2023-24 Introduction to Metabolism (King's College London) PDF
Document Details
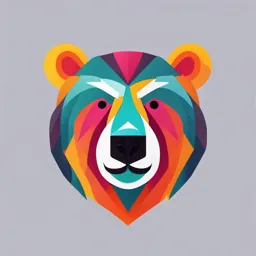
Uploaded by StableEpilogue
King's College London
2023
King's College London
Dr. Lauren Albee
Tags
Related
Summary
King's College London presentation slides on introduction to metabolism. The presentation covers learning outcomes, diagrams, and key metabolic pathways (glycolysis, TCA cycle, etc).
Full Transcript
Faculty of Life Sciences & Medicine Dr. Lauren Albee ([email protected] 4MBBS101: NAM ) Department of Biochemistry Introduction to Metabolism Introduction to Metabolism 4MBBS101: NAM...
Faculty of Life Sciences & Medicine Dr. Lauren Albee ([email protected] 4MBBS101: NAM ) Department of Biochemistry Introduction to Metabolism Introduction to Metabolism 4MBBS101: NAM Dr. Lauren Albee Biochemistry and Molecular Biology Chapter 3 and 12 Available as an e-textbook at https://bibliu.com/app/#/signinPage Essential Cell Biology Chapter 3 and Chapter 13 2 Learning outcomes After this lecture you should be able to: a) Define the terms: metabolism, anabolism, and catabolism b) Give examples of anabolic and catabolic pathways. c) Explain the concept of controlled release of energy in enzyme catalysed metabolic pathways. d) Outline the role of activated carrier molecules in energy metabolism and give examples of carrier molecules. e) Recognise the general roles of NAD/NADH, NADP/NADH, FAD/FADH2, Coenzyme A/ acetyl CoA. f) Explain the key function of ATP as the 'energy currency of living cells g) Draw a summary diagram that relates the main energy releasing metabolic pathways (glycolysis, TCA cycle, β- oxidation, oxidative phosphorylation) to each other h) Indicate the subcellular locations of each of the pathways in eukaryotic cells. 3 Reminder: eukaryotic cell structure Structure of mitochondria Intermembrane space https://www.novusbio.com/research-areas/cellular-markers/mitochondrial-markers.html 5 Summary of mitochondrial events Definition of metabolism ‘The totality of the chemical reactions and physical changes that occur in living organisms, comprising anabolism and catabolism’ Oxford Dictionary of Biochemistry and Molecular Biology The enzyme reactions of synthesis, breakdown and interconversion of essential biomolecules 7 Overview of Metabolism: catabolism Catabolism The metabolic breakdown of complex substances into smaller products - - including the breakdown of carbon compounds with the liberation of energy for use by the cell or organism Source: Essential Cell Biology. Alberts, Bray, Johnson, Hopkin, Lewis, Raff, Roberts & Walter 8 Copyright 2004 © Garland Science Publishing Overview of Metabolism: anabolism Anabolism The energy requiring part of metabolism in which simpler substances are transformed into more complex ones - as in growth or other biosynthetic processes. Source: Essential Cell Biology. Alberts, Bray, Johnson, Hopkin, Lewis, Raff, Roberts & Walter 9 Copyright 2004 © Garland Science Publishing Metabolism Catabolism Anabolism names end in ‘lysis’ names end in ‘genesis’ glycolysis gluconeogenesis lipolysis lipogenesis glycogenolysis glycogenesis generate ATP & NADH Use ATP, GTP, UTP (mitochondria) mostly in cytosol 10 Why are metabolic pathways so complicated? 11 Stepwise breakdown releases energy in useable small ‘packages’ 12 Pathways can be regulated by regulation of specific enzymes A BB CC DD E enzyme 1 enzyme 2 enzyme 3 enzyme 4 A B C D E enzyme 1 enzyme 2 enzyme 3 enzyme 4 13 Different ‘forward’ and ‘reverse’ pathways allow separate regulation Activating enzyme 1 (and/or enzyme 2) would speed up forward and reverse A B C pathways – a ‘futile cycle’ enzyme 1 enzyme 2 Activating enzyme 1 speeds up forward pathway A enzyme 1 B enzyme 2 C Activating enzyme 3 speeds up reverse pathway enzyme 4 enzyme 3 D 14 Energy released at each step is stored in activated carrier molecules 15 Some activated carrier molecules used in metabolism 16 Importance of ATP Most of the metabolic pathways covered in the Year 1 syllabus are catabolic pathways that result in ATP synthesis 17 ATP as the energy currency of the cell Adenosine triphosphate NH2 O O Chemically stable at pH 6-9 Structural features recognised by specific proteins, enzymes, etc O- _ Hydrolysis gives ADP + O=P – OH + H+ + ‘energy’ _ O- (Pi) 18 Breakdown of ATP to ADP phosphoanhydride bonds energy available for cellular work and for chemical synthesis Inorganic phosphate (Pi) ΔG is - 31 to -50 kJ mole-1 19 What makes the hydrolysis of ATP energetically so favourable? phosphoanhydride bonds Relieves electrostatic repulsion between phosphate groups The released phosphate ion is resonance stabilised: increased entropy (ΔS +ve) Concept of a ‘high energy bond’ or ‘high energy phosphate group’ old-fashioned but still useful as a quick way of describing this. 20 Hydrolysis of ATP to AMP and PPi releases almost twice as much free energy as ATP to ADP hydrolysis 21 Functions of ATP (1) Used directly in cell motility and muscle contraction (motor proteins) ATP ADP + Pi myosin/ dynein 22 Functions of ATP (2) Used in active transport systems e.g. Na+ / K+ pumps 23 Functions of ATP (3) Used in metabolic control – regulates enzyme activity 24 Functions of ATP (4) Used in metabolism to add Pi to metabolic intermediates Glucose + ATP Glucose-6-phosphate + ADP 25 Other ‘high energy’ nucleotides Other ‘high energy’ nucleotide carriers are used to drive specific biosynthetic reactions: UTP drives the synthesis of complex sugars GTP drives the synthesis of proteins 26 Some activated carrier molecules used in metabolism 27 Role of NAD+ as H atom acceptor NAD+ NADH + H+ 2H addition 2H removal NAD = Nicotinamide Adenine Dinucleotide 28 Reminder – atomic structure nucleus proton neutron + - - + electron An atom (no overall charge) Reminder – atomic structure + - Hydrogen atom 1 proton (H+) + 1 electron (e-) Transfer of a H atom means transferring H+ and e- Transfer of 2 H = transfer of 2 H+ and 2 e- H+ + e- (= H) H+ (released to solution) e- (Transferred with the H, but ends up here) NAD+ NADH + H+ When two H atoms are transferred to NAD+ from a donor, one proton (H+) is released to solution. Reminder: reduction and oxidation NAD+ NADH + H+ reduction oxidation NADP replaces NAD in anabolic reactions e.g. lipid synthesis NAD+ NADP+ 33 Flavin adenine dinucleotide ADENINE FAD RIBOSE Flavins act as H acceptors FAD + 2H FADH2 Some activated carrier molecules used in metabolism 36 Acetyl-CoA 37 Acetyl-CoA Blob that you do not need to worry about CoenzymeA (CoA) is a blob* you do not need to worry about. *not a scientific term. It is actually a dinucleotide with a vitamin and sulphur-containing group. 38 Acetyl-CoA 2 carbons carried here Blob that you do not need to worry about Acetyl-CoA is a carrier of an acyl group (2 carbons), and not electrons or hydrogens. 39 Acetyl-CoA Some of the energy from oxidation of glucose and fatty acids stored here Blob that you do not need to worry about A ‘high energy’ thioester bond links the acetyl group to Coenzyme A 40 Metabolic reactions require: Fuel molecules (substrates / Intermediates) + Enzyme catalysts + Cofactors Coenzymes Activating ions / Mg2+ Zn2+ prosthetic Cl- group Examples of Enzyme Cofactors ATP ADP + Pi ATP acts as a ‘high energy’ cofactor for kinase enzymes ATP breakdown releases approx 31 kJ of energy per mole Summary Diagrams Biochemistry textbooks show summary diagrams of metabolic pathways with links to other main metabolic processes Cell Biology textbooks show summary diagrams with the cellular location of the main metabolic pathways Catabolism of sugars, fats & amino acids occurs in 3 stages Cell Structure Cytosol Mitochondria Summary of mitochondrial events MCQ 1 1. How do enzymes catalyse biological reactions? They: A. increase the activation energy B. alter the equilibrium of the reaction C. stabilise the transition state D. stabilise the substrate/s E. stabilise the product/s 47 MCQ 2 2. A metal ion that is required for an enzyme’s activity is termed a: A. cofactor B. co-enzyme C. co-substrate D. iron-sulphur centre E. prosthetic group 48 MCQ 3 3. What is the correct definition of catabolism? A. A series of reactions that leads to the breakdown of amino acids only B. A series of reactions that form complex products from smaller starting reactants. C. The enzyme reactions of synthesis, breakdown, and interconversion of essential biomolecules D. The enzymatic reactions within a cell that lead to the breakdown of complex products into smaller products. E. A series of reactions that leads to the formation of cellulose in animal cells. 49 MCQ 4 4. Which of the following features generally tend to increase the number of catalytic events an enzyme accomplishes per second? A. More positive free energy of binding between enzyme and substrate B. Increased strength of binding between enzyme and product C. Increased temperature D. Increased diffusion rate of substrate E. Increased salt concentration 50 4. Which of the following features generally tend to increase the number of catalytic events an enzyme accomplishes per second? A. More positive free energy of binding between enzyme and substrate B. Increased strength of binding between enzyme and product C. Increased temperature D. Increased diffusion rate of substrate E. Increased salt concentration k1 k2 E + S ⇌ ES E + P k-1 N.B. Negative free energy favours a reaction 51 EXTRA REVISION MATERIAL These following slides are a preview of what is to come during our metabolism lectures. I will not be covering them in lecture (unless we finish super early), but they are a summary of the events to help you revise. 52 Glucose Metabolism Glucose is metabolised via the glycolysis pathway + pyruvate pyruvate glucose Glucose (6 carbons) is oxidised to 2 molecules of pyruvate (3 carbons) in a pathway known as glycolysis. Glucose is trapped in the cell by phosphorylation to glucose 6- phosphate glucose glucose 6-phosphate Glucose enters the cell, where it is trapped by phosphorylation to glucose 6-phosphate (the negative charges on the phosphate group prevents the molecules diffusing back across the lipid membrane). A series of enzymatic reactions then oxidise glucose 6-phosphate to pyruvate. Aerobic and anaerobic metabolism Aerobic metabolism: if sufficient O2 is available, pyruvate is transported into the mitochondria, where it undergoes irreversible oxidative decarboxylation, losing CO2 (1 carbon) and forming acetyl-CoA (carrier of 2 carbons). Anaerobic metabolism: if there is a Lack of O2 lack of oxygen, pyruvate is reduced (anaerobic metabolism) pyruvate lactate to lactate, with simultaneous (3 carbons) (3 carbons) oxidation of the co-factor NADH (to (2 x pyruvate from 1 x glucose) NAD+). Sufficient O2 (aerobic metabolism) Cytoplasm Glucose: a summary Fatty Acid Metabolism Fatty acids are activated in the cytosol O CH3 – (CH2)n – CH2 – CH2 – C – O – H Fatty acid H H O CH3 – (CH2)n – C – C – C – S-CoA Fatty acyl-CoA H H Fatty acids are activated in the cytoplasm by the addition of coenzyme A (CoA). Fatty acyl-CoA molecules are then transported into the mitochondria. Fatty acyl-CoA molecules are metabolised via the β-oxidation pathway Fatty acyl-CoA (16 carbons) β-oxidation + Fatty acyl-CoA (14 carbons) acetyl-CoA (carrier of 2 carbons) In the mitochondria, fatty acyl-CoA molecules are metabolised by the β- oxidation pathway: they are ‘chopped up’ into 2 carbon units carried by acetyl-CoA. Fatty acids: a summary Both the glycolysis and β-oxidation pathways produce acetyl- CoA. (i.e. oxidation of both glucose and fatty acids produces acetyl-CoA) How is the acetyl-CoA used to produce ATP? TCA cycle The TCA cycle Acetyl-CoA (carrier of 2 carbons) condenses with oxaloacetate (4 carbons) to form citrate (6 carbons). The TCA cycle The TCA cycle is a series of enzymatic reactions. In these reactions: 2 carbons are lost as CO2 oxaloacetate is reformed, ready to condense with another molecule of acetyl-CoA. The TCA cycle The TCA cycle produces 3 molecules of NADH + H+ and 1 molecule of FADH2 for each ‘turn’ of the cycle. Reminder: NADH and FADH2 are electron carriers. Electron Transport Chain The electron transport chain The reduced electron carriers NADH and FADH2 produced by the TCA cycle are oxidised by the electron transport chain. Mitochondrion The electron transport chain The electron transport chain is a series of protein complexes embedded in the inner mitochondrial membrane. Electrons are transported along the chain (i.e. from one protein complex to the next), before finally they reduce O2 forming H2O (i.e. oxygen is the final electron acceptor). Mitochondrion The electron transport chain The energy released by the transfer of electrons is used by the protein complexes to pump protons (H+) into the intermembrane space, creating an electrochemical gradient (lots of H+ in the intermembrane space, fewer H+ in the mitochondrial matrix). matrix Mitochondrion intermembrane space The electron transport chain Protons flow back through the membrane via a protein complex called ATP Synthase, driving the synthesis of ATP from ADP + Pi. matrix Mitochondrion ATP synthase intermembrane space Amino Acid Metabolism Amino acid metabolism (often called ‘nitrogen metabolism’) Amino acids that are surplus to requirements are not stored. Instead, the N from the α‑amino group is removed and the remaining carbon skeleton is further metabolised. Amino acid metabolism is complex and varied, but we will cover the general principles in this lecture. Deamination: removal of N glutamate α-ketoglutarate (An example of a NH ↓ 4 + ‘carbon skeleton’) Removed from body via urea cycle The liver The removal of N from amino acids is called ‘deamination’. Only amino acid to undergo a meaningful level of deamination in humans is glutamate (1 N) - this deamination occurs in the liver. Transamination: conversion of one amino acid to another In most tissues, amino acids are converted into glutamate by a process call ‘transamination’. Amino acid + α-ketoglutarate ↔ ketoacid + glutamate Example: alanine + α-ketoglutarate ↔ pyruvate + glutamate (is a ketoacid) Glutamine carries 2 nitrogen atoms Glutamine is a carrier of 2 nitrogen atoms (2 N). In the tissues, glutamate (1 N) is converted to glutamine (2 N), which is NH4+ released into the bloodstream as a safe carrier of 2 N to the liver for deamination. Glutamine is formed from glutamate by addition of NH2 (from NH4+). Glutamate reformed from glutamine in the liver In the liver, glutamate (1 N) is reformed from glutamine (2 N), with the loss of NH4+. Glutamate is then deaminated to α-ketoglutarate, releasing NH4+. The NH4+ molecules are removed from the body by the urea cycle (which occurs in the liver). glutamine glutamate α-ketoglutarate (An example of a NH4+ NH4+ ‘carbon skeleton’) Removed from body via urea cycle The liver In most tissues: amino acid + α-ketoglutarate ↔ ketoacid + glutamate (Transamination NH4+ ) glutamine The bloodstream (Deamination) glutamine glutamate α-ketoglutarate (An example of a NH4+ NH4+ ‘carbon skeleton’) Removed from body via urea cycle Amino acids: The liver a summary