Reabsorption and Secretion in Tubules and Collecting Ducts PDF
Document Details
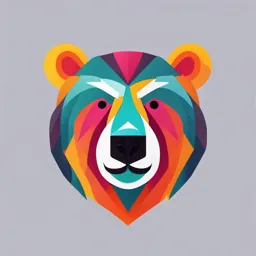
Uploaded by IndividualizedCactus
Tags
Summary
This document discusses reabsorption and secretion in the kidney's tubules and collecting ducts. It details the processes of tubular reabsorption and secretion, including the roles of transport proteins and the transport maximum. Key substances like glucose and proteins and their handling in the nephron are also examined.
Full Transcript
Page 965 24.6 Reabsorption and Secretion in Tubules and Collecting Ducts Tubular fluid flows through the proximal convoluted tubule, nephron loop, and distal convoluted tubule, then into collecting tubules and collecting ducts, as described in section 24.4b. Substances are reabsorbed when they move...
Page 965 24.6 Reabsorption and Secretion in Tubules and Collecting Ducts Tubular fluid flows through the proximal convoluted tubule, nephron loop, and distal convoluted tubule, then into collecting tubules and collecting ducts, as described in section 24.4b. Substances are reabsorbed when they move from the tubular fluid back into the blood through tubular reabsorption. Additionally, some substances that were not initially filtered at the glomerulus are eliminated from the blood, as they are moved from the blood into the tubular fluid through tubular secretion. Here we provide an overview of transport processes and consider transport maximum and renal threshold. We then consider the details of the substances reabsorbed and secreted. These are organized as follows: Substances reabsorbed completely Substances with regulated reabsorption (meaning that some, but not all, of the substance is reabsorbed) Substances eliminated as waste products Finally, we describe the establishment of a concentration gradient in the interstitial fluid, which drives the reabsorption of water controlled by antidiuretic hormone (ADH). Page 966 Page 967 24.6a Overview of Transport Processes LEARNING OBJECTIVE 31. Explain the primary anatomic structures and physiologic conditions that affect tubular reabsorption and secretion. This section provides a general overview of important anatomic structures and physiologic conditions in the kidney that influence reabsorption and secretion of substances. Please refer to figure 24.16 as you read through the following description: The barrier that a substance must cross is the simple epithelium of the tubule wall. Substances can either pass between the epithelial cells of the tubular wall by paracellular (păr′a-sel′yū-lăr) transport or, more commonly, move through the epithelial cells by transcellular (trănz′sel′yū-lăr; trans = across) transport. During transcellular transport, a substance must cross two plasma membranes: the luminal membrane that is in contact with tubular fluid and the basolateral membrane that rests on the basement membrane. The order in which the substance crosses these membranes depends upon whether it is being reabsorbed or secreted. Different transport proteins ( table 24.1) are embedded within the two membranes. They control the movement of various substances using membrane transport processes that include simple or facilitated diffusion, osmosis, primary and secondary active transport, and vesicular transport (see section 4.3). Peritubular capillaries have both low hydrostatic pressure (8 mm Hg), because of the loss of fluid during filtration at the glomerulus, and high colloid osmotic (oncotic) pressure (>30 mm Hg) exerted by protein, because most proteins remain in the blood during filtration. These two important properties facilitate reabsorption of substances from the filtrate within the renal tubule into the peritubular capillaries through bulk flow (see section 20.3b). Figure 24.16 Convoluted Tubules and Peritubular Capillaries. This segment of the proximal convoluted tubule and peritubular capillaries illustrates both the anatomic structures and physiologic conditions that influence tubular reabsorption and tubular secretion. Concept Overview Physiology Interactives Table 24.1 Pumps Transport Proteins in Cells of the Renal Tubule Carriers (Porters) Na+/K+ ATPase Glucose uniporter Channels Na+ channels H+ ATPase Na+/glucose symporter K+ channels Ca2+ ATPase Na+/HCO3– symporter Aquaporins (H2O channels) Na+/H+ antiporter Cl–/HCO3– antiporter Na+/K+/2 CI– symporter Page 968 INTEGRATE LEARNING STRATEGY 24.4 Movement of filtrate and tubular fluid through the nephron is analogous to movement of items along a conveyor belt. The fluid’s path is like the conveyer belt, and substances are first loaded onto the beginning of the conveyor belt (filtration). Then, some of these substances are retrieved from the conveyor belt (tubular reabsorption). Additional substances not originally filtered can be added to the conveyor belt along its length (tubular secretion). Whatever is on the conveyor belt at the end represents the substances that make up urine. Jaak Nilson/Spaces Images/Blend Images LLC Although reabsorption and secretion of substances occur along the entire length of the nephron tubule, collecting tubules, and collecting ducts, most reabsorption occurs in the proximal convoluted tubule, where the uptake processes are aided by the extensive microvilli on the cells’ luminal surfaces that increase their surface area (see section 24.3a). WHAT DID YOU LEARN? 24 What are the significant anatomic and physiologic factors that influence tubular reabsorption and tubular secretion? 24.6b Transport Maximum and Renal Threshold LEARNING OBJECTIVE 32. Define transport maximum and renal threshold. The transport maximum (Tm) is the maximum amount of a substance that can be reabsorbed (or secreted) across the tubule epithelium in a given period of time (i.e., the substance’s rate of movement). This maximum is dependent upon the number of the transport proteins in the epithelial cell membrane specific for the substance. For example, the Tm for glucose reabsorption by the glucose transport proteins is approximately 375 milligrams per minute (mg/min). As long as the tubular fluid contains no more than this amount of glucose passing through a region of the renal tubule every minute, all of the glucose will be reabsorbed. If the tubular fluid contains more than this amount, then the transport proteins are saturated, and cannot move any additional substance. Thus, the excess glucose is excreted in the urine. In comparison, the renal threshold is the maximum plasma concentration of a substance that can be transported in the blood without the substance being excreted in the urine. Thus, renal threshold is the maximum plasma concentration value whereby the transport maximum is not exceeded. Consequently, the substance does not appear in the urine. For example, the renal threshold for glucose in blood plasma is 300 milligrams per deciliter (mg/dL). Above this concentration, the substance is so concentrated in the plasma, and thus in the tubular fluid following filtration, that the transport proteins are saturated. The Tm is exceeded, and the substance remains within the tubular fluid and is excreted in the urine. INTEGRATE CLINICAL VIEW 24.3 Glucosuria Glucosuria (or glycosuria) is the excretion of glucose in urine. This occurs when the plasma glucose concentration exceeds approximately 300 milligrams per deciliter (mg/dL) and the transport maximum (Tm) rate for glucose of 375 milligrams per minute (mg/min) has been exceeded. Glucose molecules act as an osmotic diuretic, pulling water into the tubular fluid and causing additional loss of fluid in the urine. Glucosuria, along with frequent urination and thirst, are classical signs of diabetes mellitus (see Clinical View 17.8: “Conditions Resulting in Abnormal Blood Glucose Levels”). WHAT DO YOU THINK? 4 Explain why increased urine production and dehydration are symptoms of diabetes mellitus. WHAT DID YOU LEARN? 25 What is the transport maximum of a substance? How is it different from the renal threshold of the substance? 24.6c Substances Reabsorbed Completely LEARNING OBJECTIVES 33. Explain the reabsorption of nutrients such as glucose. 34. Describe the process by which protein is transported out of the filtrate and into the blood. Some substances are not normal components of urine because, although they are filtered, 100% of the substance is reabsorbed (or transported) from the tubular fluid back into the blood. The location for this reabsorption typically is in the proximal convoluted tubule. Two major types of substances fall into this category: nutrients (e.g., glucose, amino acids, lactate) and the small amount of filtered plasma proteins. Nutrient Reabsorption Nutrients are normally reabsorbed completely in the proximal convoluted tubule where each nutrient has its own specific transport proteins. We use glucose as an example to describe how nutrients may be reabsorbed completely. See figure 24.17 as your read this section. Figure 24.17 Glucose Reabsorption. Glucose reabsorption occurs in the proximal convoluted tubule. In a healthy individual, 100% of the glucose is reabsorbed. Glucose is transported (1) across the luminal membrane against its concentration gradient—this occurs by secondary active transport via Na+/glucose symporters— and (2) across the basolateral membrane down its concentration gradient by facilitated diffusion via glucose uniporters. Watch Video: Cotransport: Symport and Antiport Glucose concentration is relatively high inside the tubule cell and relatively low within both the tubular fluid and interstitial fluid. Glucose is first moved into the tubule cell across the luminal membrane by Na+/glucose symporter proteins. Kinetic energy from Na+ moving down its concentration gradient (see section 3.1a) into the tubule cell is used to move glucose up its concentration gradient into the tubule cell by secondary active transport (see section 4.3c). Glucose is then moved across the basolateral membrane by glucose uniporters (carriers) out of the tubule cell down its concentration gradient via facilitated diffusion. Glucose ultimately is returned to the blood in the peritubular capillaries. As with many other substances that rely on membrane transport proteins, there is a maximum amount of glucose that can be reabsorbed per unit time (see Clinical View 24.3: “Glucosuria”). (Note that the Na+ gradient, which is established by Na+/K+ pumps, is discussed in section 24.6d.) Page 969 Reclaiming Small Amounts of Filtered Protein Although most proteins are not freely filtered in the glomerulus because of their relatively large size and negative charge, some small and medium-sized proteins, such as insulin and angiotensin, and limited amounts of large proteins (e.g., about 0.02% of albumin) may appear in the filtrate. Protein is transported from the tubular fluid in the proximal convoluted tubule back into the blood so as not to be excreted in the urine. See read this section. figure 24.18 as you Figure 24.18 Reclaiming Filtered Protein. The small amounts of filtered protein are brought into the tubular cell across the luminal membrane by endocytosis. The vesicle containing protein fuses with a lysosome and the protein is digested into amino acids. The amino acids are released at the basolateral membrane by exocytosis. *Some protein is first digested into amino acids by plasma membrane enzymes (peptidases) before being brought into the tubular cell. We use the general term transported here (instead of reabsorbed) because the proteins actually undergo structural changes while being reabsorbed. Protein is moved across the luminal membrane by endocytosis (either pinocytosis or receptor-mediated endocytosis; see section 4.3c). Lysosomes in these tubule cells then digest the proteins into their amino acid building blocks. These amino acids are released by exocytosis across the basolateral membrane and then enter the blood. Very small proteins, such as angiotensin II, are degraded by peptidases within the luminal membrane and the amino acids are absorbed directly into the tubule cell. Thus, proteins are first degraded into amino acids, which are then absorbed into the blood. WHAT DO YOU THINK? 5 What happens to blood concentration of plasma proteins in an individual with renal disease that results in either (a) damage to the filtration membrane or (b) decreased filtration at the filtration membrane? Page 970 Given the kidney’s normal function in not filtering most plasma proteins and degrading the plasma proteins that are filtered, what are the clinical implications of renal disease that alter these processes? First, consider the consequences if the renal disease results in higher than normal filtration of plasma proteins (e.g., damage to the filtration membrane or high systemic blood pressure). These abnormal changes result in saturation of the cellular structures of the tubules involved in protein uptake, and protein is lost in the urine (a condition called proteinuria). Consequently, blood concentration of all plasma proteins decreases. Second, consider the consequences if the renal disease results in lower than normal filtration of plasma proteins (e.g., changes that accompany chronic kidney disease to varying degrees, depending upon the state of the disease). These abnormal changes result in a decrease in the filtration of small plasma proteins and their degradation within the kidney tubules. Consequently, blood concentration of these plasma proteins increases. Plasma protein levels that are either too low or too high result in unwanted physiologic outcomes (e.g., too low of plasma proteins can cause edema; see section 25.2b). WHAT DID YOU LEARN? 26 Diagram how glucose is reabsorbed across the two membranes of the tubule cells. 27 Why are proteins said to be transported rather than simply reabsorbed in the proximal convoluted tubule? 24.6d Substances with Regulated Reabsorption LEARNING OBJECTIVES 35. List substances for which reabsorption is regulated. 36. Describe how the reabsorption of sodium, potassium, calcium, and phosphate occurs. 37. Describe the reabsorption of water, and compare how it is regulated by the actions of aldosterone and antidiuretic hormone. 38. Describe how pH is regulated by intercalated cells. Some substances (e.g., nutrients) are recovered completely from the tubular fluid, whereas others (e.g., ions, water) are not completely reabsorbed, resulting in a variable and often small percentage of that substance being excreted into the urine. By varying the amount of a substance excreted, the nephron has a substantial role in regulating the blood level of that substance. A number of substances fall into the category of undergoing regulated reabsorption, including Na+, water, K+, HCO3−, and Ca2+. We will see that the reabsorption of Na+ plays a pivotal role in the reabsorption of many of these other substances. WHAT DO YOU THINK? 6 If a substance in the blood is filtered, and not all of it is completely reabsorbed back into the blood, does its level in the blood increase, decrease, or stay the same? Explain. + Sodium (Na+) Reabsorption The amount of sodium (Na+) reabsorbed from the tubular fluid can vary from 98% to 100%. Unlike glucose and other nutrients, Na+ is reabsorbed along the entire length of the nephron tubule, collecting tubules and collecting ducts, with the majority of the Na+ (about 65%) reabsorbed in the proximal convoluted tubule ( figure 24.19a). Approximately 25% is reabsorbed in the nephron loop, about 5% in the DCT, and a varying (and regulated) amount in the collecting tubule and collecting duct. Figure 24.19 Sodium (Na+) Reabsorption (a) Na+ reabsorption occurs along most of the length of the renal tubule. (b) The majority of Na+ is reabsorbed in the proximal convoluted tubule. Na+ moves across the luminal membrane down its concentration gradient by facilitated diffusion via various types of Na+ transport proteins, and is moved across the basolateral membrane against its concentration gradient by Na+/K+ pumps. (c) The amount of Na+ excreted in the urine is regulated in the collecting tubules and collecting ducts by hormones binding to principal cells. Aldosterone binds to receptors within principal cells, increasing the number of both Na+ channels and Na+/K+ pumps. The net effect is that additional Na+ is reabsorbed, water follows by osmosis, and additional K+ is secreted. figure 24.19b illustrates Na+ reabsorption in the PCT. Sodium concentration is relatively low inside the tubule cell and relatively high within both the tubular fluid and interstitial fluid. (The Na+ concentration gradient is established by Na+/K+ pumps, as described shortly.) Thus, Na+ moves passively by facilitated diffusion down its concentration gradient across the luminal membrane into the tubular cell of the PCT. The specific type of transport protein involved in the movement across the luminal membrane varies in the different sections of the renal tubule. INTEGRATE LEARNING STRATEGY 24.5 Use the phrase “Al likes salt” to remember that aldosterone helps our bodies retain sodium. Also, note the word roots of atrial natriuretic peptide, which causes sodium (Na+, from Latin natrium) to be excreted in the urine (-uretic). Embedded in the basolateral membrane are Na+/K+ pumps (see section 4.3c). These + pumps move Na from within the tubular cell into the interstitial fluid, whereas K+ is moved from the interstitial fluid into the tubular cell. This keeps the Na+ concentration relatively low within tubule cells. The Na+ then enters the peritubular and vasa recta capillaries through intercellular clefts. The energy consumption of these pumps is substantial; they use approximately 80% of all energy invested in active transport within nephrons. If we consider that Na+ intake can vary from as little as 0.05 gram per day (g/day) if an individual is on a low-sodium diet to perhaps as high as 25 g/day, regulation of Na+ output is critical if blood Na+ levels are to be maintained within a normal homeostatic range. The amount of Na+ that is excreted in urine ranges from 0% to 2% of the total filtered Na+. Hormonal regulation of Na+ excreted in the urine occurs through aldosterone and atrial natriuretic peptide hormones in response to their binding to receptors of principal cells in the collecting tubule and collecting duct ( figure 24.19c). Aldosterone (al-dos′tĕr-ōn) is a steroid hormone (see section 17.5a) produced by the adrenal cortex. It enters principal cells to bind with intracellular receptors to form a hormone-receptor complex that stimulates protein synthesis of Na+ channels and Na+/K+ pumps. These additional transport proteins become embedded in the plasma membranes of principal cells and Na+ reabsorption increases. Water follows the Na+ by osmosis, resulting in reabsorption of an isotonic fluid. Note that K+ is secreted into the tubular fluid during this process. Atrial natriuretic peptide (ANP) inhibits both the reabsorption of Na+ in the proximal convoluted tubule and collecting tubules and the release of aldosterone (not shown in figure 24.19). Consequently, more Na+ and water (because water follows Na+ by osmosis) are excreted in urine. Recall that ANP increases GFR, a process that also increases urine output (see section 24.5e). Thus, the release of ANP provides a means of increasing urine output to decrease blood volume and blood pressure to within normal limits. Note that the details for both aldosterone and ANP are included in table R.7. Hormones that influence Na+ reabsorption are included in table 24.2. Table 24.2 Hormones That Influence Na+ Reabsorption Increase Na+ Reabsorption Decrease Na+ Reabsorption Aldosterone Atrial natriuretic peptide Cortisol Progesterone Estrogen Parathyroid hormone Growth hormone Glucagon Thyroid hormone Insulin Page 971 Water (H2O) Reabsorption Water movement occurs by osmosis. It is reabsorbed by paracellular transport between cells, and by transcellular transport through specific water transport proteins called aquaporins (see section 4.3b). Of the approximately 180 L of water filtered daily, all except an average of approximately 1.5 L is reabsorbed. The exact amount of water reabsorbed depends upon both fluid intake and fluid excreted through other routes (e.g., sweating). The tubule varies in its permeability to water along its length, and the concentration of the tubular fluid changes as it moves through the different sections of the tubule. Approximately 65% of the water in the tubular fluid is reabsorbed in the proximal convoluted tubule ( figure 24.20a). The aquaporins here are permanent components of the luminal membrane and are relatively constant in number. The movement of water out of the proximal convoluted tubule follows Na+ by osmosis, and it is referred to as obligatory water reabsorption. This water movement is dependent upon the movement of Na+, so the water is “obliged” to follow Na+. The movement of Na+ and water is equivalent, and an isotonic tubular fluid is maintained as it moves through the proximal convoluted tubule. Figure 24.20 Water Reabsorption. (a) Obligatory water reabsorption occurs in the proximal convoluted tubule (PCT): about 65%. In the nephron loop, approximately 10% of the water is reabsorbed. The amount excreted in the urine is regulated in the collecting tubules (CTs) and collecting ducts (CDs) in response to binding of antidiuretic hormone (ADH). (b) ADH binds to principal cells to cause vesicles containing aquaporin proteins (produced within tubular cells) to migrate to the luminal membrane. Additional water is “pulled” out of tubules by osmosis through the greater number of aquaporins. In the nephron loop, only about 10% of the filtered water in the tubular fluid is reabsorbed. Water moves from the descending limb of the nephron loop into the vasa recta. Both the ascending limb of the nephron loop and the distal convoluted tubule are impermeable to water, and no water reabsorption occurs there. As tubular fluid moves through the collecting tubules and collecting ducts, water reabsorption is controlled primarily by aldosterone and antidiuretic hormone. Recall that aldosterone increases the number of Na+/K+ pumps and Na+ channels in principal cells, thus increasing both Na+ and water reabsorption. Consequently, the concentration of tubular fluid is maintained ( figure 24.19). In contrast, antidiuretic (an′tē-dī′yū′ret′ik; anti = against, ouresis = urination) hormone (ADH) is released from the posterior pituitary gland when we are dehydrated. ADH binds to receptors of principal cells of the collecting tubules and collecting ducts to increase the migration of vesicles containing aquaporins to the luminal membrane. This action provides additional channels for water reabsorption ( figure 24.20b). Page 972 The osmotic force caused by the concentration gradient within the interstitial fluid (described in section 24.6f) pulls water from the tubule. Thus, water reabsorption regulated by ADH near the end of the tubule is independent of Na+ reabsorption, and as a result, solute concentration of the tubular fluid increases. Tubular reabsorption of water in response to ADH is referred to as facultative water reabsorption. ADH and Urine Production How concentrated the urine will become is dependent upon the relative amounts of ADH bound to the principal cells. Greater amounts of ADH result in larger amounts of water reabsorption by principal cells and less water remaining in the tubular fluid; thus, a smaller volume of a more concentrated urine is produced. Conditions of extreme dehydration result in subsequent high concentrations of ADH—thus, urine volume can be as little as about 0.5 L/day. Urine can be as concentrated as 1200 milliosmoles (mOsm), which is the same concentration as the interstitial fluid outside the tubules (see section 24.6f). Under these conditions, the urine generally appears noticeably darker yellow in color. In comparison, lower amounts of ADH result in smaller amounts of water reabsorption by principal cells and more water remaining in the tubular fluid—which produces a larger volume of a less concentrated urine. Conditions of overhydration (e.g., drinking a liter of soda) result in lower concentrations of ADH—thus, urine output can be several liters per day, and (with the aid of pumps that move salt out of the tubular fluid) can be as dilute as 50 mOsm. Under these conditions, the urine will appear a light yellow to very pale yellow color. INTEGRATE CONCEPT CONNECTION Blood pressure is dependent upon three major variables: cardiac output (see section 19.9), resistance (see section 20.5b), and blood volume (see section 18.1b). These variables and their influence on blood pressure are integrated in section 20.6. This section discusses both the neural regulation of blood pressure, which is controlled by the cardiovascular center (see section 20.6a), and hormonal regulation of blood pressure, which is regulated through the renin-angiotensin system, as well as aldosterone, antidiuretic hormone, and atrial natriuretic peptide hormones (see sections 20.6b and 25.4, and table R.7). The kidneys influence blood pressure (a) through the release of the enzyme renin, which is required for the formation of angiotensin II (see section 20.6b), and (b) by altering urine output. An increase in urine output decreases blood volume (and thus blood pressure), whereas a decrease in urine output helps to maintain blood volume (and thus blood pressure). The three major variables that influence blood pressure are integrated in figure 20.16. Reabsorption and Secretion of Potassium Potassium is unlike other substances previously covered because it is both reabsorbed from the tubular fluid and secreted into the tubular fluid ( figure 24.21). The result may be a net reabsorption of K+, with little being lost in urine, or a net secretion, with larger losses in the urine. Figure 24.21 Potassium Movement. Potassium is both reabsorbed and secreted along different segments of the nephron tubule, collecting tubule, and collecting duct. The amount of K+ lost in the urine is dependent upon the activity of principal cells in the collecting tubules and collecting ducts in response to aldosterone. In the proximal convoluted tubule, 60% to 80% of the K+ in the tubular fluid is reabsorbed by paracellular transport. This reabsorption of K+ is dependent upon the movement of Na+, as follows: 1. Sodium is reabsorbed across the luminal membrane. 2. Water follows the Na+. 3. The concentration of the remaining solutes in the tubular fluid increases as water follows the movement of Na+. 4. Consequently, the solute concentration of tubular fluid is greater than in the interstitial fluid, creating a gradient between the tubular fluid and interstitial fluid. 5. Potassium moves down its concentration gradient from the tubular fluid into the blood by the paracellular route. 6. These conditions also allow the passive reabsorption of other solutes, including other cations (Mg2+, Ca2+), phosphate ion (PO43−), fatty acids, and urea. Page 973 Approximately 10% to 20% of the K+ in tubular fluid is reabsorbed in the thick segment of the nephron loop ascending limb by both transcellular and paracellular transport. Either a net reabsorption or net secretion of K+ may occur in the collecting tubules and collecting ducts. The two specialized cells within these segments, intercalated cells and principal cells, have opposite effects on K+ movement. Type A intercalated cells reabsorb K+ continuously, whereas principal cells secrete K+ at varying rates based on aldosterone levels. We have seen that aldosterone stimulates principal cells to secrete K+, whereas Na+ and water are reabsorbed. A significant stimulant to cause release of aldosterone from the adrenal cortex is an elevated K+ blood level. Thus, this mechanism involves negative feedback to keep blood K+ levels close to optimum values. Calcium and Phosphate Balance Calcium and phosphate are two substances generally considered together because 99% of the body’s calcium is stored in bone, and the majority is stored as calcium phosphate. Approximately 60% of the Ca2+ in blood becomes part of the filtrate and then the tubular fluid. The remainder of the Ca2+ is bound to protein in the blood and is prevented from being filtered. In comparison, 90% to 95% of the PO43− is filtered as blood passes through glomeruli. The amount of Ca2+ and PO43− excreted in the urine is regulated by parathyroid hormone (PTH), and thus this hormone influences blood levels of both Ca2+ and PO43−. Recall from section 7.6b that PTH is released from the parathyroid gland in response to decreased blood Ca2+. One target of PTH is the kidney tubules ( figure 24.22). PTH inhibits PO43− reabsorption in the proximal convoluted tubule, and it stimulates Ca2+ reabsorption in the distal convoluted tubule. As additional PO43− is eliminated via the urine, less PO43− is available to form calcium phosphate, the major calcium salt deposited in bone. Thus, Ca2+ redeposited in the bone decreases, and blood Ca2+ increases. Figure 24.22 PTH Regulation of Calcium Ion and Phosphate Ion Reabsorption. Calcium (Ca2+) and phosphate ions ( ) are both reabsorbed in various segments of the tubule. The amounts reabsorbed are regulated by parathyroid hormone (PTH), which both inhibits the reabsorption of PO43− in the proximal convoluted tubule (PCT) and stimulates Ca2+ reabsorption in the distal convoluted tubule (DCT). Bicarbonate Ions, Hydrogen Ions, and pH The movement of bicarbonate ions (HCO3−, a weak base) and hydrogen ions (H+, an acid) plays a significant role in regulating pH of both urine and the blood. Bicarbonate ions move freely across the filtration membrane, whereas only small amounts of H+ are filtered, with most remaining in the blood. (H+ is not generally filtered because it is attached to chemical structures in the blood, such as proteins.) The filtered HCO3− must be “reclaimed” to ensure blood pH does not become too acidic ( figure 24.23a). (Note that filtered HCO3− is not specifically reabsorbed, but instead is “replaced” by a process that is described in figure 24.23b.) Approximately 80% to 90% of HCO3− is reclaimed from the tubular fluid, primarily in the proximal convoluted tubule. The remaining 10% to 20% is taken up from the thick segment of the ascending limb of the nephron loop. Thus, as the tubular fluid enters the distal convoluted tubule, usually 0% of the HCO3− originally in the filtrate remains in the tubular fluid. Page 974 Figure 24.23 Bicarbonate Ion and Hydrogen Ion Movement. (a) Bicarbonate movement occurs along certain portions of the nephron. (b) In the proximal convoluted tubule (PCT), approximately 80–90% of the filtered is replaced by an indirect mechanism. The remainder of filtered is replaced in the nephron loop (thick segment of ascending limb). (c) When [H+] is elevated, type A intercalated cells in the collecting tubules (CT) and collecting ducts (CD) synthesize new molecules of H+ and. H+ is secreted into tubular fluid and is absorbed into the blood. (Note: This is not reabsorption because the bicarbonate is being formed by type A intercalated cells, not simply moved from the filtrate into the blood.) Type B intercalated cells (not shown) are essentially “flipped” type A intercalated cells. Type B intercalated cells function when [H+] levels are decreased by secreting into the tubular fluid and absorbing H+ into the blood. The pH of urine, and consequently the pH of blood, is regulated by intercalated cells in the collecting tubules and collecting ducts ( figure 24.23c). Exactly how this regulation occurs depends upon the blood concentration of H+, which is expressed as [H+]. Increased [H+] typically occurs, for example, in individuals consuming a more acidic diet, which is a diet that includes animal protein and wheat (a typical Western diet). As a result, type A intercalated cells are actively engaged in secreting H+ (which is excreted in the urine) and synthesizing newly formed HCO3− (which is reabsorbed into the blood). The result is a decrease in urine pH (i.e., urine is more acidic) and an increase in blood pH (i.e., blood is more alkaline). The details of this process are described in figure 24.23c. Page 975 Decreased blood [H+] is more typical of individuals consuming a more alkaline diet, such as is a diet high in fruits and vegetables and little or no animal protein. In this case, type B intercalated cells are active (not shown in the figure). The action of type B cells is the reverse of that of type A cells. Think of type B intercalated cells as “flipped” type A cells: Type B cells reabsorb H+ (which lowers blood pH) and secrete (which increases urine pH). The actions of the type A and type B intercalated cells in regulating blood pH are a significant component of how the body maintains pH balance (see section 25.5b). WHAT DID YOU LEARN? 28 How does Na+ reabsorption occur? Which two hormones are involved? 29 What is the effect of parathyroid hormone on the reabsorption of both PO43− and Ca2+? 30 How is the movement of H+ and intercalated cells? regulated by type A and type B 24.6e Substances Eliminated as Waste Products LEARNING OBJECTIVES 39. Name the three nitrogenous waste products, and describe the fate of each. 40. List examples of other substances typically eliminated by kidneys. The urinary system prevents the accumulation of metabolic waste, various hormones and their metabolites, and foreign substances (e.g., drugs, chemicals) by eliminating them in the urine. Materials to be excreted in the urine are filtered at the glomerulus, secreted along the tubule pathway to ensure their elimination, or both filtered and secreted. Elimination of Nitrogenous Waste Nitrogenous waste is metabolic waste that contains nitrogen. The body’s main nitrogenous waste products are (a) urea, a small, water-soluble molecule produced from protein breakdown in the liver; (b) uric acid, produced from nucleic acid breakdown primarily in the liver; and (c) creatinine (krē-at′i-nēn, -nin), produced from metabolism of creatine in muscle tissue. Urea and uric acid are both reabsorbed and secreted, whereas creatinine is only secreted. Here we describe the processing of urea. Urea levels in the blood range from 3 to 9 milliequivalents per liter (mEq/L) and depend upon protein intake. Urea is freely filtered and is both reabsorbed and secreted in different areas of the tubules. Approximately half is reabsorbed at the proximal convoluted tubule by paracellular movement but is then secreted back into the tubule at the nephron loop by urea uniporters. Thus, 100% of the filtered urea is present in the tubular fluid as it enters the distal convoluted tubules. However, about 50% of the urea is then reabsorbed at the collecting tubules, leaving about 50% of the filtered urea to be excreted in the urine. In addition to being eliminated as a waste product, urea serves a functional role in the kidneys by helping to establish the concentration gradient in the interstitial fluid of the kidney (described in section 24.6f). Elimination of Drugs and Bioactive Substances The kidneys eliminate potentially harmful substances through both filtration and secretion in addition to eliminating nitrogenous wastes. Most secretion of these and other substances (except K+) occurs in the proximal convoluted tubule and includes the following: Certain drugs. Antibiotics (including penicillin and sulfonamides), aspirin (salicylate), morphine, chemotherapy drugs, saccharin, and chemicals in marijuana are just a few examples of the drugs that are eliminated in the urine. Other metabolic wastes. Urobilin (a bilirubin breakdown product; see section 18.3b) and hormone metabolites (e.g., intermediates of hormone breakdown) are examples of metabolic wastes that are eliminated in urine. Some hormones. Human chorionic gonadotropin (hCG) is a hormone produced in abundance during the early months of a woman’s pregnancy. It is an example of a hormone that is eliminated in the urine (see sections 29.2c and 29.5b). The presence of hCG in the urine can be used to verify a pregnancy with a pregnancy test completed at home or in the doctor’s office. Other examples of excreted hormones include epinephrine and prostaglandins. WHAT DID YOU LEARN? 31 What are some examples of drugs and bioactive substances eliminated in the urine? 24.6f Establishing the Concentration Gradient LEARNING OBJECTIVES 41. Explain what is meant by the countercurrent multiplier that occurs within the nephron loop. 42. Describe the countercurrent exchange system that maintains the concentration gradient. 43. Explain the contribution of urea cycling to the concentration gradient. An additional function that normally occurs within the kidneys is the establishment and maintenance of a concentration gradient in the interstitial fluid surrounding renal tubules ( figure 24.24). This concentration gradient is due to relatively high concentration of various solutes in the interstitial fluid, including Na+ and chloride ion (Cl−), which progressively increase in concentration from the cortex into the medulla. The concentration gradient is established and maintained by nephron loops of juxtamedullary nephrons (see section 24.3a). Figure 24.24 Interstitial Fluid Concentration Gradient. A visual representation of how the concentration gradient is established (by the countercurrent multiplier) and maintained (by countercurrent exchange). These processes occur simultaneously. (a) The countercurrent multiplier is a positive feedback mechanism within the nephron loop that establishes the salt concentration gradient in the nearby interstitial fluid. (b) Countercurrent exchange involves the vasa recta, and helps maintain the salt concentration gradient established by the countercurrent multiplier. Concept Overview Physiology Interactives The function of this concentration gradient is to exert osmotic pressure to pull water from the tubular fluid within collecting tubules and ducts; the water is then reabsorbed into the blood. Water movement from tubular fluid must cross tubular cells through aquaporins (see section 4.3b). Recall from section 24.6d that the number of aquaporins is regulated by antidiuretic hormone (ADH). Thus, controlled water reabsorption in the collecting tubules and collecting ducts by ADH requires both (a) a concentration gradient established and maintained by nephron loops (which is the “pulling force”) and (b) the presence of aquaporins in tubular cells (which are the “water channels” regulated by ADH). Note that water reabsorption is altered through changes in either of these two structures (see Clinical View 24.4: “Diuretics” and Clinical View 25.6: “Diabetes Insipidus”). This section describes how the concentration gradient in the interstitial fluid is established and maintained by (a) the nephron loop via the countercurrent multiplier, (b) the vasa recta via the countercurrent exchange system, and (c) urea recycling. Page 976 The Nephron Loop A positive feedback mechanism called the countercurrent multiplier involves the nephron loop and is partially responsible for establishing the salt concentration gradient within the interstitial fluid ( figure 24.24a). Countercurrent refers to the tubular fluid’s “reversing” its relative direction as it moves first through the descending limb and then through the ascending limb of the loop. Multiplier refers to the positive feedback loop that increases the concentration of salts (e.g., Na+, Cl–) within the interstitial fluid. The juxtamedullary nephrons (see process. section 24.3a) with their long nephron loops are primarily involved in this The descending limb of the nephron loop is permeable to water. It is also impermeable to the movement of salts out of the tubule. Water is moved from the tubular fluid into the interstitial fluid as a result, whereas salts are retained within the tubular fluid. (Note that solute concentration in the tubule increases from 300 mOsm to as much as 1200 mOsm as the tubular fluid moves through the tubule deeper into the nephron loop within the medulla— by the loss of water and retention of salt.) In contrast to the descending limb, the ascending limb is impermeable to water, and it actively pumps salt out of the tubular fluid into the interstitial fluid. As a result, water is retained in the tubular fluid, and salt is moved from the tubular fluid into the interstitial fluid. (Note that the solute concentration of the tubular fluid becomes diluted from 1200 mOsm to 100 mOsm as it moves through the ascending limb.) Most importantly, however, is that the salt concentration in the interstitial fluid surrounding the tubules increases moving from the renal cortex into the renal medulla, which sets up a positive feedback loop. Consider that as the salt concentration in the interstitial fluid increases, (a) an even greater amount of water moves out of the descending limb, and (b) this increases the salt concentration in the tubular fluid that flows into the ascending limb, which (c) allows even more salts to be pumped out of the ascending limb. Consequently, the concentration of salts in the interstitial fluid is increased, or “multiplied,” through this positive feedback loop. Vasa Recta The concentration gradient established in the interstitial fluid by the nephron loop is maintained by the unique anatomic arrangement and physiologic conditions of the vasa recta ( figure 24.24b). The blood within the vasa recta moves in the opposite direction to the tubular fluid flow within the adjacent nephron loop. So, the blood flows deep into the medulla alongside the ascending limb of the nephron loop, and it then flows toward the cortex alongside the descending limb of the nephron loop. The process by which the vasa recta help maintain the concentration gradient is termed countercurrent exchange. Recall that countercurrent refers to flow in opposite directions—in this case, blood flows in opposite directions within neighboring, parallel capillaries. The term exchange refers to the passive exchange of both salts and water between the blood and the interstitial fluid around the nephron loop. The countercurrent exchange process occurs as follows: As the blood flows through the vasa recta deep into the renal medulla alongside the ascending limb, water moves by osmosis out of these capillaries into the more concentrated interstitial fluid. At the same time, salts in the interstitial fluid enter the vasa recta by diffusion down their concentration gradients. Thus, the blood in the vasa recta is losing water and gaining salts, and the concentration of total salt in the blood increases. Thus, as blood within the vasa recta moves into the deepest part of the renal medulla, the blood becomes more and more concentrated. If the vasa recta were to continue deep into the renal medulla, these salts would be transported away in the blood. However, the path of the blood flow in the vasa recta makes a 180-degree turn and is positioned alongside the descending limb of the nephron loop toward the cortex. Salts are now transported into a region in which osmotic and solute gradients reverse. Here, the salts diffuse back out of the blood into the interstitial fluid, while water moves into the vasa recta. Page 977 Thus, as blood within the vasa recta flows toward the renal cortex, it becomes less and less concentrated. In fact, the blood within the vasa recta returning to the renal cortex has approximately the same concentration (or is slightly less concentrated) as when it first left the cortex. Most importantly, the salts remain, and are responsible for the concentration gradient in the interstitial fluid. Urea Recycling An important and additional contribution to maintaining the concentration gradient in the interstitial fluid occurs with urea recycling. Recycled urea, in fact, makes up approximately one-half of the solutes of the interstitial fluid concentration gradient. Urea is removed from the tubular fluid in the collecting duct by urea uniporters, as mentioned in section 24.6e: urea diffuses back into the tubular fluid in the thin segment of the ascending limb. Because both the thick segment of the ascending limb and the DCT are not permeable to urea, urea remains within the tubular fluid until it reaches the collecting duct, where it is removed from the tubular fluid. Thus, urea is “cycled” between the collecting duct and the ascending limb of the nephron loop. Some of this urea remains in the interstitial fluid, contributing to its concentration gradient. Summary of Reabsorption and Secretion The processes by which the nephron tubules, collecting tubules, and collecting ducts transform filtrate into urine in the different components of the nephron can be summarized and integrated as follows ( figure 24.25): 1. After filtration of plasma occurs, a majority of most substances are either reabsorbed or secreted into the proximal convoluted tubules. Additional amounts of substances are reabsorbed as the tubular fluid moves through the nephron loop. 2. The nephron loop and the surrounding vasa recta are, along with the recycling of urea between the collecting duct and nephron loop, responsible for establishing the concentration gradient of the interstitial fluid. This gradient is necessary for the normal function of ADH to regulate water reabsorption in the collecting tubules and ducts. 3. The regulation of the amount of specific substances excreted in urine is controlled mainly by principal cells and by type A and type B intercalated cells within the collecting tubules and collecting ducts. The fluid leaving the collecting ducts at the renal papilla is called urine. 4. Urine is composed of water and various dissolved substances, including ions (e.g., Na+, K+, Cl−), waste products, and perhaps drugs. Urine is drained into the renal sinus of the kidney and later excreted by the urinary tract. Under normal circumstances, urine does not contain either (a) formed elements of the blood (erythrocytes, leukocytes, platelets) because they are not filtered or (b) nutrients (e.g., glucose) and protein because they are 100% reabsorbed or reclaimed in the PCT. Page 978 INTEGRATE CONCEPT OVERVIEW Figure 24.25 Tubular Reabsorption and Tubular Secretion. Various reabsorption and secretion processes occur along the (a) proximal convoluted tubule, (b) nephron loop, (c) distal convoluted tubule, collecting tubules, and collecting ducts. WHAT DID YOU LEARN? 32 How is the concentration gradient that is essential for normal function of ADH in water reabsorption established and maintained? 33 Which substances are reabsorbed in tubular processing in the different regions of the nephron? Which are secreted? What are the general processes that occur in the different regions of the tubules: (a) PCT, (b) nephron loop, and (c) DCT, CT, and CD? INTEGRATE CLINICAL VIEW 24.4 Diuretics Diuretics (dī-ū-ret΄ik) are substances that increase excretion of urine. Diuretic drugs are a common treatment for hypertension because, by increasing urine output, both blood volume and blood pressure are decreased. As a result, diuretic drugs are sometimes referred to as water pills because of this increased urine output. Diuretic drugs are grouped into different classes depending upon their mechanism of action. Loop diuretics, such as furosemide (Lasix) and bumetanide, inhibit the transport protein (Na+/K+/2 Cl– transporters) that pump the Na+ salts within the nephron loop and lower the concentration gradient of the interstitial fluid. Thus, less osmotic force is present to pull fluid out of the collecting tubule and collecting ducts, and more fluid is excreted in urine. Thiazides, such as chlorothiazide and hydrochlorothiazide, interfere with Na+ and water reabsorption in the distal convoluted tubule. Thus, less Na+ and water are reabsorbed, increasing urine output.