MP & Neuro Physiology PDF
Document Details
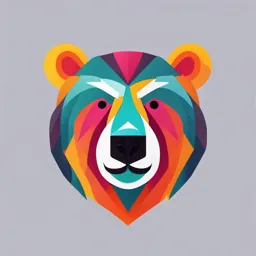
Uploaded by ProminentParticle
German Jordanian University
Dr. Abbas Al-Momany, (Ph.D)
Tags
Summary
These notes describe the membrane potential, focusing on the role of ions like K+ and Na+. It also explores the effect of fixed anions and the concept of equilibrium potentials in cellular processes.
Full Transcript
The Membrane Potential Instructor: Dr. Abbas Al-Momany, (Ph.D) A.The Membrane Potential There is a difference in charge on each side of the plasma membrane due to Permeability properties of the membrane Action of Na+/K+ pumps Negatively charg...
The Membrane Potential Instructor: Dr. Abbas Al-Momany, (Ph.D) A.The Membrane Potential There is a difference in charge on each side of the plasma membrane due to Permeability properties of the membrane Action of Na+/K+ pumps Negatively charged molecules inside the cell This difference in charge is called a potential difference The potential difference make the inside of the cell is negative compared to the outside. Effect of Fixed Anions on Distribution of Cations Cellular proteins and the phosphate groups of ATP and other organic molecules (Fixed + + + Anions) are negatively charged at the pH of Electrical attraction Plasma membrane the cell cytoplasm. These negative ions ( anions ) are “fixed” Fixed anions within the cell because they cannot – penetrate the plasma membrane. + + + + + + In this way, fixed anions within the cell influence the distribution of inorganic cations (mainly K , Na , and Ca ) between + + + the extracellular and intracellular Concentration gradient compartments. 4.Membrane Potential: K+ K+ accumulates at high concentrations in the cell because: 1)The Na+/K+ pumps actively bring in K+. 2)The membrane is highly permeable to K+. 3)Negative anions inside the cell attract cations outside the cell. The K+ concentration inside is 150 mEq/L and out is 5 mEq/L As a result of the unequal distribution of charges between the inside and outside of cells, each cell acts as a tiny battery with the positive pole outside the plasma membrane and the negative pole inside B.Equilibrium Potentials No net movement of the charged ions in & out of the cell Even with all the K+ inside the cell, the negative molecules inside and all of the sodium outside, the cell is more negative inside compared to outside. This potential difference can be measured as a voltage. Because the membrane is so permeable to K+, this difference is often maintained by K+ concentration gradient. it is of critical importance in muscle contraction, the regulation of the heartbeat, and the generation of nerve impulses 2.K+ Equilibrium Because the plasma membrane is usually more permeable to K than to any other ion, the membrane potential is usually determined primarily by the K concentration gradient. Addressing just K+, the electrical attraction would pull K+ into the cell until it reaches a point where the concentration gradient drawing K+ out matches this pull in. 1) K+ would reach an equilibrium, with more K+ inside than outside. 2) Normal cells have 150mM K+ inside and 5mM K+ outside. The resulting potential difference measured in voltage would be the equilibrium potential (EK) for K+; measured at −90mV. 1) This means the inside has a voltage 90mV lower than the outside. 2) This is the voltage needed to maintain 150 mM K+ inside and 5mM K+ outside. Nernst Equation Nernst equation :calculate equilibrium potential for a particular ion when its concentrations are known 3.Na+ Equilibrium Sodium is also an important ion for establishing membrane potential. The concentration of sodium in a normal cell is 12mM inside and 145mM outside. To keep so much sodium out, the inside would have to be positive to repel the sodium ions. The equilibrium potential for sodium is +66mV. The membrane is less permeable to Na+, so the actual membrane potential is closer to that of the more permeable K+. Concentration of ions in the intracellular and extracellular fluids C.Resting Membrane Potential Membrane potential of a cell NOT producing any impulses. Depends on: a. Ratio of the concentrations of each ion on either side of the membrane b.Specific permeability to each ion K+, Na+, Ca2+ and Cl− contribute to the resting potential. Membrane potential of a cell not producing any impulses a. Because the membrane is most permeable to K+, it has the greatest influence. b.A change in the permeability of the membrane for any ion will change the resting potential. c. A change in the concentration of any ion inside or outside the cell will change the resting potential. d.Key to how neurons work In most cells, the resting potential is between -65mV and -85mV. a. Neurons are usually at −70mV. b.Close to K+ equilibrium potential When a neuron sends an impulse, it changes the permeability of Na+, driving the membrane potential closer to the equilibrium potential for Na+. Resting Membrane Potential –70 mV Voltmeter + – Fixed anions – Na+ Na+ K+ K+ Copyright © The McGraw-Hill Companies, Inc. Permission required for reproduction or display. 6.Role of Na+/K+ Pump 1. Acts to counter K+ leaking out 2. It transports 2 K+ in for every 3 Na+ out to maintain the voltage difference. 3. Keeps both the resting potential and the concentration differences stable – electrogenic effect Processes that influence the resting membrane potential The Nervous System Instructor: Dr. Abbas Al-Momany, (Ph.D) 15 Introduction to the Nervous System Nervous System divided into: 1. Central nervous system: brain and spinal cord 2. Peripheral nervous system: cranial and spinal nerves Nervous tissue is composed of two types of cells: 1. Neurons that conduct impulses but generally can not divide. 2. Glial cells (neuroglia) that support the neurons and can not conduct impulses, but can divide B.Neurons Neurons are structural and functional units of the nervous system General functions A. Respond to chemical and physical stimuli B. Conduct electrochemical impulses C. Release chemical regulators D. They enable perception of sensory stimuli, learning, memory, and control of muscles and glands Most can not divide, but can repair Unlike neurons glial cells are able to divide by mitosis. This helps to explain why brain tumors in adults are usually composed of glial cells rather than of neurons. General structure of neurons Neurons vary in size and shape, but they all have: Dendrites Axon hillock Direction of 1) A cell body that contains the nucleus, Nissl bodies, conduction and other organelles; cluster in groups called nuclei in the CNS and ganglia in the PNS. Nissl bodies are Collateral axon composed of large stacks of rough endoplasmic (a) reticulum that are needed for the synthesis of Cell body motor neuron membrane proteins. Axon 2) Dendrites: (from the Greek dendron = tree branch) are thin, branched processes that extend from the Ax on cytoplasm of the cell body. Dendrites provide a Direction of conduction receptive area that transmits graded electrochemical (b) impulses to the cell body sensory neuron 1) Axon: The axon is a longer process that conducts Dendrites impulses, called action potentials away from the cell body Axons 1) Vary in length from a few millimeters to a meter 2) Connected to the cell body by the axon hillock where action potentials are generated at the initial segment of the axon. 3) Covered in myelin with open spots called nodes of Ranvier Classification of Neurons and Nerves Functional classification of neurons – based on direction impulses are conducted 1) Sensory neurons or afferent: conduct impulses from sensory receptors to the CNS 2) Motor neurons or efferent neurons: conduct impulses from the CNS to target organs (muscles or glands) 3) Association/interneurons: located completely within the CNS and integrate functions of the nervous system Classification of Neurons and Nerves Motor Neurons There are two types of motor neurons: 1) Somatic motor neurons: responsible for reflexes and voluntary control of skeletal muscles 2) Autonomic motor neurons: innervate involuntary targets such as smooth muscle, cardiac muscle, and glands. The cell bodies of the autonomic neurons that innervate these organs are located outside the CNS in autonomic ganglia. Two subdivisions of autonomic neurons: A. Sympathetic – emergency situations; “fight or flight” B. Parasympathetic – normal functions; “rest and digest” Functional Categories of Neurons Central Nervous System (CNS) Peripheral Nervous System (PNS) Association neuron (interneuron) Sensory neuron Receptors Somatic motor neuron Skeletal muscles Autonomic motor neurons Smooth muscle Cardiac muscle Glands Autonomic ganglion Structural Classification of Neurons Based on the number of processes that extend from the cell body neurons are classified into: 1) Pseudounipolar: (from the Late Latin pseudo = false) single short process that branches like a T to form 2 longer processes; sensory neurons 2) Bipolar neurons: have two processes, one on either end; found in retina of eye 3) Multipolar neurons: several dendrites and one axon; most common type Structural Classification of Neurons Pseudounipolar Dendritic branches Bipolar Dendrite Multipolar Axon Dendrites Copyright © The McGraw-Hill Companies, Inc. Permission required for reproduction or display. Nerve A nerve is a bundle of axons located outside the CNS. Most nerves are composed of both motor and sensory fibers and are thus called mixed nerves. Some of the cranial nerves, however, contain sensory fibers only. These are the nerves that serve the special senses of sight, hearing, taste, and smell. A bundle of axons in the CNS is called a tract. Neuroglia (glial cells) Cells that are non-conducting but support neurons Two types are found in the PNS: 1) Schwann cells (neurolemmocytes): form myelin sheaths around peripheral axons 2) Satellite cells (ganglionic gliocytes): support cell bodies within the ganglia of the PNS Four types are found in the CNS: 1) Oligodendrocytes: form myelin sheaths around the axons of CNS neurons 2) Microglia: migrate around CNS tissue and phagocytize foreign and degenerated material 3) Astrocytes: They regulate the external chemical environment of neurons by removing excess potassium ions, and recycling neurotransmitters released during synaptic transmission. Astrocytes may regulate vasoconstriction and vasodilation by producing substances such as arachidonic acid, 4) Ependymal cells: line the ventricles and secrete cerebrospinal fluid Electrical Activity in Axons: Resting Membrane Potential Neurons have a resting potential of −70mV. A. Established by large negative molecules inside the cell B. Na+/K+ pumps C. Permeability of the membrane to positively charged, inorganic ions At rest, there is a high concentration of K+ inside the cell and Na+ outside the cell. Altering Membrane Potential 1. Although all cells have a membrane potential, only a few types of cells have been shown to alter their membrane potential in response to stimulation 2. Neurons and muscle cells can change their membrane potentials. This is called excitability or irritability 3. Caused by changes in the permeability to certain ions 4. An increase in membrane permeability to a specific ion results in the diffusion of that ion down its electrochemical gradient , either into or out of the cell.. 5. Flow of ions are called ion currents which occur in limited areas where ion channels are located Changes in Membrane Potential 1) At rest, a neuron is considered polarized when the inside is more negative than the outside. 2) When the membrane potential inside the cell increases (becomes more positive), this is called depolarization. 3) A return to resting potential is called repolarization. 4) When the membrane potential inside the cell decreases (becomes more negative), this is called hyperpolarization. 5) Depolarization occurs when positive ions enter the cell (usually Na+). 6) Hyperpolarization occurs when positive ions leave the cell (usually K+) or negative ions (Cl−) enter the cell. 7) Depolarization of the cell is excitatory. 8) Hyperpolarization is inhibitory. Changes in Membrane Potential Copyright © The McGraw-Hill Companies, Inc. Permission required for reproduction or display. Axon Recording electrodes Changes can be recorded on an oscilloscope by recording the voltage mV +60 inside and outside the +40 cell. 0 –40 Depolarization (stimulation) –60 rmp –80 Hyperpolarization (inhibition) Ion Gating in Axons Ions such as Na+ , K + , and others pass through ion channels in the plasma membrane that are said to be gated channels. Changes in membrane potential are controlled by changes in the flow of ions through channels. A. K+ has two types of channels: 1) Not gated (always open); sometimes called K+ leakage channels 2) Voltage-gated K+ channels; open when a particular membrane potential is reached; closed at resting potential B. Na+ has only voltage-gated channels that are closed at rest; the membrane is less permeable to Na+ at rest. Voltage-Gated Na+ Channels 1) These channels open if the membrane potential depolarizes to −55mV. This is called the threshold. 2) Sodium rushes in due to the electrochemical gradient. 3) Membrane potential climbs toward sodium equilibrium potential. 4) These channels are deactivated at +30mV. A Voltage-Gated K+Ion Channel 1) At around +30mV, Channel closed at resting membrane Channel open by depolarization voltage-gated K+ potential (action potential) channels open, and K+ rushes out of the cell following the electrochemical gradient. Channel inactivated during refractory period 2) This makes the cell repolarize back toward the potassium equilibrium potential. Action Potentials 1. At threshold membrane potential (−55mV), voltage-gated Na+ channels open, and Na+ rushes in. As the cell depolarizes, more Na+ channels are open, and the cell becomes more and more permeable to Na+. A. This is a positive feedback loop. B. Causes an overshoot of the membrane potential C. Membrane potential reaches +30mV. D. This is called depolarization 2.At +30mV, Na+ channels close, and K+ channels open. A. Results in repolarization of membrane potential B. This is a negative feedback loop Depolarization of an Axon More depolarization + Membrane potential +30 depolarizes from Action –70 mV to +30 mV potential Voltage regulated Na+ diffuses Na+ gates open into cell 0 1 1 2 Membrane Na+ in K+ out potential (millivolts) Depolarization stimulus Threshold –50 – Less depolarization Membrane potential –70 2 repolarizes from Stimulus +30 mV to –70 mV Resting Voltage regulated K+diffuses membrane K+ gates open out of cell potential 0 1 2 3 4 5 6 7 Time (msec) These changes in Na+ and K+ diffusion and the resulting changes in the membrane potential they produce constitute an event called the action potential, or nerve impulse. Action Potentials Copyright © The McGraw-Hill Companies, Inc. Permission required for reproduction or display. Sodium equilibrium potential +30 Membrane potential (millivolts) Caused by Na+ diffusion into axon 0 Caused by K+ diffusion out of axon –50 Resting membrane potential –70 Potassium 0 1 2 3 4 equilibrium Time (milliseconds) potential 1. Gated Na+ channels open 2a. Inactivation of Na+ Na+ and K+ diffusion channels begins 2b. Gated K+ channels open 3. Inactivation of K+ channels begins 4. Gated Na+ and K+ channels closed 0 1 2 3 4 Time (milliseconds) After-Hyperpolarization 1) Repolarization actually overshoots resting potential and gets down to −85mV. 2) This does not reach potassium equilibrium potential because voltage- gated K+ channels are inactivated as the membrane potential falls. 3) Na+/K+ pumps quickly reestablish resting potential. All-or-None Law a. Once threshold has been reached, an action potential will happen. b. The size of the stimulus will not affect the size of the action potential; it will always reach +30mV. c.The size of the stimulus will not affect action potential duration. (-70 mV +30 mV -70 mV) = 3 msec 40 All-or-None Law The amplitude (size) of action potentials is all or-none. When depolarization is below a threshold value, the voltage-regulated gates are closed; when depolarization reaches threshold, a maximum potential change (the action potential) is produced Because the change from -70 mV to+30 mV and back to - 70 mV lasts only about 3 msec, the image of an action potential on an oscilloscope screen looks like a spike. Action potentials are therefore sometimes called spike potentials Coding for Stimulus Intensity A stronger stimulus will make action potentials occur more frequently. (frequency modulated) A stronger stimulus may also activate more neurons in a nerve. This is called recruitment. Refractory Periods If a stimulus of a given intensity is maintained at one point of an axon and depolarizes it to threshold, action potentials will be produced at that point at a given frequency (number per second). As the stimulus strength is increased, the frequency of action potentials produced at that point will increase accordingly. As action potentials are produced with increasing frequency, the time between successive action potentials will decrease—but only up to a minimum time interval. The interval between successive action potentials will never become so short as to allow a new action potential to be produced before the preceding one has finished. During the time that a patch of axon membrane is producing an action potential, it is incapable of responding to further stimulation= is refractory If a second stimulus is applied during most of the time that an action potential is being produced, the second stimulus will have no effect on the axon membrane. The membrane is thus said to be in an absolute refractory period; it cannot respond to any subsequent stimulus. Refractory Periods A. Action potentials can only increase in frequency to a certain point. There is a refractory period after an action potential when the neuron cannot become excited again. B. The absolute refractory period occurs during the action potential. Na+ channels are inactive (not just closed). C. The relative refractory period is when K+ channels are still open. Only a very strong stimulus can overcome this. D. Each action potential remains a separate, all-or-none event. Refractory Periods During the time that the Na channels are in the process of recovering from their inactivated state and the K channels are still open, the membrane is said to be in a relative refractory period Cable Properties of Neurons Copyright © The McGraw-Hill Companies, Inc. Permission required for reproduction or display. A. The ability of neurons to Threshold conduct charges through their cytoplasm –60 mV B. if the depolarization is below threshold (about -55 mV) the –70 mV change in membrane potential will be localized to within 1 to 2 mm of the point of + + + + + + + + – – – – – – – – stimulation Axon + C. Poor due to high internal – – – – – – – – resistance to the spread of + + + + + + + + charges and leaking of charges through the membrane D. Neurons could not depend on cable properties to move an + Injection of positive impulse down the length of an charges (depolarization) axon. by stimulating electrode Conduction of Nerve Impulses Copyright © The McGraw-Hill Companies, Inc. Permission required for reproduction or display. Axon 1. When an action potential occurs at a given point on a neuron membrane, voltage- gated Na+ Action potential begins – – + + + + channels open as a wave down + + – – – – 1 Na+ Axon the length of the axon. + + – – – + – + – + – + Action potential K+ is regenerated here + + – – + + 2. The action potential at one – – + + – – 2 Na+ location serves as the – + – + + + – – – + – + depolarization stimulus for the K+ Action potential is+ regenerated here next region of the axon + + + K + – – – – – – + + 3 Na+ – – – – + + + + + + – – K+ = Resting potential = Depolarization = Repolarization Conduction in an unmyelinated neuron In an unmyelinated axon, every patch of membrane that contains Na and K channels can produce an action potential. Action potentials are thus produced along the entire length of the axon. The amplitude of each action potential is the same – conducted without decrement (without decreasing in amplitude). Because action potentials must be produced at every fraction of a micrometer in an unmyelinated axon, the conduction rate is relatively slow. The conduction rate is substantially faster if the axon is myelinated, because fewer action potentials are produced along a given length of myelinated axon. Conduction in a myelinated neuron A. Myelin provides insulation, improving the speed of cable properties. B. Nodes of Ranvier allow Na+ and K+ to cross the membrane every 1−2 mm. C. Na+ ion channels are concentrated at the nodes D. Action potentials “leap” from node to node. E. This is called saltatory conduction. Conduction in a Myelinated Neuron Action Action potential potential was here was here Na+ Myelin + –– + + – – – ++ – ++ – – + –– + + Axon Na+ = Resting potential = Depolarization = Repolarization Action Potential Conduction Speed A. Increased by: 1) Increased diameter of the neuron. This reduces resistance to the spread of charges via cable properties. 2) Myelination because of saltatory conduction B. Examples 1) Thin, unmyelinated neuron speed 1.0m/sec 2) Thick, myelinated neuron speed 100m/sec The Synapse A. A synapse is the functional connection between a neuron and the cell it is signaling A. In the CNS, this second cell will be another neuron. B. In the PNS, the second cell will be in a muscle or gland; often called myoneural or neuromuscular junctions B. If one neuron is signaling another neuron, the first is called the presynaptic neuron, and the second is called the postsynaptic neuron. A. A presynaptic neuron can signal the dendrite, cell body, or axon of a second neuron. B. There are axodendritic, axosomatic, and axoaxonic synapses. C. Most synapses are axodendritic and are 1 direction C. Synapses can be electrical or chemical Types of Synaptic Connections 1- Electrical Synapses Cells are joined by gap junctions. In gap junctions, the membranes of the two cells are Copyright © The McGraw-Hill Companies, Inc. Permission separated by only 2 nanometers. required for reproduction or display. Cytoplasm Ions and molecules to pass from one cell to the other. Plasma membrane Stimulation causes of one cell phosphorylation or Plasma dephosphorylation of connexin membrane proteins to open or close the of adjacent channels cell Two cells, Connexin Electrical synapses occur in interconnected proteins smooth muscle and cardiac by gap forming gap muscle, between some neurons junctions Cytoplasm junctions of the brain, and between glial cells. 2. Chemical Synapses Copyright © The McGraw-Hill Companies, Inc. Permission required for reproduction or display. Most synapses involve the release of a chemical called a neurotransmitter from the axon’s terminal buttons. Mitochondria Terminal The synaptic cleft is very bouton of axon small, and the presynaptic and postsynaptic cells are Synaptic held close together by cell vesicles adhesion molecules Synaptic cleft (CAMs). Postsynaptic cell (skeletal muscle) © John Heuser, Washington University School of Medicine, St. Louis, MO Release of Neurotransmitter A. Neurotransmitter is enclosed in synaptic vesicles in the axon terminal. 1)When the action potential reaches the end of the axon, voltage-gated Ca2+ channels open. 2)Ca2+ stimulates the fusing of synaptic vesicles to the plasma membrane and exocytosis of neurotransmitter. 3)A greater frequency of action potential results in more stimulation of the postsynaptic neuron. Ca2+ and Synaptic Vesicles 1)When Ca2+ enters the cell, it binds to a protein called synaptotagmin that serves as a Ca2+ sensor 2)Vesicles containing neurotransmitter are docked at the plasma membrane by three SNARE proteins. 3)The Ca2+ synaptotagmin complex stimulates vesicle fusion to the membrane 4)Forms a pore to release the NT Release of Neurotransmitter Neurotransmitter Axon 1. Action potentials diffuses across the Action terminal potentials reach axon terminals Ca2+ synapse, where it binds Action potentials to a specific receptor Sensor protein 2. Voltage-gated Ca2+ protein. + channels open Ca2+ Ca2+ 1-The Ca2+ Synaptic vesicles neurotransmitter is Ca2+– protein complex 3. Ca2+ binds to sensor referred to as the SNARE complex protein in cytoplasm ligand. Docking Ca2+ Ca2+ Fusion Synaptic This results in the cleft Exocytosis 4. Ca2+-protein complex opening of chemically stimulates fusion and exocytosis of regulated ion channels Neurotransmitter neurotransmitter (also called ligand- Postsynaptic cell released gated ion channels). Ca2+ Graded Potential 1)When ligand-gated ion channels open, the membrane potential changes depending on which ion channel is open. A. Opening Na+ or Ca2+ channels results in a graded depolarization called an excitatory postsynaptic potential (EPSP). B. Opening K+ or Cl− channels results in a graded hyperpolarization called inhibitory postsynaptic potential (IPSP). EPSPs and IPSPs A. EPSPs move the membrane potential closer to threshold; may require EPSPs from several neurons to actually produce an action potential B. IPSPs move the membrane potential farther from threshold C. IPSP Can counter EPSPs from other neurons so summation of EPSPs and IPSPs at the initial segment of the axon (next to the axon hillock) determines whether an action potential occurs. Summary of Neurotransmitter Action 1. Acetylcholine (ACh) ACh is a neurotransmitter that directly opens ion channels when it binds to its receptor. A. In some cases, ACh is excitatory, and in other cases it is inhibitory, depending on the organ involved B. Excitatory in some areas of the CNS, in some autonomic motor neurons, and in all somatic motor neurons C. Inhibitory in some autonomic motor neurons Two Types of Acetylcholine Receptors 1. Nicotinic ACh receptors A. Can be stimulated by nicotine B. Found on the motor end plate of skeletal muscle cells, in autonomic ganglia, and in some parts of the CNS 2. Muscarinic ACh receptors A. Can be stimulated by muscarine (from poisonous mushrooms) B. Found in CNS and plasma membrane of smooth and cardiac muscles and glands innervated by autonomic motor neurons Agonists and Antagonists 1) Agonists: drugs that can stimulate a receptor 1. Nicotine for nicotinic ACh receptors (so named because they can also be activated by nicotine) 2. Muscarine for muscarinic ACh receptors (so named because these effects can also be produced by muscarine (a drug derived from certain poisonous mushrooms). 2) Antagonists: drugs that inhibit a receptor. For example: a)Atropine is an antagonist for muscarinic receptors. b)Curare is an antagonist for nicotinic receptors. B. Chemically Regulated Channels The majority of synapses in the nervous system is one-way and occurs through the release of chemical neurotransmitters from presynaptic axon endings. These presynaptic endings, called terminal boutons Binding of a neurotransmitter to a receptor can open an ion channel in one of two ways: A. Ligand-gated channels B. G-protein coupled channels 1.Ligand-Gated Channels A. The receptor protein has extracellular sites that bind to the neurotransmitter ligands, while part of the protein spans the plasma membrane and has a central ion channel. B. Nicotinic ACh receptors are ligand-gated channels with two receptor sites for two AChs. C. Binding of 2 acetylcholine molecules opens a channel that allows both Na+ and K+ passage. 1) Na+ flows in, and K+ flows out. 2) Due to electrochemical gradient, more Na+ flows in than K+ out. D. This inward flow of Na+ depolarizes the cell, creating an EPSP. 1) EPSPs occur in the dendrites and cell bodies. 2) EPSPs from the binding of several ACh molecules can be added together to produce greater depolarization - graded 3) This may reach the threshold for voltage-gated channels in the axon hillock, leading to action potential. Nicotinic ACh Receptors 3.G-Protein Coupled Channels Another group of chemically regulated ion channels that, like the ligand-gated channels, are opened by the binding of a neurotransmitter to its receptor protein This group of channels differs from ligand-gated channels in that the receptors and the ion channels are different, separate membrane proteins, i.e binding of the neurotransmitter ligand to its receptor can open the ion channel only indirectly. Unlike the nicotinic receptors, these receptors do not contain ion channels 3.G-Protein Coupled Channels A. The neurotransmitter receptor is separate from the protein that serves as the ion channel. 1. Binding at the receptor opens ion channels indirectly by using a G-protein coupled channels. 2. Muscarinic ACh receptors interact with ion channels in this way as well as dopamine and norepinephrine receptors B. Associated with a G-protein 1. G-proteins have three subunits (alpha, beta, and gamma). 2. Binding of one acetylcholine results in the dissociation of the alpha subunit. 3. Either the alpha or the beta-gamma diffuses through the membrane to the ion channel. 4. This opens the channel for short period of time. 5. The G-protein subunits dissociate from the channel and it closes Activation of the G alpha subunit of a G-protein-coupled receptor In unstimulated cells, the state of G alpha (orange circles) is defined by its interaction with GDP, G beta-gamma (purple circles), and a G-protein-coupled receptor (GPCR; light green loops). Inactive Upon receptor stimulation by a ligand called an agonist, the state of the receptor changes. G alpha dissociates from the receptor and G beta-gamma, and GTP is exchanged for the bound GDP, which leads to G alpha activation. G alpha then goes on to activate other molecules in the cell. Nature Publishing Group Li, J. et al. The Molecule Pages database. Nature 420, 716-717 (2002). G-protein Coupled Channels G-protein couple channels, cont d.Binding of acetylcholine opens K+ channels in some tissues (IPSP) or closes K+ channels in others (EPSP). 1)In the heart, K+ channels are opened by the beta-gamma complex, creating IPSPs (hyperpolarization) that slow the heart rate. 2)In the smooth muscles of the stomach, K+ channels are closed by the alpha subunit, producing EPSPs (depolarization) and the contraction of these muscles. Action of Acetylcholinesterase (AChE) The bond between ACh and its receptor protein exists for only a brief instant. The ACh-receptor complex quickly dissociates but can be quickly re-formed as long as free ACh is in the vicinity. In order for activity in the postsynaptic cell to be stopped, free ACh must be inactivated very soon after it is released. The inactivation of ACh is achieved by means of an enzyme called acetylcholinesterase, or AChE, which is present on the postsynaptic membrane or immediately outside the membrane. AChE hydrolyzes acetylcholine into acetate and choline, which can then reenter the presynaptic axon terminals and be resynthesized into acetylcholine (ACh). Action of Acetylcholinesterase (AChE) Copyright © The McGraw-Hill Companies, Inc. Permission required for reproduction or display. Presynaptic axon Presynaptic axon Acetylcholine Acetate Choline Acetylcholinesterase Receptor Postsynaptic cell Postsynaptic cell To stop the activity AChE is an enzyme that inactivates ACh Hydrolyzes ACh into acetate and choline, in the postsynaptic activity shortly after it binds to the receptor. which are taken back into the presynaptic cell cell for reuse. 74