Fluids, Electrolytes, and Nutrition PDF
Document Details
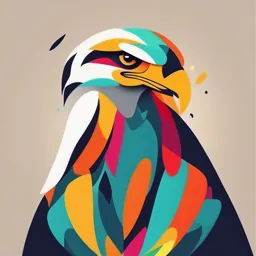
Uploaded by UnrealEinsteinium
Tags
Related
- Introduction To Fluid & Electrolyte Management (2023) PDF
- MCPC II: Study Group Session #1 PDF 2024
- Optimizing Perioperative Care: Enhanced Recovery & Chinese Medicine (Surgery 212) PDF
- 2024_Fluids & Electrolytes.pdf
- IV Therapy: Infiltration, Irritation, and Fluid Volume Overload PDF
- Fluid and Parenteral Nutrition PDF Lecture Notes
Summary
This document provides information on fluid management, including the distribution of total body fluid and intravenous fluids. It details crystalloids like normal saline and lactated Ringer solution, and colloids. The text also describes fluid resuscitation and maintenance intravenous fluids.
Full Transcript
Fluids, Electrolytes, and Nutrition I. FLUID MANAGEMENT A. Distribution of total body fluid (TBF) (Figure 1) 60% of TBF is intracellular (enclosed by the cell membrane) 75% of extracellular fluid is interstitial (this fluid “bathes the cells” and is separated from intravascular space by the semi...
Fluids, Electrolytes, and Nutrition I. FLUID MANAGEMENT A. Distribution of total body fluid (TBF) (Figure 1) 60% of TBF is intracellular (enclosed by the cell membrane) 75% of extracellular fluid is interstitial (this fluid “bathes the cells” and is separated from intravascular space by the semipermeable capillary membrane) 40% of TBF is extracellular 25% of extracellular fluid is intravascular (about 5 L of blood volume) Figure 1. Distribution of total body fluid. TBF = total body fluid. 1. 2. 3. stimated as 60% of lean body weight (LBW) in men and 50% in women; a healthy adult man (weight E 70 kg) has about 42 L of fluid Total body water is further divided into intracellular (IC) space and extracellular (EC) space. a. About 60% of TBF is IC, and 40% is EC; the IC and EC fluid compartments are separated by cell membranes, which are highly permeable to water. b. The EC compartment is also divided into the interstitial (IS) space and the intravascular space; the IS and intravascular fluid compartments are separated by the capillary membrane, which is permeable to almost all solutes except proteins. i. 75% of the EC fluid is in the IS space. ii. 25% of the EC fluid is in the intravascular space; the EC fluid in the intravascular space is known as plasma, and it measures about 3 L; if you also consider about 2 L of fluid found in red blood cells (thus, IC fluid), the total blood volume is about 5 L. The approximate distribution of TBF into the IC and EC compartments with further distribution of the EC fluid into the IS and intravascular compartments is important to remember for determining the distribution of intravenous fluid. B. Distribution of intravenous fluid (Table 1) Table 1. Distribution of Intravenous Fluid Intravenous Fluid Normal saline Lactated Ringer solution Normosol-R and Plasma-Lyte Infused Volume (mL) 1000 1000 1000 Equivalent Intravascular Volume Expansion (mL) 250 250 250 5% Dextrose 1000 100 Albumin 5% Albumin 25% Hydroxyethyl starch 6% 500 100 500 500 500 500 ACCP Updates in Therapeutics® 2023: Pharmacotherapy Preparatory Review and Recertification Course 1-360 Fluids, Electrolytes, and Nutrition 1. rystalloids are intravenous fluids that can contain water, sodium (Na+), chloride (Cl–), and other elecC trolytes. Lactated Ringer solution is a crystalloid that contains mostly Na+ and Cl–, but also lactate, potassium (K+), and calcium (Ca2+). Normosol-R and Plasma-Lyte are crystalloids that contain mostly Na+ and Cl– but also acetate, K+, and magnesium (Mg2+). D5W is also a crystalloid, but it should not be used for fluid resuscitation because of the smaller amount of fluid that remains in the intravascular compartment. a. Na and Cl– do not freely cross into cells, but they will distribute evenly in the EC space. b. For 0.9% sodium chloride or lactated Ringer solution, only 25% remains in the intravascular space, and 75% distributes in the IS space; therefore, when 1 L of 0.9% sodium chloride or lactated Ringer solution is administered, about 250 mL of fluid remains in the intravascular compartment. 2. D5W is isosmotic and, because of rapid metabolism, it has the net effect of administering “free” water. a. D5W is metabolized to water and carbon dioxide. b. Water can cross any membrane in the body; therefore, it is evenly distributed in TBF (“free” because it is free to cross any membrane). i. Many experts avoid administering D5W whenever possible in patients with neurologic injury and elevated intracranial pressure (ICP) because it can cross into cerebral cells, causing further elevation in ICP. ii. Some practitioners avoid the use of D5W because of the risk of hyperglycemia, although D5W contains only 5 g of dextrose/100 mL, which is equivalent to 17 kcal/100 mL. c. For D5W, 60% distributes to the IC space and 40% distributes to the EC space. Of the 40% distributed to the EC space, 25% remains in the intravascular space, and 75% distributes to the IS space. Therefore, when 1 L of D5W is administered intravenously, about 100 mL of fluid remains in the intravascular compartment. 3. Colloids include packed red blood cells, pooled human plasma (5% albumin, 25% albumin, and 5% plasma protein fraction), semisynthetic glucose polymers (dextran), and semisynthetic hydroxyethyl starch (hetastarch). a. Colloids are too large to cross the capillary membrane; therefore, they remain primarily in the intravascular space (although a small portion “leaks” into the IS space). b. Except for 25% albumin, administering 500 mL of colloid results in a 500-mL intravascular volume expansion. c. Because 25% albumin has an oncotic pressure about 5-fold that of normal plasma, it causes a fluid shift from the IS space into the intravascular space. For this reason, 100 mL of 25% albumin results in around 500 mL of intravascular volume expansion. This hyperoncotic solution should generally be avoided in patients requiring fluid resuscitation, because although the intravascular space expands, fluid shifts out of the IS space, potentially causing dehydration. It may be useful in patients who do not require fluid resuscitation but who could benefit from a redistribution of fluid (e.g., ascites, pleural effusions). d. Hydroxyethyl starch and dextran products have been associated with coagulopathy and kidney impairment. In addition to acute kidney injury, hydroxyethyl starch is associated with increased mortality in critically ill patients (JAMA 2013;309:678-88; N Engl J Med 2012;367:124-34). C. Fluid Resuscitation 1. Intravascular fluid depletion can occur because of shock (hypovolemic or septic shock), and it is associated with reduced cardiac function and organ hypoperfusion. 2. Signs or symptoms (Box 1) usually occur when about 15% (750 mL) of blood volume is lost (e.g., hemorrhage) or shifts out of the intravascular space (e.g., septic shock). ACCP Updates in Therapeutics® 2023: Pharmacotherapy Preparatory Review and Recertification Course 1-361 Fluids, Electrolytes, and Nutrition Box 1. Signs and Symptoms of Intravascular Volume Depletion Tachycardia (HR > 100 beats/minute) Hypotension (SBP < 80 mm Hg) Orthostatic changes in HR or BP Increased BUN/SCr ratio > 20:1 Dry mucous membranes Decreased skin turgor Reduced urine output Dizziness Improvement in HR and BP after a 500- to 1000-mL fluid bolus BP = blood pressure; BUN = blood urea nitrogen; HR = heart rate; SBP = systolic blood pressure; SCr = serum creatinine. 3. 4. 5. Fluid resuscitation is indicated for patients with signs or symptoms of intravascular volume depletion. The goal of fluid resuscitation is to restore intravascular volume and to prevent organ hypoperfusion. Because intravascular volume depletion can cause organ dysfunction and death, prompt resuscitation is necessary. a. Intravenous fluids are infused rapidly, preferably through a large-bore catheter. b. Intravenous fluids are administered as a 500- to 1000-mL bolus, (~30 mL/kg in septic patients) after which the patient is reevaluated; this process is continued as long as signs and symptoms of intravascular volume depletion are improving (Box 1). Table 2. Content of Common Crystalloid Solutions Sodium chloride 0.9% (NS) Lactated Ringer (LR) Normosol-R Contents (mEq/L) Osmolarity (mOsmol/L) Na 154 Cl 154 Na 130 Cl 109 K4 Ca 3 Lactate 28 Na 140 Cl 98 K5 Mg 3 Acetate 27/Gluconate 23 308 273 295 Ca = calcium; Cl = chloride; K = potassium; Mg = magnesium; Na = sodium. 6. Crystalloids are recommended for fluid resuscitation in hypovolemia (Table 2). a. Lactated Ringer solution is historically preferred in surgery and trauma patients, but no evidence suggests superiority over normal saline for fluid resuscitation in these settings. b. The lactate in lactated Ringer solution is metabolized to bicarbonate, and it can theoretically be useful for metabolic acidosis; however, lactate metabolism is impaired during shock. Thus, lactated Ringer solution may be an ineffective source of bicarbonate. ACCP Updates in Therapeutics® 2023: Pharmacotherapy Preparatory Review and Recertification Course 1-362 Fluids, Electrolytes, and Nutrition c. 7. Lactated Ringer solution has been considered to provide a more physiologic amount of Cl (109 mmol/L) than 0.9% sodium chloride (154 mmol/L). In the SALT-ED trial, compared with 0.9% sodium chloride, a balanced fluid regimen (e.g., lactated Ringer solution, Plasma-Lyte 148) resulted in a lower rate of a composite outcome of any cause of death, new renal replacement therapy, and persistent renal dysfunction in intensive care unit (ICU) patients. There was no difference found in hospital-free days in non-ICU patients (N Engl J Med 2018; 378:819-39). However, more recent trials have found no differences between balanced crystalloids and 0.9% sodium chloride. The BaSICS trial, which compared a balanced solution to 0.9% sodium chloride, found no difference in 90-day mortality in critically ill patients (JAMA 2021;326:818-829.) Another trial showed no difference in death or acute kidney injury in ICU patients receiving balanced crystalloids versus 0.9% sodium chloride (N Engl J Med 2022;386:815-826). There is no difference between crystalloids and colloids in the time to achieve fluid resuscitation or in patient outcomes. Colloids have not been shown to be superior to crystalloids, and they are associated with higher cost and some adverse effects. The following are examples of other, although controversial, uses of colloids: a. Colloids can be considered after fluid resuscitation with crystalloid (usually 4–6 L) has failed to achieve hemodynamic goals or after clinically significant edema limits the further administration of crystalloid. b. Albumin can be considered in patients with a low albumin concentration who have required a large volume of resuscitation fluids or in cirrhotic patients receiving a large volume paracentesis. c. Albumin (theoretically, 25% is preferred) can be considered in conjunction with diuretics for patients with clinically significant edema (e.g., pulmonary edema causing respiratory failure) and a low albumin concentration, when appropriately dosed diuretics are ineffective. D. Maintenance intravenous fluids 1. Maintenance intravenous fluids are indicated in patients who are unable to tolerate oral fluids. 2. The goal of maintenance intravenous fluids is to prevent dehydration and to maintain a normal fluid and electrolyte balance. 3. Maintenance intravenous fluids are typically administered as a continuous infusion through a peripheral or central intravenous catheter. 4. Common methods of estimating the daily volume in children and adults: a. Administer 100 mL/kg for first 10 kg, followed by 50 mL/kg for the next 10–20 kg (i.e., 1500 mL for the first 20 kg) plus 20 mL/kg for every kilogram greater than 20 kg or b. Administer 20–40 mL/kg/day (for adults only). c. Adjust fluids according to the individual patient’s input, output, and estimated insensible loss. 5. A typical maintenance intravenous fluid is D5W with 0.45% sodium chloride plus 20–40 mEq of potassium chloride per liter. The potassium chloride content can be adjusted for the individual patient. 6. If 150 mEq of sodium bicarbonate is added to 850 mL of 0.9% sodium chloride, the resultant solution is equivalent to about 1.6% sodium chloride. When an infusion of 150 mEq of sodium bicarbonate per liter is indicated, it is recommended to add sodium bicarbonate to D5W or sterile water for injection instead of 0.9% sodium chloride. ACCP Updates in Therapeutics® 2023: Pharmacotherapy Preparatory Review and Recertification Course 1-363 Fluids, Electrolytes, and Nutrition Patient Case Questions 1 and 2 pertain to the following case. A 65-year-old man (weight 80 kg) with a 3-day history of a body temperature of 102°F (38.9°C), lethargy, and productive cough is hospitalized for community-acquired pneumonia. His medical history includes uncontrolled hypertension and coronary artery disease. His vital signs include heart rate 104 beats/minute, blood pressure 112/68 mm Hg, and body temperature 101.4°F (38.6°C). His urine output is 10 mL/hour, K+ is 4 mEq/L, BUN is 46 mg/dL, SCr is 1.7 mg/dL, and WBC is 10.4 × 103 cells/mm3. Other laboratory values are normal. 1. Which is most appropriate at this time? A. Furosemide 40 mg intravenously. B. Albumin 25% 100 mL intravenously over 60 minutes. C. Lactated Ringer solution 1000 mL intravenously over 60 minutes. D. D5W/0.45% sodium chloride plus potassium chloride 20 mEq/L to infuse at 110 mL/hour. 2. fter 2 days of appropriate antibiotic treatment, the patient has a WBC of 9 × 103 cells/mm3, and he is afebrile. A His blood pressure is 135/85 mm Hg, and his urine output is 45 mL/hour. His albumin is 3.2 g/dL, BUN is 14 mg/dL, K+ is 3.9 mEq/L, and SCr is 1.4 mg/dL. All other laboratory values are normal. His appetite is still poor, and he is not taking adequate fluids. He has peripheral intravenous access. Which option is most appropriate to initiate? A. Peripheral PN to infuse at 110 mL/hour. B. Albumin 5% 500 mL intravenously over 60 minutes. C. D5W/0.45% sodium chloride plus potassium chloride 20 mEq/L to infuse at 110 mL/hour. D. Lactated Ringer solution to infuse at 75 mL/hour. II. OSMOLALITY A. Plasma osmolality is normally 275–290 mOsm/kg. 1. Terminology a. Osmolality is a measure of the osmoles of solute per kilogram of solvent (Osm/kg), whereas osmolarity is a measure of osmoles of solute per liter of solution (Osm/L). b. Plasma osmolarity (mOsm/L) can be calculated as osmolality × 0.995, showing that there is no clinically significant difference between them (i.e., plasma osmolarity is about 1% lower than plasma osmolality). 2. Plasma osmolality is maintained within a normal range by thirst and secretion of arginine vasopressin (i.e., antidiuretic hormone [ADH]) from the posterior pituitary. 3. Sodium salts are the primary determinant of plasma osmolality, and they regulate fluid shifts between the IC and EC fluid compartments. 4. Plasma osmolality (mOsm/kg) can be estimated: (2 × Na+ mEq/L) + (glucose mg/dL/18) + [(BUN mg/ dL) ÷ 2.8]. 5. Increases in plasma osmolality cause an osmotic shift of fluid into the plasma, resulting in cellular dehydration and shrinkage. 6. Decreases in plasma osmolality cause an osmotic shift of fluid into cells, resulting in cellular overhydration and swelling. ACCP Updates in Therapeutics® 2023: Pharmacotherapy Preparatory Review and Recertification Course 1-364 Fluids, Electrolytes, and Nutrition B. Intravenous fluids can be classified by their osmolarity relative to plasma. 1. Isotonic fluid does not result in a fluid shift between fluid compartments because the osmolarity is similar to plasma. 2. Hypertonic fluid, such as NaCl 3%, can cause fluid to shift from the IC to the EC compartment, with subsequent cellular dehydration and shrinkage. 3. Hypotonic fluid, such as NaCl 0.225%, with an osmolarity less than 150 mOsm/L can cause fluid to shift from the EC to the IC compartment, with subsequent cellular overhydration and swelling. a. Red blood cell swelling can cause cell rupture (i.e., hemolysis). b. Brain cells can swell, causing cerebral edema and herniation; this is most likely to occur with rapid sodium lowering. C. Definitions 1. Equivalent weight = Molecular weight (MW) divided by valence. a. A milliequivalent (mEq) = 1/1000 of an equivalent. b. Examples of equivalent weight are shown in Table 3. c. 1 mol = equivalent weight Table 3. Electrolyte MW, Valence, and Equivalent Weight Electrolyte Sodium Potassium Chloride Magnesium MW 23 39 35.5 24 Valence 1 1 1 2 Equivalent Weight (g) 23 39 35.5 12 MW = molecular weight. 2. Osmoles = number of particles in a solution (assuming complete dissociation). a. A milliosmole = 1/1000 of an osmole. b. Examples of osmoles are shown in Table 4. Table 4. Osmoles Salt NaCl KCl CaCl2 Osmoles 2 2 3 CaCl2 = calcium chloride; KCl = potassium chloride; NaCl = sodium chloride. 3. Converting MW to milliequivalents (Box 2) Box 2. Converting MW to Milliequivalents Convert 23.4% NaCl (concentrated NaCl) to mEq/mL MW of NaCl = 23 + 35.5 = 58.5 (add MW of Na + Cl) 23.4 g 1 equiv 1000 mEq ––––––– × –––––––– × –––––––––– = 4 mEq/mL 100 mL 58.5 g 1 equiv MW = molecular weight; NaCl = sodium chloride. ACCP Updates in Therapeutics® 2023: Pharmacotherapy Preparatory Review and Recertification Course 1-365 Fluids, Electrolytes, and Nutrition D. Calculating the osmolarity of intravenous fluids in milliosmoles per liter 1. The osmotic coefficient can be used to calculate the osmolarity of intravenous fluids because salt forms do not completely dissociate in solution. However, commercially available products often have different reported osmolarities than if if the osmolarity is calculated using the osmotic coefficient. a. With sodium chloride, for example, there is some ionic attraction between Na+ and Cl, and they do not completely dissociate; rather, they are about 93% dissociated in solution (thus, the osmotic coefficient is 0.93). b. In clinical practice and in commercially available products, most do not consider the osmotic coefficient when calculating the osmolarity of sodium chloride or other electrolytes. In reality, the osmotic coefficient is probably not clinically relevant (but it is used in the following examples for completeness). 2. Normal saline (0.9% sodium chloride) (Table 5) Table 5. Calculation for Normal Saline Using the Osmotic Coefficient Molecular Weight Osmoles Osmotic Coefficient 58.5 g/mol 2 0.93 0.9 g 1 mol 2 Osm 1000 mOsm 1000 mL ––––––– × –––––– × –––––– × –––––––––– × –––––––– × 0.93 = 287 mOsm/L 100 mL 58.5 g 1 mol 1 Osm 1 L 3. D5W (MW 198 g/mol) (Box 3) Box 3. Calculation for D5W 5 g × 1 mol 1000 mOsm 1000 mL = 252.5 mOsm/L ––––––– × –––––– × –––––––––––– × –––––––– 100 mL 198 g 1 mol 1 L 4. 5. Osmolarity of D5W/normal saline using the osmotic coefficient = 252.5 mOsm/L + 287 mOsm/L = 539.5 mOsm/L. Osmolarity of normal saline + potassium chloride 20 mEq/L (Box 4) Box 4. Calculation for NS Plus 20 mEq KCl Using the Osmotic Coefficient Step 1: Convert mEq to weight (g) 1 equiv 74.5 g 20 mEq × –––––––––– × –––––––– = 1.49 g of KCl 1000 mEq 1 equiv Step 2: Calculate mOsm/L 1.49 g 1 mol 2 Osm 1000 mOsm ––––––– × –––––––– × –––––––– × –––––––––––– = 40 mOsm/L L 74.5 g 1 mol 1 Osm Step 3: Add osmolarity of NS + KCl = 287 mOsm/L + 40 mOsm/L = 327 mOsm/L NS = normal saline; KCl = potassium chloride. ACCP Updates in Therapeutics® 2023: Pharmacotherapy Preparatory Review and Recertification Course 1-366 Fluids, Electrolytes, and Nutrition III. HYPERTONIC SALINE A. C oncentration: Typically use 3% (954 mOsm/L), 7.5% (2393 mOsm/L), or 23.4% (7462 mOsm/L). Sodium chloride 3% and 23.4% are available commercially, whereas other concentrations must be extemporaneously prepared. B. Common uses of hypertonic saline 1. Hypertonic saline is used in traumatic brain injury to reduce an elevated ICP and thereby increase cerebral perfusion pressure. It is typically used if sustained ICP is greater than 20 mm Hg as measured with an ICP monitor. It is also used for cerebral edema or herniation. 2. Hypertonic saline is used for symptomatic hyponatremia (symptoms described later in the Hyponatremia section). a. Symptoms generally do not occur unless serum sodium is 120 mEq/L or less, and they increase in severity as Na+ decreases. b. Symptoms of severe hyponatremia may include coma and seizures. c. In an effort to prevent severe symptoms from occurring, some practitioners treat asymptomatic or moderately symptomatic (e.g., lethargy, confusion) hyponatremia before serum sodium concentrations reach 120 mEq/L or less because of the increased risk of severe symptoms below this concentration. C. Inappropriate use of hypertonic saline 1. Chronic asymptomatic hyponatremia a. Asymptomatic syndrome of inappropriate secretion of antidiuretic hormone (SIADH) is usually treated with fluid restriction of less than 1000 mL of fluid per day. b. Hyponatremia is generally a water problem (i.e., an excess of free water) rather than a deficiency of Na; thus, hypertonic saline makes little sense in the absence of symptoms (see Hyponatremia section). 2. Hyponatremia associated with severe hyperglycemia (pseudohyponatremia; i.e., diabetic ketoacidosis) a. Typically, serum sodium decreases in a nonlinear fashion in response to hyperglycemia (i.e., Na+ decreases by about 1.6 mEq/L for every 100-mg/dL elevation in glucose of 100–400 mg/dL; however, another version of the formula shows that Na+ decreases by about 2.4 mEq/L for every 100-mg/dL elevation in glucose above 100 mg/dL). Corrected Na+ = serum Na+ + [1.6 (glucose – 100)/100] b. As hyperglycemia is corrected with insulin, the serum sodium will normalize. 3. Hyponatremia associated with hypervolemia (i.e., heart failure leads to tissue hypoperfusion, which triggers ADH secretion, causing reabsorption of water from the kidneys and leading to hyponatremia) a. In general, this situation is treated with fluid restriction or diuresis. b. Symptomatic hyponatremia is uncommon in patients with heart failure. c. Hypertonic saline could be considered in symptomatic patients; however, they may also need diuresis to prevent worsening volume overload. ACCP Updates in Therapeutics® 2023: Pharmacotherapy Preparatory Review and Recertification Course 1-367 Fluids, Electrolytes, and Nutrition D. Preparation of hypertonic saline (Commercially available products should be used whenever possible to minimize the risk of error) (Figure 2) Steps Choose base solutions Example For this example, use concentrated NaCl available as 23.4% vials and sterile water to make 1000 mL of 7.5% HS Set up alligation 23.4% 7.5% 0 Add and subtract 7.5 parts (from 23.4% NaCl) 23.4% 7.5% 15.9 parts (from sterile water) 23.4 parts total 7.5 parts/23.4 parts = x/1000 mL; x = 320.5 mL of 23.4% NaCl 15.9 parts/23.4 parts = x/1000 mL; x = 679.5 mL of sterile water 0 Divide Figure 2. Calculations to prepare hypertonic saline. HS = hypertonic saline; NaCl = sodium chloride. E. Hypertonic saline dose 1. Dose options for traumatic brain injury and other neurological injuries a. 3% hypertonic saline 250 mL or 2–4 mL/kg intravenously over 1–15 minutes administered for elevated ICP or cerebral edema b. 23.4% hypertonic saline 30 mL over 20–30 minutes administered for elevated ICP i. Standing orders such as 30 mL every 4–6 hours are sometimes used or may be used as needed for a sustained ICP greater than 20 mm Hg. ii. If hypertonic saline is needed for prolonged reduction in ICP, a 3% hypertonic saline continuous infusion may be recommended. 2. Dose options for patients with symptomatic hyponatremia a. Treatment of patients with symptomatic hyponatremia involves a small but quick increase in serum sodium by 0.75–1 mEq/L/hour to a concentration of 120 mEq/L, though not more than 10–12 mEq in 24 hours. Next, the infusion can be reduced so that Na+ increases by 0.5 mEq/L/hour. For severe symptoms, it is reasonable to increase serum sodium by up to 2 mEq/L/hour for a short time, as long as the maximum change of 10–12 mEq in 24 hours is not exceeded. If hypertonic saline is used for mild symptoms, a slower change in serum sodium of 0.5 mEq/L/hour would be appropriate, although some would avoid hypertonic saline altogether. Some protocols are more conservative, recommending a maximum change of 8 mEq in 24 hours. If the maximum rate is exceeded in 24 hours or the rate in sodium rise is increasing too rapidly, some practitioners recommend counteracting the quick rise with desmopressin or dextrose 5% in water (more info in hypernatremia section). b. Estimate an infusion rate of 3% hypertonic saline by multiplying ideal body weight (IBW) by desired rate of serum sodium increase per hour. (Note: IBW is used to avoid overdosing patients with obesity.) i. For example, 70 kg × 1 mEq/L/hour = 70 mL/hour to increase serum sodium by 1 mEq/L in 1 hour. The infusion can be adjusted to achieve goal changes in serum sodium. ii. Infusion rate of 3% hypertonic saline is generally 1–2 mL/kg/hour. iii. In general, 3% hypertonic saline is not recommended in asymptomatic patients; if used in an asymptomatic patient, the administration rate should generally not exceed 0.5–1 mL/kg/hour. ACCP Updates in Therapeutics® 2023: Pharmacotherapy Preparatory Review and Recertification Course 1-368 Fluids, Electrolytes, and Nutrition c. F. lternatively, some practitioners recommend a 250-mL bolus of 2%–3% hypertonic saline over A 30 minutes or 50 mL of 3% hypertonic saline administered as a bolus every 30 minutes for two doses. Administration of hypertonic saline 1. Use central intravenous access because the osmolarity is greater than 900 mOsm/L. 2. If no central line is available, consider using 2% hypertonic saline. 3. Some practitioners use 3% hypertonic saline through a peripheral intravenous access site in an emergency, because the osmolarity is close to the cutoff range for peripheral administration. Recent literature suggests that 3% sodium chloride can safely be administered through a peripheral line (J Neurosci Nurs 2017;49:191-5). If a peripheral site is used, use a large vein and monitor for phlebitis. G. Clinical goals and monitoring for administering hypertonic saline in patients with symptomatic hyponatremia 1. Goals a. Decrease symptoms (described later). b. Safe serum sodium achieved usually in the range of 120–125 mEq/L to avoid adverse neurologic outcomes. Note that the immediate goal for patients with symptomatic hyponatremia is not necessarily a normal serum sodium. c. Reached maximum safe amount of change in serum sodium i. Maximum safe amount of change is generally regarded as 10–12 mEq/L in 24 hours. ii. Some practitioners suggest a maximum change of 8 mEq/L in 24 hours. 2. Monitoring of serum sodium every 1–4 hours depending on severity of symptoms H. Complications of hypertonic saline 1. Osmotic demyelination syndrome (includes central pontine and extrapontine myelinolysis) can occur with rapid correction of hyponatremia. a. It is characterized initially by lethargy and affect changes, followed by permanent neurologic damage, including paraparesis, quadriparesis, dysarthria, dysphagia, and coma. b. It is more likely to occur with rapid correction of chronic hyponatremia than with acute hyponatremia. This partly explains why it is advisable not to administer hypertonic saline in patients with chronic asymptomatic hyponatremia. c. Prevent this complication by avoiding changes in serum sodium of more than 10–12 mEq/L in 24 hours or more than 18 mEq/L in 48 hours. 2. Hypokalemia can occur with large volumes of hypertonic saline. 3. Hyperchloremic acidosis can result from the administration of chloride salts (i.e., sodium chloride). It can be prevented by administering hypertonic saline in a 1:1 or 2:1 ratio of sodium chloride and sodium acetate or using fluid with less chloride content. 4. Hypernatremia 5. Phlebitis if administered in a peripheral vein 6. Heart failure a. Fluid overload can result from initial volume expansion. b. Over time, hypertonic saline can have a diuretic effect, leading to intravascular volume depletion. 7. Coagulopathy caused by platelet dysfunction 8. Hypotension if hypertonic saline is administered rapidly I. Other considerations when using hypertonic saline. a. Because hypokalemia can cause hyponatremia, remember to correct K+ depletion if present. As K+ is replaced, serum sodium will increase. ACCP Updates in Therapeutics® 2023: Pharmacotherapy Preparatory Review and Recertification Course 1-369