + HCl(g) ➙ H3O+(aq) + Cl‾(aq) For this reaction to take place, the molecules must collide in such a way that the H of the HCl hits the O of the water, as shown in Figure 2(a). A collision in which the Cl hits the O, as shown in Figure 2(b), cannot lead to a reaction, even if sufficient energy is available. 2 35 / 96 Reaction Rates & Chemical Equilibrium Chemistry Dr. Saeed Mohammed Ismaeel Figure 2 Molecules must be properly oriented for a reaction to take place. (a) HCl and H2O molecules are oriented so that the H of HCl collides with the O of H2O, and a reaction takes place. (b) No reaction takes place because Cl, and not H, collides with the O of H2O. The colored arrows show the path of the molecules. What Is The Relationship Between Activation Energy And Reaction Rate?: Figure 3 shows a typical energy diagram for an exothermic reaction. The products have a lower energy than the reactants; we might, therefore, expect the reaction to take place rapidly. As the curve shows, however, the reactants cannot be converted to products without the necessary activation energy. The activation energy is like a hill. If we are in a mountainous region, we may find that the only way to go from one point to another is to climb over a hill. It is the same in a chemical reaction. Even though the products may have a lower energy than the reactants, the products cannot form unless the reactants “go over the hill” or over a high pass—that is, gain the necessary activation energy. Let us look into this issue more closely. In a typical reaction, existing bonds are broken and new bonds form. For example, when H2 reacts with N2 to give NH3, six covalent bonds must break, and six new covalent bonds must form. The activation energy is inversely related to the rate of the reaction. The lower the activation energy, the faster the reaction; the higher the activation energy, the slower the reaction. The top of the hill on an energy diagram is called the transition state. When the reacting molecules reach this point, one or more original bonds are partially broken, and one or more new bonds may be in the process of formation. 3 36 / 96 Reaction Rates & Chemical Equilibrium Chemistry Dr. Saeed Mohammed Ismaeel Figure 3 Energy diagram for the exothermic reaction How We Can Change The Rate Of a Chemical Reaction?: A. Nature of the Reactants: In general, reactions that take place between ions in aqueous solution are extremely rapid, occurring almost instantaneously. Activation energies for these reactions are very low because usually no covalent bonds must be broken. As we might expect, reactions between covalent molecules, whether in aqueous solution or not, take place much more slowly. Many of these reactions require 15 min to 24 h or longer for most of the reactants to be converted to the products. Some reactions take a good deal longer, of course, but they are seldom useful. B. Concentration: Consider the following reaction: A + B ➙ C + D In most cases, the reaction rate increases when we increase the concentration of either or both reactants. For many reactions—though by no means all—a direct relationship exists between concentration and reaction rate; that is, when the concentration of a reactant is doubled, the reaction rate also doubles. This outcome is easily understandable on the basis of the collision theory. If we double the concentration of A, there are twice as many molecules of A in the same volume, so the molecules of B in that volume now collide with 4 37 / 96 Reaction Rates & Chemical Equilibrium Chemistry Dr. Saeed Mohammed Ismaeel twice as many A molecules per second than before. Given that the reaction rate depends on the number of effective collisions per second, the rate doubles. In the case where one of the reactants is a solid, the rate is affected by the surface area of the solid. For this reason, a substance in powder form reacts faster than the same substance in the form of large chunks. For reactions in the gas phase, an increase in pressure usually increases the rate. C. Temperature: In virtually all cases, reaction rates increase with increasing temperature. What causes reaction rates to increase with increasing temperature? Once again, we turn to collision theory. Here temperature has two effects: 1. When the temperature increases, molecules move more rapidly, which means that they collide more frequently. More frequent collisions mean higher reaction rates. However, this factor is much less important than the second factor. 2. A reaction between two molecules takes place only if an effective collision occurs—a collision with an energy equal to or greater than the activation energy. When the temperature increases, not only is the average speed (kinetic energy) of the molecules greater, but there is also a different distribution of speeds. The number of very fast molecules increases much more than the number with the average speed (Figure 4). As a consequence, the number of effective collisions rises even more than the total number of collisions. Not only do more collisions take place, but the percentage of collisions that have an energy greater than the activation energy also rises. This factor is mainly responsible for the sharp increase in reaction rates with increasing temperature. Figure 4 Distribution of kinetic energies (molecular velocities) at two temperatures. The kinetic energy on the x-axis designated Ea indicates the energy (molecular velocity) necessary to pass through the activation energy barrier. The shaded areas represent the fraction of molecules that have kinetic energies (molecular velocities) greater than the activation energy. 5 38 / 96 Reaction Rates & Chemical Equilibrium Chemistry Dr. Saeed Mohammed Ismaeel Why High Fever Is Dangerous ?: A sustained body temperature of 41.7°C (107°F) is invariably fatal. We can now see why a high fever is dangerous. Normal body temperature is 37°C (98.6°F), and all the many reactions in the body—including respiration, digestion, and the synthesis of various compounds—take place at that temperature. If an increase of 10°C causes the rates of most reactions to approximately double, then an increase of even 1°C makes them go significantly faster than normal. Fever is a protective mechanism, and a small increase in temperature allows the body to kill germs faster by mobilizing the immune defense mechanism. This increase must be small, however: A rise of 1°C brings the temperature to 38°C (100.4°F); a rise of 3°C brings it to 40°C (104°F). A temperature higher than 104°F increases reaction rates to the danger point. One can easily detect the increase in reaction rates when a patient has a high fever. The pulse rate increases and breathing becomes faster as the body attempts to supply increased amounts of oxygen for the accelerated reactions. A marathon runner, for example, may become overheated on a hot and humid day. After a time, perspiration can no longer cool his or her body effectively, and the runner may suffer hyperthermia or heat stroke, which, if not treated properly, can cause brain damage. The Effects of Lowering Body Temperature: Like a significant increase in body temperature, a substantial decrease in body temperature below 37°C (98.6°F) can prove harmful because reaction rates are abnormally low. It is sometimes possible to take advantage of this effect. In some heart operations, for example, it is necessary to stop the flow of oxygen to the brain for a considerable time. At 37°C (98.6°F), the brain cannot survive without oxygen for longer than about 5 min without suffering permanent damage. When the patient’s body temperature is deliberately lowered to about 28 to 30°C (82.4 to 86°F), however, the oxygen flow can be stopped for a considerable time without causing damage because reaction rates slow down. At 25.6°C (78°F), the body’s oxygen consumption is reduced by 50%. D. Presence of a Catalyst Any substance that increases the rate of a reaction without itself being used up is called a catalyst. Many catalysts are known—some that increase the rate of only one reaction and others that can affect several reactions. Although we have seen that we can speed up reactions by increasing the temperature, in some cases they remain too slow even at the highest temperatures we can conveniently reach. In other cases, it is not feasible to increase the temperature—perhaps because other, unwanted reactions would be speeded up, too. Catalysts work by allowing the reaction to take a different pathway, one with a lower activation energy. Without the catalyst, the reactants would have to get over the higher energy hill (Figure 5). The catalyst provides a lower hill. As we have seen, a lower activation energy means a higher reaction rate. 6 39 / 96 Reaction Rates & Chemical Equilibrium Chemistry Dr. Saeed Mohammed Ismaeel Heterogeneous catalyst: A catalyst in a separate phase from the reactants—for example, the solid platinum, Pt(s), in the reaction between CH2O(g) and H2(g). Homogeneous catalyst: A catalyst in the same phase as the reactants—for example, enzymes in body tissues. Figure 5 Energy diagram for a catalyzed reaction. The dashed line shows the energy curve for the uncatalyzed process. The catalyst provides an alternative pathway whose activation energy is lower. What Does It Mean To Say That a Reaction Has Reached Equilibrium ?: Many reactions are irreversible. When a piece of paper is completely burned, the products are CO2 and H2O. Anyone who takes pure CO2 and H2O and tries to make them react to give paper and oxygen will not succeed. Other reactions are reversible. A reversible reaction can be made to go in either direction. For example, if we mix carbon monoxide with water in the gas phase at a high temperature, carbon dioxide and hydrogen are produced: As the reaction proceeds, the concentrations of CO and H2O gradually decrease because both reactants are being used up. In turn, the rate of the reaction gradually decreases because it depends on the concentrations of the reactants. 7 40 / 96 Reaction Rates & Chemical Equilibrium Chemistry Dr. Saeed Mohammed Ismaeel But what is happening in the other direction? Before we added the carbon monoxide, no carbon dioxide or hydrogen was present. As soon as the forward reaction began, it produced small amounts of these substances, and we now have some CO2 and H2. These two compounds will now, of course, begin reacting with each other (the reverse reaction). At first, the reverse reaction is very slow. As the concentrations of H 2 and CO2 (produced by the forward reaction) gradually increase, the rate of the reverse reaction also gradually increases. We have a situation, then, in which the rate of the forward reaction gradually decreases, while the rate of the reverse reaction (which began at zero) gradually increases. Eventually the two rates become equal. At this point, the process is in dynamic equilibrium (or just equilibrium). What Is an Equilibrium Constant And How Do We Use It ?: Chemical equilibria can be treated by a simple mathematical expression. First, let us write the following reaction as the general equation for all reversible reactions: aA + bB cC + dD The double arrow shows that the reaction is reversible. In general, any number of substances can be present on either side. Once equilibrium is reached, the following equation is valid, where K is a constant called the equilibrium constant: When we multiply the equilibrium concentrations of the substances on the right side of the chemical equation and divide this product by the equilibrium concentrations of the substances on the left side (after raising each number to the appropriate power), we get the equilibrium constant, a number that does not change. The equilibrium constant is different for every reaction. Some reactions have a large K ; others have a small K. The equilibrium constant for a given reaction remains the same no matter what happens to the concentrations, but the same is not true for changes in temperature. The value of K, however, does change when the temperature changes. What Is Le Chatelier’s Principle?: In 1888, Henri Le Chatelier (1850–1936) put forth the statement known as Le Chatelier’s principle: If an external stress is applied to a system in equilibrium, the system reacts in such a way as to partially relieve that stress. 8 41 / 96 Reaction Rates & Chemical Equilibrium Chemistry Dr. Saeed Mohammed Ismaeel A. Addition of a Reaction Component: Suppose that the reaction between acetic acid and ethanol has reached equilibrium: H2SO4 CH3COOH + C2H5OH CH3COOC2H5 + H2O Acetic acid Ethanol Ethyl acetate This means that the reaction flask contains all four substances (plus the catalyst) and that their concentrations no longer change. We now disturb the system by adding some acetic acid. Adding CH3COOH H2SO4 CH3COOH + C2H5OH CH3COOC2H5 + H2O Acetic acid Ethanol Ethyl acetate Equilibrium shifts to formation of more products The result is that the concentration of acetic acid suddenly increases, which increases the rate of the forward reaction. As a consequence, the concentrations of the products (ethyl acetate and water) begin to increase. At the same time, the concentrations of reactants decrease. Now, an increase in the concentrations of the products causes the rate of the reverse reaction to increase, but the rate of the forward reaction is decreasing, so eventually the two rates will be equal again and a new equilibrium will be established. When that happens, the concentrations are once again constant, but they are not the same as they were before the addition of the acetic acid. The concentrations of ethyl acetate and water are higher now, and the concentration of ethanol is lower. The concentration of acetic acid is higher because we added some, but it is less than it was immediately after we made the addition. When we add more of any component to a system in equilibrium, that addition constitutes a stress. The system relieves this stress by increasing the concentrations of the components on the other side of the equilibrium equation. We say that the equilibrium shifts in the opposite direction. The addition of acetic acid, on the left side of the equation, causes the rate of the forward reaction to increase and the reaction to move toward the right: More ethyl acetate and water form, and some of the acetic acid and ethanol are used up. The same thing happens if we add ethanol. On the other hand, if we add water or ethyl acetate, the rate of the reverse reaction increases, and the reaction shifts to the left. 9 42 / 96 Reaction Rates & Chemical Equilibrium Chemistry Dr. Saeed Mohammed Ismaeel We can summarize by saying that the addition of any component causes the equilibrium to shift to the opposite side. B. Removal of a Reaction Component: It is not always as easy to remove a component from a reaction mixture as it is to add one, but there are often ways to do it. The removal of a component, or even a decrease in its concentration, lowers the corresponding reaction rate and changes the position of the equilibrium. If we remove a reactant, the reaction shifts to the left, toward the side from which the reactant was removed. If we remove a product, the reaction shifts to the right, toward the side from which the product was removed. In the case of the acetic acid–ethanol equilibrium, ethyl acetate has the lowest boiling point of the four components and can be removed by distillation. The equilibrium then shifts to that side so that more ethyl acetate is produced to compensate for the removal. The concentrations of acetic acid and ethanol decrease, and the concentration of water increases. The effect of removing a component is thus the opposite of adding one. The removal of a component causes the equilibrium to shift to the side from which the component was removed. H2SO4 Removing ethyl acetate CH3COOH + C2H5OH H2O + CH3COOC2H5 Acetic acid Ethanol Ethyl acetate Equilibrium shifts to formation of more products No matter what happens to the individual concentrations, the value of the equilibrium constant remains unchanged. C. Change in Temperature: The effect of a change in temperature on a reaction that has reached equilibrium depends on whether the reaction is exothermic (gives off heat) or endothermic (requires heat). Let us look first at an exothermic reaction: 2H2(g) + O2(g) 2H2O(l) + 137,000 cal per mol H2O If we consider heat to be a product of this reaction, then we can use Le Chatelier’s principle and the same type of reasoning as we did before. An increase in temperature means that we are adding heat. Because heat is a product, its addition pushes the equilibrium to the opposite side. We can therefore say that, if this exothermic reaction is at equilibrium and we increase the temperature, the reaction goes to the left—the concentrations of H2 and O2 increase and that of H2O decreases. This is true of all exothermic reactions. 10 43 / 96 Reaction Rates & Chemical Equilibrium Chemistry Dr. Saeed Mohammed Ismaeel An increase in temperature drives an exothermic reaction toward the reactants (to the left). A decrease in temperature drives an exothermic reaction toward the products (to the right). For an endothermic reaction, of course, the opposite is true. An increase in temperature drives an endothermic reaction toward the products (to the right). A decrease in temperature drives an endothermic reaction toward the reactants (to the left). Recall from Section 8.4 that a change in temperature changes not only the position of equilibrium but also the value of K , the equilibrium constant. D. Change in Pressure: A change in pressure influences the equilibrium only if one or more components of the reaction mixture are gases. Consider the following equilibrium reaction: N2O4(g) 2NO2(g Dinitrogen tetroxide Nitrogen dioxide In this equilibrium, we have one mole of gas as a reactant and two moles of gas as products. According to Le Chatelier’s principle, an increase in pressure shifts the equilibrium in the direction that will decrease the moles in the gas phase and thus decrease the internal pressure. In the preceding reaction, the equilibrium will shift to the left. An increase in pressure shifts the reaction toward the side with fewer moles of gas. A decrease in pressure shifts the reaction toward the side with more moles of gas. E. The Effects of a Catalyst: A catalyst increases the rate of a reaction without itself being changed. For a reversible reaction, catalysts always increase the rates of both the forward and reverse reactions to the same extent. Therefore, the addition of a catalyst has no effect on the position of equilibrium. However, adding a catalyst to a system not yet at equilibrium causes it to reach equilibrium faster than it would without the catalyst. 11 44 / 96 Solutions & Colloids Chemistry Dr. Saeed Mohammed Ismaeel SOLUTIONS & COLLOIDS INTRODUCTION: In our daily lives, however, we more frequently encounter mixtures—systems having more than one component. Air, smoke, sea water, milk, blood, and rocks, for example, are mixtures If a mixture is uniform throughout at the molecular level, we call it a homogeneous mixture or, more commonly, a solution. Air and sea water, for example, are both solutions. Cigarette smoke, milk, and blood plasma may look homogeneous, but they do not have the transparency of air or sea water. These mixtures are classified as colloidal dispersions (suspensions). What are The Most Common Types of Solutions?: When we think of a solution, we normally think of a liquid. Liquid solutions, such as sugar in water, are the most common kind, but there are also solutions that are gases or solids. In fact, all mixtures of gases are solutions. Because gas molecules are far apart from each other and much empty space separates them, two or more gases can mix with each other in any proportions. Because the mixing takes place at the molecular level, a true solution always forms; that is, there are no heterogeneous mixtures of gases. With solids, we are at the other extreme. Whenever we mix solids, we get a heterogeneous mixture. Because even microscopic pieces of solid still contain many billions of particles (molecules, ions, or atoms), there is no way to achieve mixing at the molecular level. Homogeneous mixtures of solids (or alloys), such as brass, do exist, but we make them by melting the solids, mixing the molten components, and allowing the mixture to solidify. Table 1 lists the five most common types of solutions. Examples of other types (gas in solid, liquid in gas, and so on) are also known but are much less important. When a solution consists of a solid or a gas dissolved in a liquid, the liquid is called the solvent, and the solid or gas is called the solute. A solvent may have several solutes dissolved in it, even of different types. A common example is spring water, in which gases (carbon dioxide and oxygen) and solids (salts) are dissolved in the solvent, water. When one liquid is dissolved in another, a question may arise regarding which is the solvent and which is the solute. In most cases, the one present in the greater amount is called the solvent, but no rigid rule applies. 1 45 / 96 Solutions & Colloids Chemistry Dr. Saeed Mohammed Ismaeel What Are The Distinguishing Characteristics Of Solutions?: The following are some properties of solutions: 1. The distribution of particles in a solution is uniform. Every part of the solution has exactly the same composition and properties as every other part. That, in fact, is the definition of “homogeneous.” 2. The components of a solution do not separate on standing. A solution of vinegar (acetic acid in water), for example, will never separate. 3. A solution cannot be separated into its components by filtration. Both the solvent and the solute pass through a filter paper. 4. For any given solute and solvent, it is possible to make solutions of many different compositions. For example, we can easily make a solution of 1 g of glucose in 100 g of water, or 2 g, or 6 g, or 8.7 g, or any other amount up to the solubility limit. 5. Solutions are almost always transparent. They may be colorless or colored, but we can usually see through them. Solid solutions are exceptions. 6. Solutions can be separated into pure components. Common separation methods include distillation and chromatography. The separation of a solution into its components is a physical change, not a chemical one. What Factors Affect Solubility ?: The solubility of a solid in a liquid is the maximum amount of the solid that will dissolve in a given amount of a particular solvent at a given temperature. Suppose we wish to make a solution of table salt (NaCl) in water. We take some water, add a few grams of salt, and stir. At first, we see the particles of salt suspended in the water. Soon, however, all the salt dissolves. Now let us add more salt and continue to stir. Again, the salt dissolves. Can we 2 46 / 96 Solutions & Colloids Chemistry Dr. Saeed Mohammed Ismaeel repeat this process indefinitely? The answer is no—there is a limit. The solubility of table salt is 36.2 g per 100 g of water at 30°C. If we add more salt than that amount, the excess solid will not dissolve but rather will remain suspended as long as we keep stirring; it will sink to the bottom after we stop stirring. Solubility is a physical constant, like melting point or boiling point. Each solid has a different solubility in every liquid. Some solids have a very low solubility in a particular solvent; we often call these solids insoluble. Others have a much higher solubility; we call these soluble. Even for soluble solids however, there is always a solubility limit. The same is true for gases dissolved in liquids. Different gases have different solubilities in a solvent. Some liquids are essentially insoluble in other liquids (gasoline in water), whereas others are soluble to a limit. For example, 100 g of water dissolves about 6 g of diethyl ether (another liquid). If we add more ether than that amount, we see two layers. Some liquids, however, are completely soluble in other liquids, no matter how much is present. An example is ethanol, C2H6O, and water, which form a solution no matter what quantities of each are mixed. We say that water and ethanol are miscible in all proportions. When a solvent contains all the solute it can hold at a given temperature, we call the solution saturated. Any solution containing a lesser amount of solute is unsaturated. If we add more solute to a saturated solution at constant temperature, it looks as if none of the additional solid dissolves, because the solution already holds all the solute that it can. Actually an equilibrium is at work in this situation. Some particles of the additional solute dissolve, whereas an equal quantity of dissolved solute comes out of solution. Thus, even though the concentration of dissolved solute does not change, the solute particles themselves are constantly going into and out of solution. A supersaturated solution contains more solute in the solvent than it can normally hold at a given temperature under equilibrium conditions. A supersaturated solution is not stable; when disturbed in any way, such as by stirring or shaking, the excess solute precipitates— thus the solution returns to equilibrium and becomes merely saturated. Whether a particular solute dissolves in a particular solvent depends on several factors, as discussed next. A. Nature of the Solvent and the Solute: The more similar two compounds are, the more likely that one will be soluble in the other. Here the rule is “like dissolves like.” This is not an absolute rule, but it does apply in most cases. 3 47 / 96 Solutions & Colloids Chemistry Dr. Saeed Mohammed Ismaeel In other words, polar compounds dissolve in polar solvents, and nonpolar compounds dissolve in nonpolar solvents(Polar compounds dissolve in polar compounds because the positive end of the dipole of one molecule attracts the negative end of the dipole of the other molecule ). For example, the liquids benzene (C6H6) and carbon tetrachloride (CCl4) are nonpolar compounds. They dissolve in each other, and other nonpolar materials, such as gasoline, dissolve in them. In contrast, ionic compounds such as sodium chloride (NaCl) and polar compounds such as table sugar (C12H22O11) are insoluble in these solvents. The most important polar solvent is water. We have already seen that most ionic compounds are soluble in water, as are small covalent compounds that can form hydrogen bonds with water. It is worth noting that even polar molecules are usually insoluble in water if they cannot either react with water or form hydrogen bonds with water molecules. B. Temperature: For most solids and liquids that dissolve in liquids, the rule is that solubility increases with increasing temperature. Sometimes the increase is great; at other times it is moderate. For a few substances, solubility even decreases with increasing temperature (Figure 1). For example, the solubility of glycine, H2N CH2COOH, a white, crystalline solid and a polar building block of proteins, is 52.8 g in 100 g of water at 80°C but only 33.2 g at 30°C. If, for instance, we prepare a saturated solution of glycine in 100 g of water at 80°C, it will hold 52.8 g of glycine. If we then allow it to