Molecular Biology I: Nucleic Acid Metabolism Lecture 04 (2024 S1) PDF
Document Details
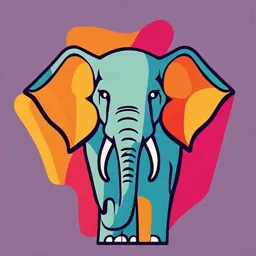
Uploaded by ZippyPelican
null
2024
Tags
Related
Summary
This document is a lecture from a Molecular Biology I course on nucleic acid metabolism. The lecture focuses on DNA replication, going over topics such as DNA topology, topoisomerases, and the differences between prokaryotic and eukaryotic DNA replication. The material covers the leading and lagging strand synthesis and the various proteins involved, including DNA polymerases, helicases, primase, and ligase.
Full Transcript
MOLECULAR BIOLOGY I: NUCLEIC ACID METABOLISM SC/BIOL 3110, 2024 S1 Lecture 04: DNA replication 1 Announcements: I am not available for Office Hours this afternoon, so I have changed this week’s Office Hours to Fri (May 17) from 10 AM to 12 noon – you can sign up via Scheduler on eClass Midterm...
MOLECULAR BIOLOGY I: NUCLEIC ACID METABOLISM SC/BIOL 3110, 2024 S1 Lecture 04: DNA replication 1 Announcements: I am not available for Office Hours this afternoon, so I have changed this week’s Office Hours to Fri (May 17) from 10 AM to 12 noon – you can sign up via Scheduler on eClass Midterm 1 will take place in-person in SLH A one week from today (May 23) from 10 to 11:30 AM. Make sure you bring a pencil for filling in bubbles on the scantron sheets, as well as eraser, calculator, and student ID. Midterm 1 will cover material from Lecture 1 to slide 48 of Lecture 4. 2 Recap of last lecture DNA topology: DNA topology can be described by the mathematical formula: Lk = Tw + Wr Where Lk = Lk = Linking number Tw = Twist Wr = Writhe The number of times one DNA strand has to pass through another to separate the strands à requires cutting of a DNA strand 3 Recap of last lecture DNA topology: DNA topology can be described by the mathematical formula: Lk = Tw + Wr Tw = the number of times one DNA strand completely wraps around the other strand (i.e. 360o) à i.e. the number of helical turns in a DNA duplex Note: while Lk has to be an integer, Tw and Wr mathematically can be fractions (e.g. 32.1. 32.2, 32.3 …) 4 Recap of last lecture DNA topology: Wr = the number of times the DNA duplex crosses over itself (supercoils) DNA wrapping in an anti-clockwise direction = left-handed Plectonemic Right-handed cross over = -ve supercoil Toroidal Left-handed wrap = -ve supercoil A flattened view of toroidal negative supercoil 5 Recap of last lecture DNA topology math: Lko = Linking number of the relaxed DNA = length of DNA divided by 10.5 For relaxed DNA, Wr = 0, so Lk = Lko = Tw DLk = Lk – Lko = Wr = # of supercoils (neg # = neg supercoils, pos # = pos supercoils) Most circular DNA isolated from bacteria or eukaryotes are negatively supercoiled Negative supercoiling stores energy to help processes such as DNA replication and transcription by making strand separation easier 6 Recap of last lecture Lk = Tw + Wr Can interconvert 7 Recap of last lecture How to create net negative supercoiled genomes in eukaryotes: Lk = Tw + Wr no change -1 +1 cannot 8 Recap of last lecture Ways to relax supercoiled DNA: 1. DNase I: introduces “nick” in DNA to relieve topological strain 2. Ethidium Bromide: = DNA intercalator 3. Topoisomerases: à physiological way to change topological properties of DNA 9 Recap of last lecture: Functions of DNA topoisomerases: 1. Remove or introduce supercoils 2. Decatenate or catenate DNA 3. Unknot or knot DNA 10 Recap of last lecture: DNA topoisomerases: Types of topoisomerases: 11 Recap of last lecture: DNA topoisomerases: Type I activity at the DNA level: Type IA relaxes –ve supercoils Type IB relaxes +ve and –ve supercoils 12 Recap of last lecture: Mechanisms of DNA topoisomerases: Topoisomerases cut, move one (or two) DNA strand(s) relative to the other, and re-ligate (re-seal) DNA à this will change linking number. 13 Recap of last lecture: Mechanisms of DNA topoisomerases: Topoisomerases cut, move one (or two) DNA strand(s) relative to the other, and re-ligate (re-seal) DNA à this will change linking number. 14 Recap of last lecture: DNA topoisomerases: Type II activity at the DNA level: 15 Recap of last lecture: Mechanisms of DNA topoisomerases: Type II topoisomerase: 16 Recap of last lecture: Mechanisms of DNA topoisomerases: Basic steps of Type II topoisomerase activity: 17 Recap of last lecture Bacterial gyrase is a specialized Type II Topoisomerases: Gyrase is a prokaryote-specific enzyme that introduces negative supercoils to the genome Turn is directional à specifically introduces negative supercoils Specific inhibitors of gyrase: Coumarins, quinolones Gyrase also relaxes positive supercoils These properties have respective functions in the initiation of DNA replication and the progression of replication forks 18 Recap of last lecture Clinical uses of topoisomerase inhibitors: Type IB topo inhibitors e.g. Camptothecin and derivatives: Type IB inhibitor: ssDNA nick à ds break after DNA replication à cell death 19 Recap of last lecture Clinical uses of topoisomerase inhibitors: Type II topoisomerase inhibitors: Two types: Catalytic inhibitors or Poisons Best for treating cancers with lower than normal amounts of topo II Catalytic inhibitors can block various steps involved in Topo II function to inhibit its activity (e.g. block DNA duplex binding or release of ADP to re-set ATPase domain). Inhibitors Poisons convert Topo II into a “poison” for the cell by increasing Topo II-induced ds DNA breaks (e.g block religation of Topo II-cleaved DNA). Poisons Best for treating cancers with higher than normal amounts of topo II 20 Recap of last lecture: 3 models of DNA replication: Note that after 1st round of replication, both dispersive and semiconservative models predicted half-blue/half-red DNA duplexes 21 Recap of last lecture: Semi conservative replication: Meselson and Stahl’s experiment directly tested the 3 models of DNA replication 22 DNA synthesis and replication: Visualizing the replication fork: Cairns (1963) used 3H thymidine to label replicating DNA from E. coli and visualized the newly synthesized DNA by autoradiography. à VERY carefully extracted radioactively labeled DNA, placed DNA on grid and exposed to film for a long time. à Proposed that E. coli DNA replicates by forming a fork, and called the replication intermediates as Theta forms (Q) 23 DNA synthesis and replication: Visualizing the replication fork: Since can see replication bubble in replicating E. coli genome… à does DNA replication proceed in unidirectional or bidirectional manner? 24 DNA synthesis and replication: Visualizing the replication fork: Cairns used short pulses of 3H thymidine to specifically visualize newly synthesized DNA at the forks. Predicted results: Actual results: 25 DNA synthesis and replication: Visualizing the replication fork: Conclusion: E. coli DNA replicates in bidirectional manner (same for all other organisms). Moreover, origin of replication lies in the middle of the replication bubble. 26 DNA synthesis and replication: E coli chromosome replication: E. coli has a circular genome, and has a single origin of replication (oriC). Need topoisomerase to decatenate the two daughter genomes 27 DNA synthesis and replication: Eukaryotic DNA replication: Eukaryotes have linear chromosomes and have multiple origins of replication. Electron micrograph showing multiple replication bubbles on eukaryotic DNA 28 DNA synthesis and replication: Eukaryotic DNA replication: Eukaryotes have linear chromosomes and have multiple origins of replication. 29 DNA synthesis and replication: Leading vs lagging strand synthesis: DNA can only synthesize in 5’ to 3’ direction à creates problems for lagging strand synthesis. Direction of replication fork movement ? 30 DNA synthesis and replication: Leading vs lagging strand synthesis: Okazaki (mid-60s) proposed 3 “formal” models for DNA replication at the replication fork: Predicted sizes of newly syn’d DNA: Long strands only Long strands + short fragments Short fragments only ! 31 DNA synthesis and replication: Leading vs lagging strand synthesis: Okazaki did pulse labeling (3H thymidine) of growing E. coli for very short durations (secs), harvested genomic DNA and separated the DNA on sucrose gradients. Looked at the sizes of the labeled fragments. Observed short pieces of DNA when pulse labeled for very short time. Longer pulse labeling resulted in longer pieces of labeled DNA. ! 32 DNA synthesis and replication: Leading vs lagging strand synthesis: Okazaki did pulse labeling (3H thymidine) of growing E. coli for very short durations (secs), harvested genomic DNA and separated the DNA on sucrose gradients. Looked at the sizes of the labeled fragments. Repeated experiment in mutant E. coli that is missing DNA ligase à no longer see longer pieces of labeled DNA at longer times of pulse labeling. Concluded that short pieces of DNA are synthesized first, which then ligate to form longer pieces of DNA. à short pieces of DNA on lagging strand = Okazaki fragments ! 33 DNA synthesis and replication: DNA replicates in a semi-discontinuous manner: Leading strand replicates in continuous manner. Lagging strand replicates in discontinuous manner. Okazaki fragments: ~ 1000-2000 nts in length in E. coli ~ 100 – 200 nts in length in eukaryotes 34 DNA synthesis and replication: List of proteins needed for DNA replication: 1. Origin binding proteins (AKA Licensing factors). 2. Helicase to unwind duplex. 3. Single Strand Binding (SSB) proteins that prevent reannealing of melted DNA strands. 4. Primase to make primers (RNA primers) to initiate DNA synthesis. 5. DNA polymerases to add nucleotides (nts) to the newly synthesizing strands. 6. “Clamp proteins” to keep DNA polymerase on DNA (help processivity). 7. “Proof-reading” machinery/activity to ensure the right nts are added. 8. Something to remove RNA primers. 9. Ligase to ligate Okazaki fragments together. 10. Topoisomerases to resolve torsional strains or decatenate daughter strands. 35 DNA synthesis and replication: 3 main steps in DNA replication: 1. Initiation 2. Elongation 3. Termination 36 DNA synthesis and replication: Initiation phase of DNA replication: Initiation of DNA synthesis occurs at specific sites = origins of replication. In E. coli: single origin of replication per genome = oriC. oriC consists of three A/T-rich regions and four 9 bp DnaA boxes DNA replication is initiated by the binding of DnaA to the DnaA boxes. This stimulates cooperative binding of 20 – 40 DnaA proteins to form large complex. DnaA binding occurs only when DNA is negatively supercoiled, and when oriC is methylated. 37 DNA synthesis and replication: Initiation phase of DNA replication in E. coli: DnaA (= Licensing factor for E. coli) is an ATP-binding protein and binds to oriC as DnaA-ATP complex. Binding of DnaA results in strand separation (melting) of the A/T-rich regions. Mechanism likely mediated by torsional strain introduced by binding of DnaA complex leading to loss of negative supercoil in the region. DNA melting is facilitated by HU proteins, but mechanism = unknown. 38 DNA synthesis and replication: Initiation phase of DNA replication in E. coli: DnaB (= helicase) and DnaC form a pre-priming complex, and they together bind DnaA complex at oriC. Two DnaB-DnaC complexes are bound per origin à one each for the two replication forks. DnaC represses helicase activity of DnaB, has a transitory role and its release marks the initiation of the replication forks moving away from the oriC. Travels along the DNA strand in the 5’ to 3’ direction, using energy from ATP 39 DNA synthesis and replication: Initiation phase of DNA replication in E. coli: DnaB (two hexamers) unwinds DNA that is opened by DnaA. DnaB acts like a rotary engine to pry apart the double helix. 40 DNA synthesis and replication: Initiation/elongation phase of DNA replication in E. coli: As DnaB hexamers move on each strand of the DNA in opposite directions, this creates two replication forks that widens the replication bubble. The increased single-stranded region enables other enzymes involved in the elongation phase of DNA replication to attach. 41 DNA synthesis and replication: Other proteins that are recruited to replication forks: Topoisomerase is recruited to resolve +ve supercoils ahead of the replication fork. Single-strand binding proteins (SSBs) bind the single-stranded DNA to prevent them from reannealing, and also to protect them from nucleases. DnaG (primase) is recruited and loaded onto the helicase to form the “primosome”. This enzyme synthesizes RNA primers (10 – 12 nts) on the leading and lagging strands to “prime” DNA synthesis by the DNA polymerase (need 3’ OH to add next nt). 42 DNA synthesis and replication: DNA polymerases: DNA polymerases catalyze the addition of nucleotides to the growing DNA chains. In E. coli, there are at least 5 DNA polymerases (some are multi-protein complexes): DNA pol I, II, III, IV and V. Numbering is based on order of discovery. DNA pol I was discovered by Arthur Kornberg à led to his Nobel prize in 1959. Kornberg and colleagues initially set out to synthesize DNA in vitro and in the process, not only identified the proteins and factors needed for DNA synthesis, but also elucidated the biochemistry of DNA replication. First observation: E. coli extract can catalyze incorporation of 14C (radioactive) thymidine into DNase sensitive product (i.e. DNA) à = in vitro assay. Second, developed way to make a-32P dTTP, which greatly improved sensitivity of assay. 43 DNA synthesis and replication: Discovery of DNA polymerase I in E. coli: Through fractionation and chromatography, were able to determine components needed for in vitro DNA synthesis: JBC, 2003, 278: 34744 1. ATP (for energy) 2. DNA à needed as template à fits in with Watson and Crick’s model of DNA replication 3. dNTPs 4. Mg2+ 5. Enzyme (which they called polymerase) 6. Primer 44 DNA synthesis and replication: Discovery of DNA polymerase I in E. coli: Kornberg and colleagues purified DNA pol I, which is capable of synthesizing DNA in vitro. DNA pol I is a single polypeptide chain that has 3 components/activities: 45 DNA synthesis and replication: General features of DNA polymerases: All polymerases have similar structure and function: It is the a-phosphate of the incoming dNTP that gets incorporated into the DNA chain whereas the b- and gphosphates are released as pyrophosphates 46 DNA synthesis and replication: General features of DNA polymerases: Thumb: positions the primer and active site Fingers: bind incoming nucleotides and fold over if base pairing is correct Palm: bind two Mg2+ ions; = catalytic site 47 DNA synthesis and replication: General features of DNA polymerases: Polymerase utilizes two metal ions to catalyze nucleotide addition: One ion (catalytic metal) is thought to lower the pKa of the 3’OH of the growing primer terminus, and promotes nucleophilic attack of the 3’O- on the a-phosphate group of the incoming dNTP The second ion (nucleotide binding metal) coordinates the triphosphate moiety and facilitates pyrophosphate dissociation. 48 Notice: This marks the boundary of where you need to study up to for the first midterm. As mentioned, midterm 1 covers from Lecture 1 to the previous slide (General features of DNA polymerase) + recap portion of next class. The material from this point forward will be covered in Midterm 2. 49 DNA synthesis and replication: E. coli DNA polymerase I vs. polymerase III: Kornberg purified the first DNA polymerase from E. coli capable of synthesizing DNA in vitro, and named it DNA pol I However, E. coli DNA pol I mutants are viable! à therefore, DNA pol I cannot be the main replicative polymerase (i.e. the polymerase responsible for replicating the E. coli genome) Instead, the main replicative polymerase in E. coli = DNA polymerase III 50 DNA synthesis and replication: E. coli DNA polymerase III holoenzyme: = multi-subunit complex that has 17 subunits. Contains four sub-assemblies: 1. The core polymerase consisting of three subunits: a (the polymerase); e (the 3'–5' exonuclease); and q (the stimulator of the 3'–5' exonuclease). 2. The sliding clamp comprising two homodimers of the b subunit, which provides the ring structure that is needed for processivity. 3. The t subunit – responsible for dimerization of the core DNA polymerase. 4. Five subunits that have clamp-loader functions — g, d, d', y and c. Helicase 51 Components of DNA pol III holoenzyme: The E. coli b-clamp: Interacts with the a (polymerase) subunit of the pol III holoenzyme. Assembles into a dimer with a 35 Angstrom diameter hole in the middle. Confers extended processivity to the DNA pol III holoenzyme – can synthesize at least 1.5 x 105 bases of the leading strand without dissociation. Also increases rate of DNA synthesis (~ 750 - 1000 nts/s). 52 Components of DNA pol III holoenzyme: Clamp is needed to keep DNA pol III on DNA: Most polymerases only synthesize a short stretch of DNA before falling off. The b-ring dimer allows the DNA pol III holoenzyme to stay on DNA and be highly processive. The clamp guide the holoenzyme and slide along the DNA duplex, but not ss DNA. The b-rings are assembled onto DNA by the clamp loaders, and the assembly/disassembly of the b-rings require ATP as energy. 53 DNA synthesis and replication: Model for E. coli replication fork: Helicase unwinds dsDNA Note opposite orientations of leading and lagging strand polymerases, and both travel in same direction as replication fork 54 DNA synthesis and replication: Model for E. coli replication fork: New primer synthesized by primase 55 DNA synthesis and replication: Model for E. coli replication fork: Primase and polymerase are both released Primase is a distributive enzyme (i.e. comes off and reloads onto DNA through interaction with DnaB) 56 DNA synthesis and replication: Model for E. coli replication fork: 57 DNA synthesis and replication: Model for E. coli replication fork: b-ring with primed DNA strand is attached to lagging strand polymerase 58 DNA synthesis and replication: E. Coli replication fork: https://dnalc.cshl.edu/resources/3d/04-mechanism-of-replicationadvanced.html 59 DNA synthesis and replication: E. Coli DNA pol III at replication fork: Model largely based on crystal structures of different components of the replication fork, and the necessity of replicating both the leading and lagging strand at the same time. Looping of the lagging strand is supported by electron micrograph (EM) pictures. 60 DNA replication and related processes: Cycle of loading and unloading DNA polymerase and clamp protein: On the leading strand, the moving DNA polymerase is tightly bound to the clamp and the two remain associated for a long time. On the lagging strand, each time the polymerase reaches the 5’ end of the proceeding Okazaki fragment, the polymerase is released, and this polymerase molecule then associates with a new clamp assembled on the RNA primer of the next Okazaki fragment. 61 DNA replication and related processes: DNA primase synthesizes RNA primers: DNA primase synthesizes RNA primers (~ 10 - 12 nt long) de novo to prime synthesis of Okazaki fragments. Primase is highly error-prone; however, synthesis of RNA primers does not require high fidelity since they eventually get removed 62 DNA replication and related processes: Removal of RNA primers: After synthesis of the Okazaki fragments, the RNA primers must be removed and replaced by DNA. This step is mediated by RNase H and DNA pol I. Ligase is needed to join Okazaki fragments. 63 DNA replication and related processes: DNA ligase seals the nicks on the lagging strand: Two classes of ligases: one uses NAD+ as cofactor (e.g E. coli), the other uses ATP as cofactor (eukaryotes). NAD = nicotinamide adenine dinucleotide NMN = nicotinamide mononucleotide NMN = nicotinamide mononucleotide 64 DNA replication and related processes: Termination of E. coli replication: The E. coli replication forks proceed in bi-directional manner until they into each other or when they hit the termination (Ter) sites. Ter is a short consensus DNA sequence (~ 23 bp) where the Tus (terminus utilization substance) protein bind. Tus is a 36 kD protein that binds DNA as a monomer. It has asymmetrical domains and its asymmetric binding to Ter enable the arrest of the replication fork progression in a directional manner. Why so many Ter sites and why this arrangment? 65 DNA replication and related processes: Termination of E. coli replication: When DnaB helicase runs into Tus-Ter from the permissive side, will displace Tus from Ter. When DnaB helicase runs into Tus-Ter from non-permissive side, will be trapped and locked. Eventually replication fork machinery disassembles (starting with the removal of the helicase) at the Tus-Ter sites. 66 DNA replication and related processes: Termination of E. coli replication: The Tus-Ter design only allows one way passage of the moving replication fork. 67 DNA replication and related processes: Fidelity of E. coli replication: Multiple factors help lower the error rate during DNA replication: This is roughly one mutation per 250 rounds of replication of the E. coli genome 68 DNA replication and related processes: Fidelity of E. coli replication: Genetics has been very useful for identifying components important for maintaining DNA replication fidelity (e.g. more Forward genetics screens): à Random mutation and isolate strains of E. coli (and other organisms) that have higher (mutator) or lower (anti-mutator) mutation rates. à Identify the genes that are mutated and study the causes of these phenotypes. Contribution of nucleotide selectivity, proofreading and DNA mismatch repair to DNA replication fidelity. The figure depicts the wide-ranging contributions of three major processes that act in series to determine DNA replication fidelity. The colored brackets illustrate the investment each process makes to the overall fidelity. Conditions that comprise each process are shown on the right 69 DNA replication and related processes: Fidelity of E. coli replication: The first proofreading step is carried out by the DNA polymerase and occurs before the new nucleotide is added to the growing chain. A) the correct nucleotide with the right geometry fit with the complementary base has higher affinity for the moving polymerase compared to the incorrect nucleotide. B) the active site of the polymerase only accommodates base pairs that have the “right” size. 70 DNA replication and related processes: Fidelity of E. coli replication: C) after nucleotide binding (but before formation of covalent bond), the polymerase undergoes an induced conformational change (closing of the “fingers”), and any incorrectly bound nucleotide is more likely to be rejected. 71 DNA replication and related processes: Fidelity of E. coli replication: Second proof-reading step mediated by the 3’ – 5’ exonuclease activity of polymerase à actively remove mis-incorporated nucleotide. 72 DNA replication and related processes: Fidelity of E. coli replication: Final proof-reading method: Strand-directed mismatch repair mechanism further lowers error rates in DNA replication. MutS detects distortion of the DNA helix and binds to a mismatched base pair. MutL binds the MutS-DNA complex and activates MutH at near-by methylated GATC to introduce a nick on the unmethylated strand of the DNA duplex. MutS/MutL scan the nearby DNA for a nick, and once detected, MutL recruits helicase to separate DNA strands and exonucleases to degrade the nicked strand all the way back pass the mismatch. DNA polymerase (pol III) comes in and fills in the gap using the methylated strand as template. 73 DNA replication and related processes: Fidelity of E. coli replication: Example of a biochemical assay for studying mismatch repair à incubate plasmid with mismatch within EcoR1 site in extracts from wild type or mutant E. coli strains and assay for repair based on sensitivity to EcoR1 cleavage. E.g.: EcoR1S = 5’GAATTC3’ EcoR1R = 3’CCTAAG5’ Biochemical assay for mismatch correction: substrate = covalently closed heteroduplex containing a mismatch within the EcoRI site. Methyl groups indicate the locations of the four GATC sites within the DNA. Cleavage of mismatch heteroduplexes with EcoRI and BamHI yields just the fulllength linear BamHI product since the hybrid EcoRI site is resistant to cutting. Mismatch correction on the strand containing the mutant EcoRI sequence renders the site sensitive to cleavage, and now treatment with both restriction endonucleases yields two fragments as indicated. 74 DNA replication and related processes: Eukaryotic DNA replication: Replication of eukaryotic cells is more complex and less well characterized. Basic steps of DNA synthesis and properties of DNA polymerases are mostly conserved between prokaryotes and eukaryotes. However, eukaryotic cells à genomes are much larger, and are chromatinized. Also, chromosomes are linear, with lots of origins of replication. DNA replication is strictly confined to S phase of cell cycle. Rate of replication is much slower (~ 75 nts/s) compared to prokaryotes(~ 1000 nts/s) à likely due to chromatin. Most chromosomal replicons do not have termination region like that of E. coli. 75 DNA replication and related processes: Eukaryotic DNA replication: Eukaryotic cells have many origins of replication. In yeast, origins are well defined and also called ARS (autonomously replicating sequences). In higher eukaryotes, origins are not well defined and difficult to identify. In higher eukaryotes, it is clear that not all potential origins of replication fire at the same time during replication. Regulation of origin firing is complex and actively investigated by research labs still. 76 DNA replication and related processes: Eukaryotic DNA replication: 1. The origin recognition complex (ORC) binds to DNA and provides a site on the chromosome where additional replication factors can associate. 2. Pre-replicative complex formation involves the association of Mcm2-7 complex with DNA at ORC. 3. Mcm2-7 proteins provide helicase activity for DNA synthesis and loading of these proteins confers competence on the origin to fire in S phase. 4. Onset of DNA synthesis requires the action of two protein kinases (cyclin dependent kinase (CDK) and Cdc7), which trigger the association of additional proteins with the origin. During the process of initiation, DNA polymerases are also recruited and DNA synthesis starts. 5. During replication, Mcm2-7 proteins move away from the origin and further assembly of pre-replicative complexes is blocked. This ensures that origins can only fire a single time 77 per cell cycle. Early G1 S G2 Simplified summary of steps in eukaryotic DNA replication DNA replication and related processes: Eukaryotic DNA replication: Once origin of replication fires, events/proteins at the replication forks are similar to that of E. coli. Pol a = primase + another DNA polymerase activity. Single strand binding protein is called RPA (Replication protein A). PCNA = b ring of DNA pol III. Eukaryotic clamp loader is called RFC (Replication factor C), and is highly similar to the prokaryotic clamp loader complex. Recent findings showed that leading strand is synthesized by Pol e whereas lagging strand switches between Pol a and Pol d. 78 DNA replication and related processes: Eukaryotic DNA replication: Main eukaryotic polymerases (there are additional ones): For base excision repair 79 DNA replication and related processes: Eukaryotic DNA replication: Cycles of switching between DNA Pol a and Pol d on the lagging strand: 1. Pol a synthesizes RNA primer (10 – 12 nts) and then ~30 nts of DNA 2. RFC displaces Pol a and recruits PCNA with Pol d 3. PCNA clamps Pol d on DNA 4. Pol d elongates Okazaki fragment synthesis 80 DNA replication and related processes: Eukaryotic DNA replication: DNA polymerase switching and processing of an Okazaki fragment on the lagging strand. A.As the DNA helicase promotes unwinding at the replication fork, DNA pol e with RFC and PCNA synthesizes DNA on the leading strand. DNA pol α initiates synthesis on the lagging strand by generating an RNA primer (red segment) followed by a short segment of DNA. Then, RFC and PCNA load a second DNA polymerase (d) to continue synthesis of the Okazaki fragment. A.As DNA pol d approaches the downstream Okazaki fragment, cleavage by RNase H1 removes the initiator RNA primer leaving a single 5′-ribonucleotide. Then, FEN1/RTH1 removes the last 5′-ribonucleotide. The resulting nick is sealed by DNA ligase. 81 DNA replication and related processes: Comparison of prokaryotic and eukaryotic DNA replication proteins: Note that PCNA is made up of homo-trimer whereas b-clamp is made up of dimer 82 DNA replication and related processes: Eukaryotic DNA replication: Termination of DNA replication in eukaryotes – not well defined. End when replication forks run into each other? 83 DNA replication and related processes: DNA replication in the context of chromatin: Eukaryotic DNA replication is even more complicated given presence of chromatin and nucleosomes. Need to disassemble chromatin ahead of the replication fork, and re-assemble nucleosomes post DNA replicaiton. PCNA directly binds to chromatin remodeling complexes such as CAF-1. 84 DNA replication and related processes: Fidelity of DNA replication in eukaryotes: Basically same as in prokaryotes, however, larger selection of polymerases with different error rates. High fidelity polymerases Low fidelity polymerase for by passing DNA lesions 85 DNA replication and related processes: Eukaryotic mismatch repair: Again, similar to prokaryotic system except not methyl-directed. Initiated by nick on one strand à = repaired strand 86