Control of Breathing Lecture Outline PDF
Document Details
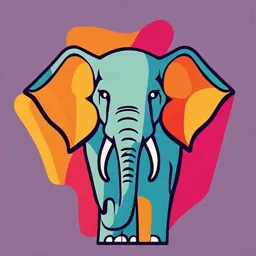
Uploaded by SuperiorPsaltery
Tags
Summary
This document is a lecture outline on the control of breathing, covering various aspects like respiratory patterns, neural mechanisms, and responses to different factors. It includes objectives, references, and potentially discusses different types of breathing patterns.
Full Transcript
Control of Breathing Lecture Outline I. Control of Breathing II. Respiratory Patterns III. Generation of Spontaneous Rhythmicity: Medullary and Pontine Respiratory Groups IV. Reflex Mechanisms of Respiratory Control V. Response to CO2, H+, O2, and Exercise 1 Control of Breathing Objectives 1. 2. 3....
Control of Breathing Lecture Outline I. Control of Breathing II. Respiratory Patterns III. Generation of Spontaneous Rhythmicity: Medullary and Pontine Respiratory Groups IV. Reflex Mechanisms of Respiratory Control V. Response to CO2, H+, O2, and Exercise 1 Control of Breathing Objectives 1. 2. 3. 4. 5. 6. 7. 8. 9. 10. 11. Describe the autonomic and voluntary control of respiration Identify the components that maintain respiratory homeostasis Identify both normal and abnormal respiratory patterns Identify the centers that generate spontaneous rhythmicity of breathing Describe the groups of neurons that effect inspiration and expiration Describe the other centers in the brainstem that may influence the spontaneous rhythmicity of breathing Lists the cardiopulmonary and other reflexes that influence the breathing pattern States the ability of the brain cortex to override the normal pattern of inspiration and expiration temporarily Describes the effects of alterations in oxygen, carbon dioxide, and hydrogen ions on the control of breathing Describes the sensors of the respiratory system for oxygen, carbon dioxide, and hydron ion concentration Explain the hyperpnea of exercise 2 References Assigned reading from your text: Levitzky Chapter 9 Along with images/information from: Boron (pre-Botzinger complex) Ganong (pre-Botzinger complex) Apex Anesthesia (bound to the NCE bibliography for their content) 3 I. Control of Breathing 4 Control of Breathing Breathing is under both involuntary control (automatically driven by pacemaker cells located in the medulla) and voluntary control (by the cerebral cortex) Neural control of ventilation: Chemical control of ventilation: Central pattern generator in the medulla and pons initiate rhythmic breathing Chemical regulation adjusts ventilation to maintain PCO2, PO2, and pH through both central and peripheral chemoreceptors Inputs come from the lung, airways, proprioceptors, and visceral reflexes Respiratory minute volume is proportional to the metabolic rate; the link between metabolism and ventilation is CO2 (not O2). Integration of Ventilation q Respiratory homeostasis: Maintains a balance of CO2 (mainly) and O2 Minimizes work of breathing Prevents overinflation of the lung Responds to irritants 6 Control of Respiration q Spontaneous respiration is produced by rhythmic discharge of motor neurons that innervate the respiratory muscles – – Discharge is totally dependent on nerve impulses Automatic (involuntary) control is driven by pacemaker cells in the medulla These cells activate motor neurons in the cervical and thoracic spinal cord that innervate muscles of respiration Breathing stops if the spinal cord is transected above the origin of the phrenic nerves Spontaneous respiration is regulated by alterations in arterial PO2, PCO2, and H+ concentration – – Changes in gases and metabolites Rate of ventilation is adjusted to metabolic demand q Voluntary control system is located in the cerebral cortex and sends impulses to the respiratory motor neurons via the corticospinal tracts Diagram of Overall Respiratory Control System q Negative feedback maintains PCO2, PO2, and pH From Ganong Figure 36-1 The volunteer control center is in cerebral cortex and the automatic control centers are in the medulla and pons. The nerve impulses or electrical signals from the respiratory neurons in these centers regulate the activity of respiratory muscles (including diaphragm) and thus the rate and depth of breathing (ventilation). The mechanoreceptors and chemoreceptors (central and peripheral) then sense the physical changes in the lungs and chest wall and the chemical changes in the blood (eg arterial PCO, arterial PO2, and [H+]) to further adjust the control of breathing. Components of Respiratory Homeostasis Sensors transduce a stimulus – Chemoreceptors sense changes in CO2, H+, and O2 – Send input to medulla and pons to integrate output to muscles Central controller integrates input – Neurons that generate the respiratory rhythm are in the medulla Initiate and maintain act of breathing Alter the rate and depth of breathing – The pons and other areas of the brain modulate the rate and depth of respiratory output Effectors respond – Respiratory muscles include the diaphragm, intercostals, accessory muscles – Reciprocal innervation orchestrates movement Afferent Efferent Homeostasis- Sensors q Sensors (afferent fibers) provide input to influence breathing Chemical control of respiration – Central (medullary) chemoreceptors Monitor the H+ concentration of CSF CO2 easily penetrates membranes – H+ and HCO3+ penetrate slowly – – Peripheral chemoreceptors – carotid and aortic bodies CO2 hydrated, dissociates, and H+ % rises Sense O2 and H+ Nonchemical controls affect breathing in particular situations – Vagal afferents from lung and airway receptors are responsible for inflation/deflation reflexes – – – Myelinated – slowly adapting fibers (smooth muscle) and rapidly adapting fibers (coughing/sneezing reflexes) Unmyelinated C fibers- transmit tickling sensations that lead to coughing (the response) – Pulmonary- juxtacapillary receptors – Bronchial Afferents from proprioceptors respond to exercise Afferents from the pons, hypothalamus, and limbic system (pain and emotional stimuli) Afferents from baroreceptors: arterial, atrial, ventricular, and pulmonary Homeostasis- Controller q Respiratory center is located in the reticular activating system in the medulla and pons Key groups of nuclei work together to Initiate breathing and ventilation Modulate the rate and depth of breathing q The centers are as follows: Medullary respiratory center is the “central controller”- aka “central pattern generator” (CPG): Dorsal respiratory group Ventral respiratory group Pre-Botzinger group located in VRG Pontine respiratory centers (modulate only) Pneumotaxic center Apneustic center 11 Homeostasis- Effectors q Axons from brain descend in spinal cord white matter to activate the muscles of respiration Inspiratory and expiratory fibers are separated in the SC SC Interneurons inhibit antagonistic respiratory muscle groups q Reciprocal innervation to respiratory muscles Motor neurons supplying expiratory muscles are inactive when motor neurons supplying inspiratory muscles are active and vice versa (with the order changed) 12 II. Respiratory Patterns 13 Respiratory Patterns 1/3 q Respiratory patterns- normal and abnormal- occur for a variety of reasons Eupnea Normal breathing Sigh Larger than normal breath that occurs ~ 6x /hr to counteract atelectasis (open alveoli) Yawn- an exaggerated sigh to TLC that increases venous return and prepares for or responds to atelectasis. eg preparing for your nap in class Apneusis Prolonged inspirations separated by brief expirationsrarely seen in humans Vagal breathing Slow, deep inspirations caused by interruption of vagal input to the brainstem 14 Respiratory Patterns 2/3 q Respiratory patterns occur for a variety of reasons Cheyne-Stokes respiration Benign pattern; cycles of gradual increase in tidal volume followed by a gradual decrease in tidal volume then a period of apnea. Seen in CHF or healthy subject sleeping at altitude Gasping Maximal brief inspiratory efforts separated by long periods expiration. Seen in severe anoxia and cardiac arrest Cluster and ataxic breathing r/t brainstem lesions Where in the brainstem? 15 Respiratory Patterns 3/3 Other patterns of breathing: Tachypnea- increased respiratory rate Apnea- Cessation of respiration Hyperventilation- Increased alveolar ventilation Hypoventilation- Decreased alveolar ventilation Kussmaul breathing- Extremely deep, rapid breathing seen in metabolic acidosis (eg DKA) 16 III. Generation of Spontaneous Rhythmicity: The Medullary and Pontine Respiratory Groups 17 Anatomical Terminology- from Moore p 8 q Below the diencephalon Ventral (anterior) denotes the front surface of the body Dorsal (posterior) denotes the back surface of the body or nearer to the back q At the junction of the brainstem and diencephalon: Rostral (Latin for beak) is used instead of anterior when describing parts of the brain toward the nose Caudal (Latin for tail) is used to indicate the back of the brain 18 Observations from Galen Identified the Origin of Respiration q From Boron Figure 32-2 - Galen was the first to determine the location of the respiratory controller. As a physician for gladiators in the Greek city of Pergamon, he observed that breathing stopped after a sword blow to the high cervical spine. A similar blow to the lower cervical spine paralyzed the arms and legs, but allowed respiration to continue. He reproduced these lesions in live animals and correctly concluded that the brain sends information via the mid-cervical spinal cord to the diaphragm. (Last half 2nd century) 19 Neurons That Control Ventilation q Neurons that control ventilation are located in the medulla The dorsal respiratory group (DRG) processes sensory input and contains primarily inspiratory neurons The ventral respiratory group (VRG) is primarily motor and contains both inspiratory and expiratory neurons q Pontine respiratory groups of neurons modulate respiratory output Pneumotaxic center inhibits apneustic center and promotes exhalation Apneustic center stimulates the DRG to promote inhalation Medullary Neurons are the Central Pattern Generator- DRG q The dorsal respiratory group (DRG) serves an afferent role in respiration Most of its neurons are located in and around the the nucleus tractus solitarius (NTS)à NTS receives sensory input from all viscera of the thorax/abdomen Integrates sensory input (afferent) via CN IX & X and other receptors CN IX and X supply the pharynx and larynx The DRG modifies its activities in response to input from chemoreceptors and baroreceptors Contains interneurons and premotor neurons Contains primarily inspiratory neurons Outflow to diaphragm and external intercostals q Composed of three populations of cells: I a and I b (inspiratory) and P-cells (expiratory): I a cells Increase activity if inflation suppressed I b cells Decrease activity if lung inflation is suppressed Involved in the Hering-Breuer reflex Pump (P) cells Interneurons that relay information from pulmonary stretch receptors 21 Medullary Neurons are the Central Pattern Generator- VRG q The ventral respiratory group (VRG) serves an efferent role in respiration Lies ventral to the DRG and is longer VRG Is primarily motor and Contains both inspiratory and expiratory neurons Sensory information to the VRG comes indirectly from the DRG Contains interneurons, premotor neurons, and somatic motor neurons Motor neurons innervate muscles of the pharynx, larynx, thorax, and abdomen q Three regions: Bötzinger complex contains interneurons that drive expiration Rostral VRG Inhibits inspiratory cells in DRG and phrenic Pre-Bötzinger complex initiates inspiration Essential pacemaker of respiratory rhythm Integrates inputs of both DRG and VRG Nucleus ambiguus – patency of the upper airway Nucleus para-ambiguails – contralateral inspiratory muscles Caudal VRG contains expiratory premotor neurons Travel down spinal cord to innervate accessory muscles of expiration Nucleus retroambigualis – contralateral expiratory muscles 22 Respiratory-Related Neurons (RRN) are Inspiratory or Expiratory q DRG and VRG contain neurons that fire more frequently during either inspiration or expiration Inspiratory neurons of the DRG Control lower motor neurons innervating the external intercostals and diaphragm These function in every respiratory cycle VRG has both inspiratory and expiratory centers Function only when breathing demands an increase Accessory respiratory muscles become involved pre-Botzinger complex is essential to all forms of breathing (pacemaker) 23 Events of Quiet and Forced Inhalation and Exhalation From Visual Anatomy and Physiology 2nd ed. Martini/Ober/Nath/Bartholomew/Petti 24 Transection of Brainstem/Spinal Cord and Modulation of Ventilation q Spirometry after brainstem transections- with and without vagus nerve transection I - Above the pons With vagi intact- no effect on breathing rate or depth With vagi cut- TV increased and RR decreased (normal balance) Conclusion- pontine respiratory groups are under influence of vagii II- Mid pons With vagi intact- No apneusis With vagi cut- Apneusis occurs Conclusion- vagus nerves inhibit the apneustic center When vagi intact, apneustic center integrates information III- Pontomedullary transection With vagi intact- gasping, irregular pattern With vagii cut- More irregular since only medullary centers intact Conclusion- Pontine and vagal inputs needed for regular breathing pattern IV- Spinomedullary Death- medullary centers responsible for initiating respiration are not able to stimulate breathing movements 25 Pontine Respiratory Groups q Two pontine respiratory groups (PRG) modulate the DRG output Apneustic center promotes inspiration Located in caudal pons Promotes inspiration by stimulating DRG During forced breathing, apneustic centers adjust the degree of stimulation in response to sensory information from vagii Prevents “switching off” inspiratory signal Pneumotaxic center (rostral pons) inhibits inspiration Located in rostral pons Inhibits the apneustic centers and promote passive or active exhalation An increase in pneumotaxic output quickens the pace of respiration by shortening the duration of each inhalation- triggers the end of inspiration A decrease in pneumotaxic output slows the respiratory pace but increases depth of respiration Stimulation plays a role in switching between inspiration and expiration IV. Reflex Mechanisms of Respiratory Control 27 Lung and Airway Receptors q Lung and airway receptors send signals to modulate breathing Pulmonary stretch receptors- (eg receptors for Herring Breuer inflation reflex) – Lie within airway smooth muscle and are stimulated by lung expansion – Prevents overexpansion of lungs during forced breathing Irritant receptors – Lie between airway epithelial cells – Stimulated by noxious gases, cigarette smoke, inhaled dusts, cold air. – Travel via CN X – Induces bronchoconstriction and hyperpnea – May play a role in bronchoconstriction of asthma for similar reasons J receptors: “juxta-capillary” – In alveolar walls close to pulmonary capillaries – Respond quickly to irritants and pulmonary vascular congestion – Stimulates tachypnea and feelings of dyspnea – Pass up CN X and can result in rapid, shallow breathing. Intense stimulation > apnea – An embolism in proximal vessels decreases J receptor stimulation and respiratory rate Inflation and Deflation Reflexes q Hering-Breuer inflation reflex prevents overexpansion Stretch receptors located in the smooth muscles of bronchi and bronchiolar walls Transmit signals via CNX to the DRG, VRG & apneustic center when lungs overstretched Respiratory muscles are inhibited by the respiratory rhythmicity centers and inhalation stop Expiratory centers of VRG are stimulated and forced exhalation begins q Hering-Breuer deflation reflex inhibits expiratory centers and stimulates inspiratory centers when lungs are deflating Decompression sensors in alveolar walls are stimulated with abrupt deflation Expiratory centers of the VRG are inhibited until exhalation stops Forced inhalation then begins with activation of the DRG and inspiratory centers of VRG May occur with pneumothorax 29 Other Influences of Respiration q Pain Somatic muscle and joint pain generally causes hyperpnea Reflexes originate from proprioceptors (muscles spindles and golgi tendon organs) Ascend via the spinal cord to respiratory centers during periods of activity Visceral pain generally causes apnea or decreased ventilation q Afferents from the hypothalamus, and limbic system transmit emotional stimuli Emotions such as anxiety may increase ventilation q Body temperature Increased body temperature stimulates ventilation Becomes a means for elimination of heat during exercise Cold temperature causes deep inspiration q Reticular activating system (RAS) Respiratory centers are part of the RAS Greatest functional activity occurs during wakefulness and is depressed during sleep During sleep PO2 decreases and PCO2 increases 30 V. Response to CO2, H+, O2, and Exercise 31 CO2 is The Most Potent Naturally Occurring Stimulus For Respiration q CO2 easily crosses the BBB and enters CSF [H+] cannot easily cross the barrier CO2 hydrates and reacts H+ ions stimulate chemoreceptors directly An increase of 2 mmHg PCO2 in CSF can indirectly stimulate these receptors 32 CO2 Is Determined by Alveolar Ventilation q Central chemoreceptors respond to changes in PaCO2 Located superficially in ventral-lateral medulla Exposed to CSF- BBB separates arterial blood and CSF Send afferent nerve impulses to the DRG Changes of the pH in CSF can profoundly effect nervous tissue of the brain: Acidosis depresses CNS activity Alkalosis causes hyper-excitability of CNS Very sensitive to slight increases in PCO2 via H+ ions q Alveolar ventilation is the most important factor in decreasing CO2 Stimulation of medullary chemoreceptors increases VT before frequency TV increases alveolar ventilation more than frequency Hyperventilation of an anesthetized patient can cause apnea indirectly through reduction of CSF PCO2 Hypoventilation and apnea will increase CO2 33 H+ Ions Stimulate The Central Chemoreceptors Directly q CSF is a weaker buffer than blood plasma Blood plasma contains 6-8 g protein/100ml CSF contains 15-45 mg protein/100 ml CSF CO2 + H2O à H2CO3 à H+ + HCO3- Occurs more readily in CSF than plasma Increased [H+]csf stimulates medullary chemoreceptors àDRGà VA Increased ventilation decreases PCO2 of the bloodà decreased PCO2 of the CSF Carbonic acid reaction in CSF reverses H+ + HCO3- à H2CO3 à H2O + CO2 CO2 is exhaledà [H+]csf decreasedà reduces stimulation of central chemoreceptors Medullary chemoreceptors can adapt to prolonged exposure to high PCO2 (over 1-2 days) Due to blood pH alterations made by the kidneys, HCO3- ions slowly diffuse through the BBB and into the CSF to neutralize [H+] Medullary chemoreceptors do not respond to hypoxia 34 Peripheral Chemoreceptors q Carotid bodies – monitors hypoxemia (PaO2 < 60 mmHg) Small masses of tissue located at the bifurcation of the common carotid artery Sensory information from carotid bodies is sent over Hering’s (sinus) nerve that joins the glossopharyngeal nerve (CN IX) and transmits impulses to the DRG q Aortic bodies – monitors arterial BP Small masses of tissue in the transverse aortic arch Sensory information sent via CN X to the DRG 35 Peripheral Chemoreceptors Monitor PO2 q Both carotid and aortic bodies have a large blood flow/gm tissue Continuously exposed to the saturated PO2 of arterial blood Factors that stimulate peripheral chemoreceptors: Change in PaO2 Change in PaCO2 Change in H+art Decreased PO2 in chemoreceptor tissue may be due to: Decreased blood flow or Decreased PaO2 Requires a significant decrease in PaO2 (~ 50 mmHg) Only peripheral chemoreceptors respond to changes in O2 (carotid>aortic) They respond to PaO2; not to changes in SaO2 or CaO2 A decrease in blood O2 content may not stimulate an increase in ventilation eg Carbon monoxide poisoning not detected Blood content of O2 is low because of preferential binding Of CO to Hb Peripheral chemoreceptors obtain adequate O2 from O2 dissolved in plasma 36 Increase in PaCO2 - Hypercapnia q It takes an increase of at least 10 mmHg PaCO2 above normal ~50 mmHg PaCO2 or greater coupled with an increased [H+] arterial blood to stimulate peripheral chemoreceptors that in turn stimulate an increase in ventilation The direct stimulus is probably [H+] in presence of a high PaCO2 A combination of hypercapnia and hypoxemia have a synergistic effect on VA 37 CO2 Response Curves 38 Acidosis q Large increase in [H+]art. Blood à a pH change 0.1- 0.2 Unitsà Acidosis The most likely reason for an increase in ventilation due to an increase in [H+] art. Blood is governed by a response from peripheral chemoreceptors Example: increase in non-volatile acid in arterial blood Diabetic ketoacidosis and Kussmaul breathing These acids do not cross the BBB Blood gas data probably shows: Normal PaO2 Decreased pH and Decreased PaCO2 Response to acidosis must be due to stimulation of peripheral chemoreceptors Peripheral chemoreceptors: Do not adapt to decreased PaO2 Will adapt to increased PaCO2 39 During Exercise q Increase in ventilation during exercise referred to as hyperpnea There are essentially no changes in PaO2 nor PaCO2 during exercise There may be a slight rise in PaO2 in moderate exercise and Or a slight decrease in PaO2 during vigorous exercise q Exercise hyperpnea is not driven by changes in PaO2 or PaCO2 levels Hyperpnea of exercise is probably due to multiple factors Cerebral cortex sends impulses to muscles and respiratory centers Proprioceptors send sensory impulses to cerebellum and to respiratory centers Increase in body temperature causes a later increase in ventilation Metabolic wastes other than CO2 may contribute to increased ventilation 40 1. The main respiratory control neurons: A. Send out regular bursts of impulses to expiratory muscles during quiet respiration B. Are unaffected by stimulation of pain receptors C. Are located in the pons D. Send our regular bursts of impulses to inspiratory muscles during quiet respiration 2. Intravenous lactic acid increases ventilation. The receptors responsible for this effect are located in the: A. Medulla oblongata B. Carotid bodies C. Lung parenchyma D. Aortic baroreceptors E. Trachea and large bronchi 3. Spontaneous respiration ceases after A. Transection of the brainstem above the pons B. Transection of brainstem at the caudal end of the medulla C. Bilateral vagotomy D. Bilateral vagotomy combined with transection of the brainstem at the superior border of the pons E. Transection of the spinal cord at the level of the first thoracic segment 4. The following physiological events that occur in vivo are listed in random order: 1. Decreased CSF pH 2. Increased arterial PCO2 3. Increased CSF PCO2 4. Stimulation of medullary chemoreceptors 5. Increased alveolar PCO2 Which of the following is the usual sequence in which these events occur when they affect respiration? A. 1,2,3,4,5 B. 4,1,3,2,5 C. 3,4,5,1,2 D. 5,2,3,1,4 E. 5,3,2,4,1 5. Injection of a drug that stimulates the carotid bodies would be expected to result in? A. A decrease in the pH of arterial blood B. A decrease in the PCO2 of arterial blood C. An increase in the HCO3- concentration of arterial blood D. An increase in plasma chloride 41