Body Systems & Tissues - Summary PDF
Document Details
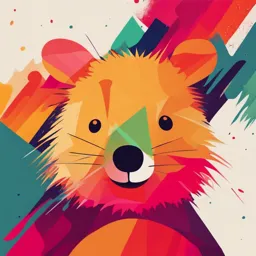
Uploaded by FragrantSpessartine
null
Tags
Summary
This document provides an overview of the human body's organization, specifically focusing on the skeletal system, including detailed information about bone structure, function, cells (osteoblasts, osteocytes, osteoclasts), and bone growth processes. It discusses concepts concerning bone growth and remodeling, the importance of growth hormone, and the role of bone marrow in the circulatory and immune systems.
Full Transcript
How the body is organised 11 body systems/ integration & scale / 4 tissue types 알아야 할 것들) distinguish between the 11 body systems, describe their major organ and function 1. Skeletal System: Bones in Humans: Humans typically have 206 bones in their body, though this can vary sli...
How the body is organised 11 body systems/ integration & scale / 4 tissue types 알아야 할 것들) distinguish between the 11 body systems, describe their major organ and function 1. Skeletal System: Bones in Humans: Humans typically have 206 bones in their body, though this can vary slightly in some cases due to extra bones (such as extra ribs or extra bones in the hands or feet). Ribs: Both male and female humans have 24 ribs, arranged in 12 sets, with 7 pairs attached to the sternum (true ribs), and 5 pairs that either float or connect indirectly (false ribs). Historical Note: The fact that humans have 24 ribs was confirmed through experimentation by the Flemish anatomist Andreas Vesalius in 1543, who studied human anatomy in great detail and corrected many misconceptions of the time. The two primary functions of bones in the human body are: 1. Support the Weight of the Body: Bones provide a rigid structure that supports the body’s weight and allows for the proper posture and positioning of body parts. 2. Withstand Forces of Contracting Muscles: Bones act as levers that muscles pull on to facilitate movement. The contractions of muscles apply forces to bones, which must be strong enough to withstand these forces during various activities such as walking, lifting, and jumping. Mature Bones Contain Three Cell Types: 1. Osteoblasts: These are bone-forming cells that secrete the bone matrix and are involved in the process of ossification (bone formation). 2. Osteocytes: These are mature bone cells that maintain the bone matrix. Osteocytes are embedded within the bone and communicate with each other through tiny channels (canaliculi). 3. Osteoclasts: These are large, multinucleated cells responsible for breaking down bone tissue. They are involved in bone resorption, which helps regulate calcium levels in the body and allows for the remodeling of bone tissue. 1. Osteoblasts Function: Osteoblasts are the bone-forming cells responsible for bone deposition throughout the life of an organism. They secrete the extracellular matrix (osteoid), which is primarily composed of collagen fibers and other proteins, providing a scaffold for mineral deposition. This matrix eventually mineralizes with calcium phosphate, specifically in the form of hydroxyapatite (Ca₁₀(PO₄)₆ (OH)₂), giving bone its rigidity and strength. Role in Bone Health: Osteoblasts are crucial in the continuous process of bone formation and remodeling, allowing bones to adapt to mechanical stress. 2. Osteocytes Function: Osteocytes are mature bone cells derived from osteoblasts. Once osteoblasts become surrounded by the calcified matrix they secreted, they differentiate into osteocytes. These cells are embedded within the bone matrix and are involved in maintaining the mineral content of the surrounding bone tissue. Osteocytes communicate with each other through tiny channels called canaliculi, allowing for the transfer of nutrients and signaling regarding mechanical stress. Role in Bone Health: Osteocytes help maintain bone homeostasis by responding to mechanical stimuli and relaying signals that can influence the activity of osteoblasts and osteoclasts. 3. Osteoclasts Function: Osteoclasts are large, multinucleated cells responsible for breaking down bone tissue during the process of bone resorption. They secrete acids (mainly hydrochloric acid) that dissolve hydroxyapatite, the mineral component of bone. Additionally, osteoclasts release enzymes such as collagenases to break down the protein component (osteoid) of the bone matrix. This resorption process is essential for the maintenance of calcium and phosphate levels in the blood and for bone remodeling. Role in Bone Health: Osteoclasts help in bone turnover, repairing microdamage, and reshaping bones. Their activity ensures that the bone matrix is constantly being remodeled and can adjust to changing stress and mechanical demands. Bone Remodeling Process Bone formation (deposition) by osteoblasts and bone resorption by osteoclasts are in a delicate balance. In younger individuals, these processes are well- matched, and bones remain strong. However, as people age (typically after the age of 30), the rate of bone resorption by osteoclasts begins to exceed the rate of bone deposition by osteoblasts. This imbalance leads to a decrease in bone mass and bone density, contributing to conditions like osteoporosis. Bone Repair When a bone breaks, osteoblasts and osteoclasts work together to repair it. Osteoblasts produce new bone material to fill in the fracture, while osteoclasts remove the old or damaged bone tissue. This collaborative process is essential for healing fractures and ensuring the bone regains its strength over time. Long bones grow during childhood primarily through a process involving chondrocytes, a type of cartilage cell. These cells play a critical role in the growth of bones, particularly in the epiphyseal plates (growth plates) at the ends of long bones. Here's an overview of the process: Bone Growth Process: 1. Chondrocytes Division and Enlargement: During childhood, chondrocytes (cartilage cells) divide and enlarge in response to growth hormone (GH). This division and enlargement help elongate the bone. 2. Calcification and Replacement: As the chondrocytes become trapped in the cartilage, they eventually undergo calcification, which means they harden due to the deposition of minerals. These calcified chondrocytes then die and are replaced by osteoblasts (bone-forming cells). This process gradually replaces the cartilage with bone tissue, contributing to the overall lengthening of the bone. 3. Role of Growth Hormone (GH): Growth hormone (GH) is essential for the division and enlargement of chondrocytes. It stimulates the growth of cartilage at the epiphyseal plates, promoting bone elongation. 4. Effect of Lack of GH - Achondroplasia: Achondroplasia is a condition caused by a deficiency or insensitivity to GH, leading to abnormal bone growth. In this case, the epiphyseal plates close prematurely or do not function properly, resulting in shorter limbs and a form of dwarfism. This occurs because the chondrocytes cannot effectively divide or enlarge in response to GH. Epiphyseal Plate Closure: In children, the epiphyseal plates are open and active, allowing for continued elongation of the bones. In late adolescence, the epiphyseal plates gradually close as the bones mature, and bone growth stops once they are fully replaced by bone tissue, marking the end of long bone elongation. Once the epiphyseal plates are closed, bones no longer elongate, and their growth is limited to changes in bone thickness and remodeling. GH Treatment in Children vs. Adults: In children: GH treatment can be effective in stimulating bone growth because their epiphyseal plates are still open. This can lead to increased bone lengthening and help treat growth deficiencies. In adults: Once the epiphyseal plates have closed after puberty, GH treatment will no longer have an effect on bone elongation, as the plates have already fused and no longer allow for the bones to grow longer. In summary, growth hormone stimulates chondrocytes to divide and enlarge, allowing for the elongation of long bones. In the case of achondroplasia, a lack of GH or its proper function leads to impaired bone growth. GH treatment can help children with growth deficiencies, but it is not effective after the epiphyseal plates close in adulthood. Bone marrow is a vital organ involved in both hematopoiesis (the production of blood cells) and the formation of cells for the immune system. It contains stem cells that give rise to various types of blood cells, including red blood cells, white blood cells, and platelets. The overlap between these two systems (circulatory and immune) is significant because they are both crucial for maintaining homeostasis and responding to bodily needs, including oxygen transport and immune defense. Key Points on Bone Marrow's Role: 1. Stem Cells in Bone Marrow: o The bone marrow contains hematopoietic stem cells (HSCs), which are pluripotent cells capable of differentiating into all blood cell types. o HSCs can give rise to two main lineages: ▪ Myeloid lineage (producing red blood cells, platelets, and some types of white blood cells). ▪ Lymphoid lineage (producing various immune cells such as T cells, B cells, and natural killer cells). 2. Overlap Between Circulatory and Immune Systems: o The circulatory system relies on red blood cells (RBCs), which are produced in the bone marrow, to transport oxygen to tissues and remove carbon dioxide from the body. o The immune system relies on white blood cells (WBCs), which are also produced in the bone marrow. These include: ▪ Neutrophils and monocytes, which are involved in innate immunity (the body’s first line of defense against pathogens). ▪ Lymphocytes (T cells, B cells, and NK cells), which are central to adaptive immunity, identifying and attacking specific pathogens or infected cells. 3. Example of System Overlap: o Lymphocyte Production: Lymphoid progenitor cells in the bone marrow differentiate into T cells, B cells, and natural killer (NK) cells. While the bone marrow generates these immune cells, some of them (like T cells) migrate to other organs (like the thymus) for further maturation. ▪ T cells play a critical role in adaptive immunity, targeting specific pathogens. ▪ B cells produce antibodies that are crucial for immune response. o Additionally, neutrophils and monocytes (from the myeloid lineage) help with both innate immune responses and with inflammation, making them part of the body’s initial defense system and contributing to the healing of wounds and fighting infections. Example of Overlap in Function: When the body experiences an infection, the immune system responds by deploying white blood cells (WBCs) from the bone marrow into the bloodstream to fight the infection. Simultaneously, the red blood cells (RBCs) in the circulation continue to transport oxygen, enabling the immune cells to perform their function more effectively. Infection or blood loss can trigger an increase in production of both red blood cells (to restore oxygen-carrying capacity) and white blood cells (to fight infection), demonstrating a functional overlap between the immune system and the circulatory system through the action of the bone marrow. In summary, bone marrow is a critical organ that bridges the circulatory and immune systems by producing both the blood cells responsible for oxygen transport and the immune cells that defend against infection. This overlap ensures that the body can respond to infections while maintaining adequate oxygen supply. 2. Muscular System: 1. Skeletal Muscle Function: Skeletal muscles are primarily responsible for moving the skeleton, allowing voluntary movements like walking, running, and lifting objects. They are also involved in facial expressions, posture maintenance, and breathing (through diaphragm contractions). Structure: Skeletal muscles are long, multinucleated fibers with a striated (striped) appearance due to the regular arrangement of actin and myosin filaments. These muscles are controlled voluntarily by the somatic nervous system. Exceptions: While most skeletal muscles move the skeleton, there are three exceptions: 1. Diaphragm: Although it is a skeletal muscle, it functions involuntarily in breathing. 2. Muscles of the middle ear: These muscles are skeletal but have a reflexive, involuntary function related to hearing. 3. Sphincter muscles: These can be skeletal and are involved in controlling the opening and closing of various body openings (like the anal sphincter) and may function under voluntary or involuntary control. 2. Cardiac Muscle Function: Cardiac muscle is responsible for the contraction of the heart, enabling the pumping of blood throughout the body. It is specialized for rhythmic, involuntary contraction and relaxation, which is essential for maintaining circulation. Structure: Like skeletal muscle, cardiac muscle has striations, but its cells are short, branched, and interconnected, allowing the heart to contract as a single unit. The cells are connected by intercalated discs, which allow for the rapid transmission of electrical impulses between cells, ensuring synchronized contractions. Control: Cardiac muscle is controlled involuntarily by the autonomic nervous system and is influenced by electrical impulses generated by the sinoatrial (SA) node, the heart's natural pacemaker. 3. Smooth Muscle Function: Smooth muscle allows for the contraction and relaxation of the walls of various hollow organs and structures in the body, including blood vessels, the digestive system, the bladder, and the reproductive organs. It plays a vital role in controlling the flow of blood through vessels and the movement of food through the digestive tract. Structure: Smooth muscle cells are non-striated, spindle-shaped cells with a single central nucleus. The lack of striations is due to a more irregular arrangement of actin and myosin filaments compared to skeletal muscle. Control: Smooth muscle is controlled involuntarily by the autonomic nervous system and can also be regulated by hormones and local chemical signals. Smooth muscle contractions are slower and more sustained than skeletal muscle contractions. Overlap Between Systems There is functional overlap between these muscle types in certain physiological systems: o Vascular Smooth Muscle & Cardiac Muscle: The contraction and relaxation of smooth muscle in blood vessels help regulate blood flow and blood pressure. Similarly, cardiac muscle contracts to pump blood. Both are critical for the cardiovascular system. o Smooth Muscle in the Digestive System & Skeletal Muscle: The digestive system involves both smooth muscle (for peristalsis and mixing) and skeletal muscle (for voluntary control of swallowing and defecation). These muscles work together to move food through the digestive tract and regulate the processes. o Skeletal Muscle & Cardiac Muscle: While skeletal muscles control voluntary movement, they work with the heart muscle (cardiac muscle) during activities like exercise, where muscle activity increases heart rate and blood flow. Galvani’s Experiment Setup: Luigi Galvani, an Italian physician and scientist, used a frog’s leg for his experiments. He suspended the leg on a metal wire and connected it to a copper wire, essentially creating a simple circuit. Observation: During a thunderstorm, Galvani noticed that the frog's leg contracted, even though the frog was already dead. He hypothesized that the electrical energy from the storm somehow triggered the muscle contractions. Interpretation: Galvani believed that muscles contained an inherent "animal electricity" and that this electricity was responsible for muscle contractions. He speculated that the electricity from the storm, possibly acting on the metal wires, had somehow triggered the contraction of the frog's muscles. Significance of Galvani's Work Animal Electricity: Galvani's work led him to propose the concept of "animal electricity," which he believed was a vital force that existed within living organisms and was responsible for muscle movement. While the term "animal electricity" was later replaced by the more modern understanding of bioelectricity, Galvani's experiments helped lay the foundation for the study of electrical signals in biological systems. Further Investigation: Although Galvani believed that the electrical stimulus originated from within the frog’s body, his work inspired other scientists, including Alessandro Volta, who refuted Galvani’s idea. Volta demonstrated that the electrical effects were due to the contact between the metal wires and the frog’s tissue, leading to the discovery of voltaic electricity (the precursor to the battery). This laid the groundwork for the development of the modern field of electrochemistry. Understanding of Nerve and Muscle Function: Galvani’s experiment was pivotal in the development of modern electrophysiology, as it demonstrated that muscles could respond to electrical stimuli. This would later contribute to the understanding that the nervous system and muscles communicate via electrical impulses. Galvani vs. Volta Galvani’s Hypothesis: He believed that electricity was generated by the animal tissues themselves and was a property of living organisms. Volta’s Counter-Argument: Volta proposed that the electrical effects were due to the materials (metals) in contact with the frog's tissues, not a special electrical force inherent in the frog’s body. Modern Relevance Electrophysiology: Today, we understand that muscle contractions are triggered by electrical impulses that originate from the nervous system. Neurons transmit electrical signals to muscles, initiating the release of calcium ions, which in turn cause the muscle fibers to contract. This phenomenon is governed by the principles of bioelectricity and ion movement, with ions like sodium (Na⁺), potassium (K⁺), calcium (Ca²⁺), and chloride (Cl⁻) playing crucial roles. Technological Applications: Galvani's work also paved the way for innovations in medical technology, including electromyography (EMG), which measures electrical activity in muscles, and electrical stimulation therapies that use electrical currents to treat muscle dysfunction. Summary Galvani’s experiment was a significant milestone in the study of bioelectricity. While his hypothesis about "animal electricity" was eventually replaced with more accurate models, his work remains a cornerstone in the understanding of how electrical signals control muscle function and how the nervous system and muscles interact. 3. Nervous system: Nerve cells, also known as neurons, are integral to the functioning of the nervous system, facilitating communication between the brain and other parts of the body. Here's a deeper look into how they work, and the significance of neurotoxins: Nerve Cells (Neurons) Structure of Neurons: o Dendrites: These receive electrical signals from other neurons. o Axon: This long projection transmits electrical impulses away from the cell body to other neurons or muscles. o Synapse: The junction between two neurons where communication occurs via neurotransmitters. At the synapse, electrical signals are converted to chemical signals to propagate the signal to the next neuron. Neurotransmitters: These are chemical messengers that transmit signals across synapses between neurons. Examples include dopamine, serotonin, and acetylcholine. Electrical Impulses: Neurons communicate through action potentials—electrical impulses that travel along the axon. These impulses are generated when ion channels in the neuron's membrane open, allowing ions like sodium (Na⁺) and potassium (K⁺) to flow in and out of the cell. The Brain The human brain consists of approximately 10^11 neurons (100 billion), making it the most complex organ in the body. Connections: Neurons in the brain form approximately 10^14 synapses—an extraordinarily high number that allows for complex processing, memory storage, and higher cognitive functions. These synapses and the neural networks they create are responsible for everything from basic motor functions to complex thinking and reasoning. Nerve Functions Motor Control: Nerve cells control muscle contractions, allowing for voluntary and involuntary movements. Electrical impulses from the brain travel via the spinal cord and peripheral nerves to stimulate muscles to contract or relax. Hormone Secretion: Neurons also play a role in regulating the endocrine system by controlling the release of hormones from glands (e.g., the hypothalamus controlling the pituitary gland). Sensory Input: Nerves transmit sensory information from the body to the brain, including sensations like touch, temperature, and pain, allowing the brain to respond to stimuli. Neurotoxins and Their Effects Neurotoxins are chemicals that interfere with the function of neurons, either by blocking nerve transmission or damaging nerve cells directly. o Therapeutic Uses: Some neurotoxins are used in medicine for therapeutic purposes. For example: ▪ Botulinum toxin (Botox) can block acetylcholine release, causing temporary muscle paralysis. It’s used in medicine to treat muscle spasms, reduce wrinkles, and manage excessive sweating. ▪ Local anesthetics like lidocaine block nerve conduction, numbing specific regions of the body during surgeries or dental procedures. o Toxic or Fatal Consequences: On the other hand, neurotoxins can have fatal consequences if exposure is uncontrolled: ▪ Tetrodotoxin (found in pufferfish) blocks sodium channels, paralyzing muscles, and can lead to death due to respiratory failure. ▪ Sarin gas (a nerve agent) blocks acetylcholinesterase, preventing neurotransmitter breakdown, causing overstimulation of muscles and potentially leading to fatal seizures. Blocking Nerve Function Neurotoxins can block nerve function by: 1. Interfering with neurotransmitter release (e.g., botulinum toxin). 2. Blocking neurotransmitter receptors (e.g., curare). 3. Inhibiting ion channels (e.g., tetrodotoxin, which blocks sodium channels). Summary Neurons are essential for communication between the brain and the rest of the body, enabling movement, sensory perception, and hormonal regulation. The brain's 100 billion neurons form vast networks through trillions of synapses, making it capable of complex functions. Neurotoxins can alter nerve function, offering therapeutic benefits when used carefully, but they can also have deadly effects if misused. Understanding the balance between these effects is crucial in both medical treatments and the study of nerve function. 4. Digestive system: The Gastrointestinal Tract (GIT) plays a crucial role in digestion, absorption, and excretion of materials, and it works in conjunction with various systems in the body, such as the muscular, respiratory, and renal systems. Structure and Function of the GIT: Length: The GIT is approximately 4.5 meters long, starting from the mouth and extending to the rectum. Function: o Ingestion: Water, food, and other materials enter the GIT. o Absorption: Useful substances like nutrients, water, and salts are absorbed into the bloodstream. o Excretion: Waste gases are exhaled through the lungs, while waste water is filtered out by the kidneys. The unabsorbed material, which includes fiber and undigested food, is expelled as feces. Mechanisms of Absorption and Secretion: The absorption and secretion of materials in the GIT are critical processes involving various mechanisms: o Absorption involves the transfer of nutrients and other molecules from the lumen of the GIT into the blood or lymph. o Secretion involves the release of digestive enzymes, acids, and bicarbonates to aid digestion or neutralize stomach acid. Integration with Other Systems: The GIT is not just a standalone system but interacts with various other body systems: 1. Muscular System: ▪ Skeletal muscles (such as the tongue and anal sphincter) assist in controlling the movement of food through the digestive tract. The tongue helps in the chewing process and the swallowing of food, while the anal sphincter controls the expulsion of feces. ▪ These muscles are not directly connected to bones but are critical for GIT function. 2. Respiratory System: ▪ Waste gas, primarily in the form of carbon dioxide, is exhaled through the lungs, which helps in maintaining pH balance and removing metabolic waste. 3. Renal System: ▪ The kidneys filter out excess water and waste products from the blood, helping maintain fluid balance and electrolyte levels, which in turn supports the digestive processes. Key Points of Integration: Tongue: Involved in mechanical digestion and swallowing; it works with the muscular system to ensure food is moved into the esophagus. Anal Sphincter: A skeletal muscle that controls defecation, ensuring the body only expels waste when appropriate. Conclusion: The GIT is an essential system for breaking down food, absorbing nutrients, and eliminating waste. Its functionality is supported by muscles and closely integrated with other systems like the respiratory and renal systems to maintain homeostasis. Understanding this integration is key to comprehending how the body processes and excretes substances efficiently. 5. Urinary system: Definition of "Excess" Water and Kidney Regulation The kidneys are key organs in regulating the body’s water balance by filtering the blood and excreting waste products and excess water as urine. The "excess" of water refers to the amount of water in the body that exceeds the optimal balance or the body's needs for maintaining homeostasis. If the blood's water volume increases beyond normal levels, the kidneys work to restore balance by excreting the excess through urine. This process is regulated by the kidneys through various mechanisms that adjust the amount of water and concentration of solutes in the blood. These mechanisms help maintain the homeostasis of water and electrolytes in the body. How Water Leaves the Blood and Becomes Urine The process of urine formation involves several key stages in the kidneys that control how much water and other substances are retained or excreted. Here's how water leaves the blood and becomes urine: 1. Filtration (Renal Corpuscle) The blood enters the kidney through the renal artery and flows into a structure called the glomerulus in the renal corpuscle (located in the nephron). The glomerulus acts as a filter, allowing water, small solutes (like salts, glucose, and waste products), and waste materials to pass through into the Bowman's capsule (the initial part of the nephron). Larger molecules, such as proteins and blood cells, are too large to pass through the filtration barrier and remain in the bloodstream. 2. Reabsorption (Proximal Convoluted Tubule, Loop of Henle, Distal Convoluted Tubule) Once the filtrate enters the nephron, the body selectively reabsorbs water and essential solutes back into the bloodstream, depending on the body's needs. The proximal convoluted tubule reabsorbs a significant amount of water, as well as glucose, amino acids, and ions. In the Loop of Henle, water and solutes are reabsorbed in a countercurrent mechanism, where the descending limb is permeable to water (leading to water reabsorption), and the ascending limb is impermeable to water but actively reabsorbs sodium and chloride. The distal convoluted tubule and collecting ducts further regulate water and electrolyte balance, depending on hormonal signals (like antidiuretic hormone (ADH)). 3. Secretion (Distal Convoluted Tubule and Collecting Ducts) The kidneys also secrete excess waste products and ions into the tubules, which are then carried through the nephron toward the collecting ducts. Potassium (K+), hydrogen ions (H+), and certain drugs are secreted into the tubular fluid, which is then eventually excreted as part of urine. 4. Water Retention and Excretion (Effect of Antidiuretic Hormone) The kidneys regulate the amount of water excreted in response to hydration status and the body's needs. When the body is hydrated, less water is reabsorbed in the kidneys, and more urine is produced. When the body is dehydrated or if there is an excess of solutes, the hypothalamus releases antidiuretic hormone (ADH), which signals the kidneys to retain water by increasing the permeability of the collecting ducts to water. This allows more water to be reabsorbed into the bloodstream, reducing the amount of water excreted in urine. When there is an excess of water in the body, the secretion of ADH decreases, leading to less water reabsorption, and more water is excreted in the urine, leading to dilute urine. 5. Excretion as Urine The final filtrate, which now contains excess water and waste products, flows into the collecting ducts and is transported to the renal pelvis, then into the ureter, and eventually to the bladder for storage until it is excreted from the body via the urethra. Summary of Mechanisms Involved in Water Excretion by Kidneys: 1. Filtration in the glomerulus to remove water and solutes from blood. 2. Reabsorption of water (and important solutes) in the proximal tubule, loop of Henle, and distal convoluted tubule. 3. Secretion of waste products and excess ions into the nephron. 4. Regulation by ADH, adjusting the permeability of the collecting ducts to water, depending on hydration status. 5. Excretion of excess water in urine when needed. Kidney's Role in Maintaining Water Balance: The kidneys play a critical role in maintaining fluid homeostasis by adjusting the amount of water reabsorbed or excreted, based on the body's hydration needs. Excess water is excreted in response to high blood volume or low solute concentration (hypotonic conditions), while water retention occurs when the body needs to conserve water to maintain blood pressure and hydration levels. In conclusion, kidneys regulate the total amount of water in the blood by balancing the amount of water reabsorbed and excreted as urine. When the body is in excess of water, the kidneys will excrete the extra fluid to maintain homeostasis. 6. Respiratory system: Oxygen and Its Role in ATP Production: Oxygen (O₂) is essential for oxidative phosphorylation, the primary process in the body for generating ATP (adenosine triphosphate), which is used as the energy currency of cells. This process occurs in the mitochondria and relies on the presence of oxygen to effectively produce ATP through the electron transport chain and chemiosmosis. Role of the Diaphragm in Gas Exchange: The diaphragm is a critical muscle that plays a central role in respiration and gas exchange: o Diaphragm Contraction: When the diaphragm contracts, it moves downward, increasing the volume of the thoracic cavity, which leads to a decrease in lung pressure. This causes air to flow into the lungs (inhalation), allowing oxygen to be absorbed into the bloodstream. o Diaphragm Relaxation: When the diaphragm relaxes, it moves upward, decreasing the thoracic cavity volume and increasing lung pressure. This forces air out of the lungs (exhalation), expelling carbon dioxide, a waste product of cellular respiration. o The diaphragm essentially drives the mechanical process of ventilation, which facilitates the exchange of gases (oxygen and carbon dioxide) between the lungs and the blood. Skeletal Muscles and the Diaphragm: Although skeletal muscles (such as those in the diaphragm) are involved in breathing, they do not directly attach to bones in the same way as other skeletal muscles that control voluntary movement. The diaphragm is a muscle that is important for the respiratory system, facilitating the movement of gases into and out of the lungs. Integration Between Systems: There is significant overlap between systems in maintaining overall body function, particularly in energy production, respiration, and muscle activity: 1. Respiratory and Muscular Systems: The diaphragm, a skeletal muscle, is involved in respiration (breathing). The contraction and relaxation of this muscle drive gas exchange in the lungs, which is essential for supplying oxygen to tissues for ATP production through oxidative phosphorylation. 2. Cardiovascular and Muscular Systems: Oxygen transported by the blood via the cardiovascular system is utilized by skeletal muscles, allowing them to perform work (movement and contraction). 3. Muscular System and Energy Production: Skeletal muscles rely on ATP for contraction. ATP is produced primarily through aerobic respiration (oxidative phosphorylation), which depends on the supply of oxygen to tissues. Conclusion: The diaphragm serves as a key player in gas exchange, driving the process of inhalation and exhalation, which is vital for providing oxygen to the body. This oxygen is then used in the process of oxidative phosphorylation to generate ATP, powering various physiological functions, including muscle contraction. This illustrates the integration between the muscular, respiratory, and cardiovascular systems to maintain the energy needs of the body. & ATP Production and Oxygen Delivery: ATP production is essential for the function of virtually every cell in the body, and this process primarily occurs through oxidative phosphorylation in the mitochondria of cells. This pathway requires oxygen (O₂) to efficiently produce ATP, which is the main energy source for cellular processes. Glucose for ATP Production: Glucose is a key substrate for ATP production, especially in the process of glycolysis (the first step of glucose metabolism). While glycolysis can occur without oxygen (anaerobic conditions), the full breakdown of glucose to yield ATP occurs in the presence of oxygen through aerobic respiration, which involves the Krebs cycle and oxidative phosphorylation. Carbon Dioxide (CO2) as a Waste Product: As cells generate ATP through metabolic processes, they also produce carbon dioxide (CO₂) as a waste product, particularly from the Krebs cycle during aerobic respiration. Need for Gas Exchange: Oxygen (O₂) must be delivered to cells to support ATP production, and carbon dioxide (CO₂), as a waste product, must be removed from cells to maintain homeostasis and prevent acidosis (a dangerous build-up of CO₂ in the blood). Therefore, there is a constant need for gas exchange between the lungs (where oxygen is absorbed and CO₂ is released) and the bloodstream (where oxygen is transported to tissues and CO₂ is carried away to be expelled through the lungs). This exchange occurs via the respiratory system, with the diaphragm playing a central role in driving ventilation (breathing). Integration of Systems for Gas Transport and Waste Removal: 1. Circulatory System: Oxygen is transported by the blood, bound to hemoglobin in red blood cells, from the lungs to tissues. Glucose is delivered via the blood to cells. 2. Respiratory System: Oxygen is absorbed into the bloodstream in the lungs, and carbon dioxide is expelled. The diaphragm and other respiratory muscles control the process of breathing. 3. Excretory System: Carbon dioxide is expelled via the lungs, while other waste products are filtered and excreted by the kidneys. Conclusion: For ATP production to occur efficiently, oxygen must reach cells, and glucose is required for energy production. The cells also produce waste products like carbon dioxide, necessitating the removal of these waste products to maintain a healthy internal environment. The respiratory system, cardiovascular system, and excretory system work together to ensure that oxygen is delivered to cells, waste products are removed, and homeostasis is maintained. 7. Circulatory system: Cardiovascular System and Blood Circulation: 1. Heart Function: o The heart is responsible for pumping blood throughout the body, beating about 3 billion times in a lifetime. o Each beat pumps around 70 mL of blood, which contains approximately 300 billion red blood cells (erythrocytes). o The heart ensures the continuous circulation of blood, which transports oxygen (O₂), nutrients, and waste products (like CO₂) to and from tissues. 2. Blood Composition and Transport: o Erythrocytes (Red Blood Cells): These cells are crucial for transporting oxygen. They contain hemoglobin, which binds to oxygen in the lungs and releases it in tissues that need it. o Plasma: The plasma of blood transports nutrients (such as glucose, amino acids, and lipids), waste products (like CO₂ and urea), and hormones throughout the body. o CO₂ Transport: After tissues produce carbon dioxide (a waste product), deoxygenated blood is carried back to the heart, then pumped to the lungs for CO₂ removal during exhalation. 3. Oxygenation and Deoxygenation Process: o Deoxygenated blood: This blood enters the heart and is pumped to the lungs for oxygenation. o Oxygenated blood: After oxygen is absorbed in the lungs, the oxygenated blood returns to the heart and is then pumped throughout the body to supply tissues with oxygen and nutrients. 4. Circulatory Frequency: o The heart beats approximately 72 times per minute on average, ensuring that oxygenated blood is continuously circulated to meet the body's metabolic needs. Integration with Endocrine System: 1. Hormone Transport via Bloodstream: o Endocrine glands secrete hormones (such as insulin, adrenaline, and thyroid hormones) directly into the bloodstream. o These hormones are transported to various organs and tissues throughout the body, helping regulate vital functions like metabolism, growth, and response to stress. o Though the transport of hormones via the bloodstream is slower compared to nerve impulses, it is highly effective, allowing hormones to reach distant organs and tissues, influencing long-term processes. 2. Coordination Between Systems: o The circulatory system and endocrine system work together to maintain homeostasis. Hormones regulate processes like growth, immune response, and metabolism, while the circulatory system ensures these signals are delivered to the appropriate locations. o For example, the hormone insulin is released by the pancreas and transported through the blood to regulate glucose levels in the body, while adrenaline released during stress helps prepare the body for a "fight or flight" response by increasing heart rate and blood flow. Key Takeaways: The heart pumps blood around the body, circulating oxygen to cells and removing waste like CO₂. Red blood cells are essential for transporting oxygen, and the plasma carries nutrients and waste. The circulatory system works in conjunction with the endocrine system, allowing hormones to be transported quickly to various organs to regulate bodily functions. This integration between systems is essential for the body's overall function and homeostasis. 8. Endocrine system: Examples of Hormonal Effects in the Body: 1. Adrenaline (Epinephrine): o Function: Released during stressful situations (e.g., "fight or flight" response), adrenaline prepares the body for immediate physical exertion. o Effects: ▪ Increases heart rate to pump more oxygenated blood to muscles. ▪ Constricts blood vessels in certain areas (e.g., skin, digestive system) to redirect blood flow to muscles and vital organs. ▪ Dilates airways to increase oxygen intake. ▪ Boosts glucose release to provide quick energy. o Integration with Circulatory System: Adrenaline travels through the bloodstream, reaching the heart, blood vessels, and muscles to facilitate the body’s quick response to stress. 2. Melatonin: o Function: Regulates the body’s biorhythms, specifically the sleep-wake cycle. o Effects: ▪ Released by the pineal gland in response to darkness. ▪ Promotes feelings of sleepiness and helps synchronize the body’s internal clock with the external environment (day-night cycle). o Integration with Circulatory System: Melatonin is transported through the blood to the brain and various tissues to regulate sleep patterns. It influences the hypothalamus and other parts of the brain involved in controlling circadian rhythms. 3. Insulin: o Function: Regulates blood glucose levels by promoting the uptake of glucose into cells. o Effects: ▪ Released by the pancreas in response to elevated blood sugar levels after eating. ▪ Helps cells absorb glucose for energy or storage, lowering blood sugar. ▪ Stimulates liver and muscles to store glucose as glycogen. o Integration with Circulatory System: Insulin travels through the blood to tissues and organs, where it facilitates glucose uptake and regulation of blood sugar levels. It is essential for energy balance and metabolism. Integration of Circulatory & Endocrine Systems: Hormones are chemical messengers that are secreted into the blood by various glands, such as the adrenal glands, pineal gland, and pancreas. Once in the bloodstream, hormones can reach virtually any part of the body, where they bind to specific receptors on target cells, triggering various physiological responses. o Adrenaline is rapidly circulated to prepare the body for an emergency response. o Melatonin influences the brain’s regulation of sleep cycles, impacting overall health and circadian rhythms. o Insulin controls glucose metabolism and energy distribution by interacting with tissues and organs. Circulatory System: Delivers hormones to their target sites efficiently but more slowly than nerve impulses. This slower, sustained action is ideal for regulating long-term processes like metabolism and growth. Key Points: The endocrine system uses hormones to communicate with distant organs and regulate vital functions, while the circulatory system transports these hormones. Hormonal signals, though slower than nerve impulses, are crucial for the long- term regulation of processes like metabolism, stress response, and sleep. Integration of these systems allows the body to respond quickly to short-term demands (e.g., adrenaline for stress) and manage long-term processes (e.g., insulin for glucose control and melatonin for sleep regulation). 9. immune system: Discrimination Between Self and Non-Self The immune system's ability to distinguish between self (the body’s own cells) and non- self (foreign substances or pathogens) is essential for protecting the body from infections, cancer, and other diseases while preventing damage to its own tissues. 1. Self: This refers to the body’s own cells and molecules, which are recognized as normal and not harmful. 2. Non-Self: These include foreign pathogens, such as viruses, bacteria, fungi, and parasites, as well as abnormal or cancerous cells. The immune system has a sophisticated mechanism to recognize and respond to non-self elements through antigens, molecules that are recognized by the immune system as foreign or dangerous. Innate Immune Responses The innate immune system is the body’s first line of defense against infections and works quickly to respond to pathogens. It does not require prior exposure to a pathogen to mount a response. 1. Physical Barriers: Skin and mucous membranes act as barriers to prevent pathogens from entering the body. 2. Phagocytosis: Immune cells like macrophages and neutrophils can engulf and destroy pathogens through a process called phagocytosis. 3. Pattern Recognition Receptors (PRRs): These receptors on immune cells recognize pathogen-associated molecular patterns (PAMPs), which are common structures found on many pathogens, enabling quick recognition and response. 4. Inflammatory Response: The innate immune system triggers inflammation in response to infection or injury to help recruit immune cells to the site of infection or damage. 5. Complement System: A group of proteins in the blood that work together to destroy pathogens or mark them for destruction by phagocytes. Adaptive Immunity The adaptive immune system is a more specific and slower response compared to the innate system, but it provides long-term immunity. It develops after exposure to a pathogen and is capable of "remembering" the pathogen, allowing for a faster response upon subsequent exposures. 1. Humoral Immunity (Antibody-Mediated): o B lymphocytes (B cells) are responsible for producing antibodies that target specific antigens on pathogens. o Antibodies bind to foreign antigens, neutralizing pathogens or marking them for destruction by other immune cells. 2. Cell-Mediated Immunity: o T lymphocytes (T cells) help recognize and destroy infected or abnormal cells, such as virus-infected cells or cancer cells. o Helper T cells (CD4+) coordinate the immune response by activating other immune cells. o Cytotoxic T cells (CD8+) kill infected or cancerous cells directly. 3. Memory Cells: o After an infection or vaccination, the immune system produces memory B cells and memory T cells that "remember" the pathogen, allowing the immune system to respond more rapidly and effectively during future exposures. Inflammatory Response The inflammatory response is a key component of the innate immune system that helps to protect the body by promoting healing and limiting infection. It involves a series of steps that are triggered when the body detects infection or injury: 1. Vasodilation: Blood vessels expand to allow more blood flow to the affected area, leading to redness and warmth. 2. Increased Permeability: Blood vessels become more permeable, allowing immune cells (e.g., neutrophils, macrophages) and proteins (e.g., antibodies, complement proteins) to leave the bloodstream and enter tissues. 3. Recruitment of Immune Cells: White blood cells are attracted to the site of infection or injury through signaling molecules called chemokines. 4. Phagocytosis: Immune cells, particularly macrophages and neutrophils, engulf and digest pathogens or dead cells. 5. Resolution: The inflammatory response is resolved once the infection or injury is cleared, and the body returns to a normal state. Summary: Discrimination between self and non-self is fundamental to immune function, and the immune system uses both innate and adaptive responses to protect the body from harmful invaders while preventing damage to its own tissues. Innate immunity provides an immediate, nonspecific defense against pathogens, while adaptive immunity is slower but more specific and provides lasting protection. The inflammatory response helps to contain and eliminate pathogens while promoting healing and tissue repair. 10. Integumentary system: Integumentary System The integumentary system is the largest organ system in animals, consisting of the skin, hair, nails, feathers, scales, skin glands, and their secretions. This system serves as a barrier to the environment and performs several critical functions to protect the body. Components of the Integumentary System 1. Skin: The skin is the body's outer covering, made up of two main layers: o Epidermis: The outermost layer, primarily composed of keratinocytes, which produce keratin, a protein that helps protect the skin. o Dermis: The layer beneath the epidermis that contains blood vessels, nerve endings, hair follicles, and sweat glands. 2. Hair: Hair serves various functions, including thermal insulation, protection from UV radiation, and sensory functions. Hair follicles are located in the dermis and are composed of keratin. 3. Nails: Nails protect the tips of fingers and toes and enhance the sense of touch. They are made of hardened keratin. 4. Feathers and Scales: These are modified forms of the skin found in birds, reptiles, and some mammals. They provide protection, assist with thermoregulation, and play a role in mating displays and camouflage. 5. Skin Glands: These include: o Sweat glands: These glands secrete sweat, which helps regulate body temperature. o Sebaceous glands: These glands secrete oils (sebum) that lubricate the skin and hair. o Mucus glands: Found in mucous membranes, they secrete mucus, which helps protect the body from pathogens and helps in lubrication. Functions of the Integumentary System 1. Protection: The skin acts as a physical barrier, protecting the body from physical damage, harmful chemicals, pathogens, and dehydration. 2. Sensory Perception: The skin contains sensory receptors that allow organisms to sense changes in temperature, pressure, pain, and touch, providing vital information about the environment. 3. Temperature Regulation: Sweat production, blood vessel dilation or constriction, and the insulating properties of hair or fur help regulate body temperature. Sweat evaporates from the skin’s surface, cooling the body. 4. Immune Defense: The skin plays a role in the innate immune system. It acts as a physical and chemical barrier against pathogens. For example: o Sweat has a low pH (around 4), which inhibits the growth of many bacteria and fungi, preventing their colonization. o The skin also produces antimicrobial peptides that destroy pathogens. 5. Excretion: The skin excretes waste products such as urea, salts, and water through sweat glands. 6. Synthesis of Vitamin D: The skin plays a critical role in the synthesis of vitamin D when exposed to sunlight. Vitamin D is necessary for calcium absorption in the intestines and bone health. 7. Coloration: The color of the skin, hair, and eyes is primarily determined by the level of melanin, a pigment produced by melanocytes in the epidermis. The amount and type of melanin determine the shades of skin, hair, and eye color. Melanin also provides protection from harmful UV radiation. Role in the Immune System The innate immune system involves immediate, non-specific defense mechanisms, and the skin is a key part of this response. The skin’s acidic pH (due to sweat and sebaceous gland secretions) helps inhibit bacterial growth and prevent infections. The skin's physical barrier prevents pathogens from entering the body. The immune cells in the skin, such as Langerhans cells, detect and respond to pathogens that manage to breach the barrier. Summary The integumentary system is vital for protecting the body, providing sensory input, and maintaining homeostasis. The skin, hair, nails, and glands work together to shield the body from environmental threats, regulate temperature, excrete waste, and contribute to the immune system. The production of melanin in the skin also helps in protecting against UV radiation and contributes to the coloration of the skin. 11. Reproductive systems: Interface of Physiology and Genetics in Reproduction The process of reproduction is fundamental to the continuation of species, and it involves the fusion of egg (female gamete) and sperm (male gamete) to give rise to offspring. This fusion is called fertilization, and it marks the beginning of the development of a new organism. The interface between physiology (the functioning of the body systems) and genetics (the inheritance of traits) is crucial for understanding how offspring inherit traits and how physiological processes contribute to successful reproduction. How Egg and Sperm Give Rise to Offspring 1. Gamete Formation (Meiosis): o Oogenesis (in females) and Spermatogenesis (in males) are the processes by which the egg and sperm are formed. o Both processes involve meiosis, a type of cell division that reduces the chromosome number by half, producing haploid cells (eggs and sperm), each with a single set of chromosomes (23 in humans). o In oogenesis, the egg is developed in the ovaries, and only one functional egg is produced from each precursor cell. o In spermatogenesis, sperm are produced in the testes, and each precursor cell produces four viable sperm. 2. Fertilization: o When sperm reaches the egg (usually in the fallopian tube in humans), fertilization occurs when the sperm's haploid set of chromosomes merges with the egg's haploid set, creating a diploid zygote with 46 chromosomes (23 pairs). o Fertilization restores the chromosome number, ensuring that the offspring inherits a complete set of genetic material, half from each parent. 3. Genetic Contribution: o The sperm and egg each contribute half of the genetic material (DNA) required to form the offspring. o Genes (segments of DNA) carry information that determines traits such as eye color, height, and susceptibility to diseases. o Alleles are different versions of a gene. The offspring inherits one allele from each parent for each gene. o Dominant and recessive alleles interact to determine which traits are expressed in the offspring. For example, a dominant allele may mask the expression of a recessive allele. 4. Development of the Embryo: o Once the zygote is formed, it undergoes mitotic divisions (cellular replication) to develop into a blastocyst, which implants in the uterine wall. o As the embryo develops, cells begin to differentiate into specialized tissues and organs, influenced by both genetic instructions (from DNA) and physiological signals (from the environment and the mother). o This involves complex interactions between genetics (the DNA blueprint) and physiology (how the cells function, interact, and organize). Interface of Physiology and Genetics in Reproduction 1. Genetic Information in Reproduction: o Genetics plays a critical role in determining the traits of offspring, passed down from both parents. The interaction between alleles determines phenotypes (observable traits), and mutations or changes in the DNA sequence can affect reproduction and development. o Genomic imprinting: In some cases, the expression of certain genes depends on whether they were inherited from the mother or the father. This is an example of how genetic inheritance and physiology interact. 2. Hormonal Regulation: o In females, hormones such as estrogen and progesterone regulate the menstrual cycle, oocyte maturation, and preparation for pregnancy. o In males, testosterone plays a key role in the production of sperm and the regulation of male reproductive physiology. o These hormones influence the timing and success of fertilization and also control the development of secondary sexual characteristics. 3. Environmental Influences on Reproduction: o Genetic predispositions can be affected by environmental factors. For example, nutrition, exposure to toxins, and even stress can influence fertility and the development of offspring. o Genetic mutations (either spontaneous or due to environmental influences like radiation) can have consequences for reproduction, such as birth defects or genetic disorders. 4. Gene Expression in Early Development: o After fertilization, the zygote's genetic information begins to direct its development through processes like gene expression and cell signaling. These processes guide the development of the embryo's organs and tissues. o Epigenetics, which involves changes in gene expression without altering the DNA sequence, can also play a role in how genes are expressed in different tissues, and in how offspring develop and respond to environmental conditions. 5. Reproductive Technologies: o Advances in assisted reproductive technologies (ART), such as in vitro fertilization (IVF) and genetic screening, highlight the intersection of physiology (reproductive health, hormone regulation) and genetics (genetic screening, embryo selection) in helping individuals achieve parenthood. Summary In summary, the process of reproduction involves both physiological mechanisms (such as hormonal regulation, gamete production, and embryo development) and genetic factors (such as inheritance patterns, gene expression, and genetic mutations). The combination of these elements ensures the creation of genetically diverse offspring and the continuation of species. The interface between physiology and genetics is crucial for understanding how the body functions during reproduction and how genetic information is transmitted to the next generation. Integration & Scale Here’s a breakdown of the biological hierarchy, showing the relationship between different levels of organization: 1. Whole Body o The entire organism, which includes all systems, tissues, cells, and genes working together. 2. Systems (11 primary systems in the body) o Muscular, Nervous, Circulatory, Respiratory, Urinary, Immune, Integumentary, Skeletal, Endocrine, Digestive, Reproductive. 3. Tissues (4 basic types) o Epithelial: Covers body surfaces and lines cavities. o Connective: Provides support and structure (e.g., bone, blood). o Muscle: Facilitates movement (e.g., skeletal, cardiac, smooth muscle). o Nervous: Transmits electrical signals (e.g., neurons). 4. Cells (~5 x 10¹³ cells in the human body) o The basic structural and functional units of life, each specialized for a particular role (e.g., red blood cells, muscle cells, neurons). 5. Genes (~30,000 genes in the human genome) o Segments of DNA that encode instructions for protein synthesis, determining the characteristics and functions of cells and organisms. This hierarchy shows how the complexity of biological organization increases from the level of the whole body down to the molecular level (genes) Scale: Genes & Proteins The scale of biological organization at the level of genes and proteins is as follows: 1. Chromosomes in the Nucleus o Humans have 46 chromosomes (23 pairs), each containing long strands of DNA that are organized into structures called chromatin. Chromosomes carry the genetic information necessary for growth, development, and functioning. 2. DNA: 3 x 10⁹ base pairs (bp) o The human genome contains approximately 3 billion base pairs of DNA. These base pairs are composed of adenine (A), thymine (T), cytosine (C), and guanine (G), which pair to form the double helix structure of DNA. 3. Genes (≈30,000) o Within this vast amount of DNA, there are about 30,000 genes, each being a sequence of DNA that codes for specific proteins. Genes are the functional units of heredity and encode instructions for building and maintaining the body. 4. DNA Transcription to RNA o A process called transcription converts DNA into messenger RNA (mRNA), which serves as a template for protein synthesis. This occurs in the nucleus. ▪ mRNA is synthesized using the DNA as a template, copying the genetic instructions. 5. RNA Translation into Protein o The mRNA leaves the nucleus and attaches to ribosomes in the cytoplasm. Here, it undergoes translation, a process in which the RNA code is used to synthesize proteins. ▪ tRNA (transfer RNA) helps to translate the mRNA sequence into an amino acid sequence, which folds into a protein. 6. Proteins: Determining Cell Function o The resulting proteins are crucial for the function of the cell. They can be enzymes, structural components, receptors, or regulators that carry out the majority of cellular functions. ▪ The specific sequence and structure of proteins determine their function in the cell, influencing processes such as metabolism, cell signaling, and gene expression. In summary, DNA is the blueprint, RNA is the messenger, and proteins are the workers that execute the functions within the cell. The examples you've provided—rhodopsin and CFTR—illustrate the relationship between protein size and its functional significance in organisms: 1. Rhodopsin (348 amino acids) o Function: Rhodopsin is a light-sensitive protein found in the retina. It plays a key role in vision, especially in low-light conditions, by initiating a signal transduction cascade in response to light exposure. o Organ vs. Protein Size: The retina, the organ in which rhodopsin is located, is approximately 107 times larger than the rhodopsin protein. This indicates that the protein is a small part of the overall organ structure, but essential for its function. The retina, as an organ, integrates many processes beyond the presence of rhodopsin, such as signal processing and integration with the brain. 2. CFTR (1480 amino acids) o Function: The CFTR (Cystic Fibrosis Transmembrane Conductance Regulator) protein acts as a chloride ion transporter in various secretory cells, including those in the lungs, pancreas, and intestines. It is crucial for regulating the flow of chloride ions and water across cell membranes, influencing the viscosity of secretions in the body. o Organism vs. Protein Size: The human organism is approximately 108 times larger than the CFTR protein. The protein is a key player in cellular processes, and defects in CFTR lead to cystic fibrosis, a condition affecting multiple organs. The organism as a whole contains a vast array of systems and functions, with CFTR being critical in regulating electrolyte balance and fluid movement across membranes. Key Insights: Protein Size vs. Organism/Organ Size: While proteins like rhodopsin and CFTR are relatively small in comparison to the organs or even the organism, they serve crucial, specialized roles that affect larger, more complex systems. Functionality in Complexity: The size of the protein is not always indicative of the complexity of the organism or organ. These proteins often interact with other molecules and cellular systems to perform their functions efficiently, making their relatively small size highly efficient for their roles in maintaining bodily functions. 4 tissue types Tissues are specialized groups of cells that work together to perform specific functions in the body. The four main types of tissue in animals are: 1. Muscle Tissue Function: Muscle tissue is responsible for the movement of the body and its parts, including voluntary movement (such as walking) and involuntary movement (such as heart contractions). Types: o Skeletal Muscle: Voluntary control, moves bones and the skeleton. o Cardiac Muscle: Involuntary control, found in the heart, responsible for pumping blood. o Smooth Muscle: Involuntary control, found in walls of organs (such as the intestines and blood vessels), helps with movement like peristalsis. 2. Nervous Tissue Function: Nervous tissue is responsible for transmitting electrical signals throughout the body. It coordinates body activities, processes information, and controls responses. Components: o Neurons: Specialized cells that transmit electrical impulses. o Glial Cells: Support, nourish, and protect neurons. 3. Connective Tissue Function: Connective tissue supports and binds other tissues and organs together. It provides structure, stores energy, and plays a role in immune responses. Types: o Loose Connective Tissue: Provides support and flexibility (e.g., adipose tissue). o Dense Connective Tissue: Strong, fibrous tissue found in tendons and ligaments. o Bone and Cartilage: Provide structural support. o Blood: Transports nutrients, gases, and wastes. 4. Epithelial Tissue Function: Epithelial tissue forms protective layers on body surfaces and organs, lines body cavities, and forms glands. It plays roles in absorption, secretion, and protection. Types: o Squamous Epithelium: Flat cells, found in areas where diffusion or filtration occurs (e.g., in the lungs and kidneys). o Cuboidal Epithelium: Cube-shaped cells, involved in secretion and absorption (e.g., in glands and kidneys). o Columnar Epithelium: Tall, column-shaped cells, often involved in absorption and secretion (e.g., in the digestive tract). o Transitional Epithelium: Cells that can change shape, found in the bladder and urinary system. Each type of tissue has a specialized role, but together they support the structure and function of the entire organism. Muscle tissue / Nervous tissue = excitable tissue Connective tissue / Epithelial tissue = non-excitable tissue Muscle Tissue (3 cell types) Function: Muscle tissue is responsible for movement in the body. It allows both voluntary and involuntary movements, including locomotion, circulation, digestion, and more. 1. Skeletal Muscle Location: Attached to bones (via tendons) Function: Voluntary movement of the skeleton, such as walking, lifting, and other controlled movements. Distinguishing Characteristics: - Long, cylindrical, and multinucleated cells. - Striated appearance (due to the arrangement of actin and myosin filaments). - Voluntary control via the somatic nervous system. Fibres: Skeletal muscle fibres are long, cylindrical, and multinucleated. These fibres are striated, meaning they have a banded appearance due to the regular arrangement of actin and myosin filaments that are responsible for muscle contraction. Location: Skeletal muscle is primarily attached to bones and tendons, with the exception of certain muscles such as the tongue, anal sphincter, and diaphragm. These muscles are responsible for voluntary movements of the skeleton, such as walking, running, and lifting. Arrangement: The muscle fibres are grouped into bundles known as fascicles. Several fascicles are then grouped together to form the entire muscle. This organization allows for coordinated and forceful contraction. Function: Movement of the Skeleton: When skeletal muscles contract, they generate force that pulls on bones through tendons, causing movement at joints. For example, the contraction of the biceps muscle causes the forearm to flex. Graded Contraction: Skeletal muscles can produce a range of force through graded contractions. This means that not all muscle fibres are recruited simultaneously; instead, groups of muscle fibres are activated progressively depending on the amount of force required for the movement. This is controlled by motor units (a motor neuron and all the muscle fibres it innervates), which can be recruited to contract in different numbers depending on the need for strength or precision. Contraction Mechanism: Skeletal muscles contract when motor neurons transmit signals to the muscle fibres. The signal causes the release of calcium ions, which initiate the sliding filament mechanism, where actin filaments slide past myosin filaments to shorten the muscle fibre, producing contraction. This recruitment mechanism allows for smooth, controlled movements and the ability to produce different levels of force depending on the task. 2. Smooth Muscle Location: Walls of internal organs (e.g., intestines, blood vessels, bladder, stomach). Function: Involuntary movements such as peristalsis (movement of food in the digestive tract), vasoconstriction (narrowing of blood vessels), and the regulation of airflow in the respiratory system. Distinguishing Characteristics: - Spindle-shaped, non-striated cells with a single central nucleus. - Involuntary control, regulated by the autonomic nervous system. Structure and Characteristics: Not Striated: Unlike skeletal and cardiac muscle, smooth muscle lacks the striations (alternating light and dark bands) caused by the organized arrangement of actin and myosin filaments. Instead, the filaments are arranged more randomly within the cells. Location: Smooth muscle is found in the walls of hollow organs and structures such as: o Digestive tract (e.g., stomach and intestines) o Urinary tract (e.g., bladder) o Reproductive organs o Blood vessels o Bronchioles in the lungs Function: Smooth muscle is responsible for the contraction of tubing-like structures in the body. It controls the movement of substances through these hollow organs by generating controlled contractions. Contractile Tubing: The smooth muscle fibres are arranged in circular layers around the lumen (the hollow space inside the organ). When the smooth muscle contracts, it narrows the lumen, pushing the contents of the tube forward. This is critical for processes like peristalsis in the digestive tract. Rhythmic Contraction: Smooth muscle contracts in a rhythmic manner, which is often involuntary and sustained. For example: o Peristalsis: Rhythmic waves of smooth muscle contractions that propel food and waste through the digestive tract. o Vasoconstriction and Vasodilation: In blood vessels, smooth muscle regulates blood flow by constricting or relaxing, affecting vessel diameter. Function in Different Systems: Digestive System: In the gastrointestinal tract, smooth muscle facilitates the movement of food by rhythmic contraction (peristalsis) and controls the sphincters that regulate the passage of food. Urinary and Reproductive Tracts: Smooth muscle controls the movement of urine from the kidneys to the bladder and the release of urine from the bladder, as well as the contraction of the uterus during childbirth. Blood Vessels and Bronchioles: Smooth muscle in blood vessel walls helps regulate blood pressure by contracting (vasoconstriction) or relaxing (vasodilation). In the bronchioles, smooth muscle regulates airflow by constricting or dilating the airways. Key Points: Involuntary Control: Smooth muscle is typically under involuntary control, regulated by the autonomic nervous system and various hormones. Slow, Sustained Contractions: Smooth muscle contractions are slower and more sustained compared to the fast, brief contractions of skeletal muscle, allowing for long-term processes like digestion, blood flow, and respiration. In summary, smooth muscle is essential for involuntary movements within internal organs and blood vessels, and its ability to contract rhythmically and sustain force helps regulate many of the body’s vital processes. 3. Cardiac Muscle Location: Heart Function: Pumps blood throughout the body by contracting the heart muscle. Distinguishing Characteristics: - Striated, branched cells with a single central nucleus. - Connected by intercalated discs, which allow synchronized contraction of the heart. - Involuntary control, regulated by the autonomic nervous system and the heart’s intrinsic pacemaker. Structure and Characteristics: Striated: Like skeletal muscle, cardiac muscle is also striated, due to the regular arrangement of actin and myosin filaments within the muscle fibres. However, cardiac muscle differs in that its cells are shorter and branched. Location: Cardiac muscle is found only in the heart. It makes up the walls of the heart chambers, responsible for pumping blood throughout the body. Interconnected Cells: The cardiac muscle fibres are interconnected to form a continuous fabric, enabling coordinated contractions across the heart. This arrangement allows for a unified contraction of the entire heart muscle, ensuring efficient blood circulation. Intercalated Discs: The muscle fibres are joined end-to-end by specialized structures called intercalated discs. These discs have two key functions: o Electrical Coupling: They contain gap junctions, which allow ions to flow between adjacent cells, facilitating the rapid transmission of electrical impulses across the muscle. o Mechanical Coupling: The discs also contain desmosomes, which help hold the cells together during contraction, providing structural integrity to the heart tissue. Function: Coordinated Contraction: The interconnected cells and the presence of intercalated discs ensure that the entire heart contracts as a single unit. This means all the fibres in the heart contract simultaneously, producing a strong, synchronized contraction that pumps blood effectively through the circulatory system. Automaticity: Cardiac muscle has intrinsic automaticity, meaning it can contract without external nervous stimulation due to pacemaker cells in the sinoatrial (SA) node of the heart. These cells generate electrical impulses that spread through the heart muscle, causing coordinated contraction and maintaining the heartbeat. In summary, cardiac muscle is specialized for the continuous, rhythmic contraction of the heart, and its striated structure, intercalated discs, and coordinated action ensure efficient blood flow throughout the body. Nervous tissue (2 types) Nerve Tissue Function: Nerve tissue is responsible for transmitting electrical impulses throughout the body, which are essential for coordination, regulation, and sensory perception. It enables the body to respond to internal and external stimuli by relaying information between different parts of the body and the brain. Location: Nerve tissue is primarily found in: Brain Spinal cord Nerves that branch out from the spinal cord to various parts of the body Distinguishing Characteristics: Connects to each other: Nerve cells (neurons) communicate with one another via electrical impulses and synapses. These impulses allow neurons to transmit information across the nervous system. Connects to other parts of the body: Neurons extend to other tissues, muscles, and organs, facilitating communication between the brain and the rest of the body, enabling motor control and sensory feedback. Cell Types in Nerve Tissue: There are two main types of cells in nerve tissue: 1. Neurons (Nerve Cells): o Function: Neurons are the functional cells of the nervous system that transmit electrical impulses. They can be sensory neurons (transmitting sensory information), motor neurons (controlling muscle movements), or interneurons (connecting different neurons). o Structure: Neurons have a unique structure that includes: ▪ Cell body: Contains the nucleus and organelles. ▪ Dendrites: Receive signals from other neurons or sensory cells. ▪ Axon: Transmits electrical impulses away from the cell body. ▪ Synaptic terminals: Release neurotransmitters to communicate with other neurons or target cells (e.g., muscles). 2. Glial Cells (Supporting Cells): o Function: Glial cells provide structural support, nutrition, and protection to neurons. They are involved in maintaining homeostasis, forming myelin (which speeds up nerve impulses), and supporting neuronal health. o Types of glial cells: ▪ Astrocytes: Provide support and maintain the blood-brain barrier. ▪ Oligodendrocytes (CNS) / Schwann Cells (PNS): Form the myelin sheath that insulates axons. ▪ Microglia: Act as immune cells in the central nervous system. ▪ Ependymal Cells: Line the ventricles of the brain and spinal cord. Key Features of Nerve Tissue: Excitability: Neurons are excitable cells, meaning they can generate electrical impulses in response to stimuli. Communication: The nervous system allows for quick, efficient communication within the body. Neurons transmit signals over long distances, such as from the spinal cord to muscles or from sensory receptors to the brain. Synaptic Transmission: Neurons communicate with one another at synapses, where electrical impulses are converted into chemical signals through the release of neurotransmitters. This allows the nervous system to coordinate complex functions like movement, cognition, and sensory perception. Summary: Nerve tissue plays a crucial role in the functioning of the nervous system. Its main cells, neurons and glial cells, work together to enable rapid communication across the body, allowing for sensory perception, motor control, and coordination of various bodily functions. Neurons Neuron Structure and Function: 1. Neurons: o Function: Neurons generate and conduct electrical impulses, also known as action potentials, that carry information throughout the body. This enables communication between the brain, spinal cord, and other body parts. 2. Dendrites (multiple): o Function: Dendrites are the tree-like branches extending from the cell body. Their primary function is to receive signals from other neurons or sensory receptors. They transmit these inputs to the cell body. 3. Cell Body: o Function: The cell body contains the nucleus and other organelles necessary for the neuron’s functioning. It integrates incoming signals from dendrites and generates an action potential if the signal is strong enough. The cell body also supports the metabolic and genetic functions of the neuron. 4. Axon (single): o Function: The axon is a long, single extension that conducts electrical impulses away from the cell body. It carries the action potential to axon terminals, where it can synapse with other neurons, muscles, or glands. This allows for communication with other cells. 5. Unidirectional Flow of Information: o The flow of electrical impulses in a neuron is unidirectional, meaning: ▪ Signals are received by the dendrites. ▪ The signal is processed in the cell body. ▪ If the signal is strong enough, it generates an action potential that travels along the axon to the terminals. ▪ The action potential is then transmitted to the next neuron or effector (muscle or gland). This system ensures that information is transmitted efficiently and in the correct direction, allowing for coordinated functions such as movement, sensation, and thought. The longest axon in humans can be found in the sciatic nerve, which runs from the spinal cord (in the lower back) to the foot. The axon of a single neuron in this nerve can be about 1 meter long or more, depending on the individual. Where does it start and finish? Start: The axon begins at the cell body of a motor neuron located in the spinal cord, specifically in the ventral horn of the spinal cord. Finish: The axon extends all the way down the leg and ends at the muscles of the lower limb (e.g., muscles in the thigh, calf, or foot), where it transmits signals for movement. The flow of information is unidirectional, meaning that electrical impulses are transmitted from the dendrites (which receive signals) to the cell body, and then down the axon to the axon terminals, where they can communicate with other neurons or muscles via synaptic transmission. Neuroglia You’re describing neuroglia (also known as glial cells), which play crucial roles in supporting neurons. Here's a more detailed explanation of their structure and function: Neuroglia (Glial Cells): 1. Structural & Functional Support: o Function: Neuroglia provide structural and functional support to neurons. They maintain the homeostasis of the nervous system, protect neurons, and facilitate the communication between neurons. o There are typically 5 neuroglial cells for every neuron in the nervous system. 2. Do Not Conduct Electrical Signals: o Unlike neurons, glial cells do not conduct electrical signals. Their primary role is to support, nourish, and protect neurons, rather than to transmit information themselves. 3. Ability to Divide: o One key distinction between neurons and neuroglial cells is that neuroglia can divide, whereas most neurons cannot divide after they are fully differentiated. This ability allows for repair and regeneration in the nervous system. 4. Types of Neuroglial Cells: o Oligodendrocytes (CNS): ▪ Location: Found in the central nervous system (CNS), which includes the brain and spinal cord. ▪ Function: They form the myelin sheath around axons in the CNS. The myelin sheath speeds up electrical signal transmission and ensures efficient communication between neurons. o Schwann Cells (PNS): ▪ Location: Found in the peripheral nervous system (PNS), which includes nerves outside the brain and spinal cord. ▪ Function: Similar to oligodendrocytes, Schwann cells also form the myelin sheath around axons in the PNS. They help with signal transmission and support neuron function in peripheral nerves. In summary, neuroglia play a supportive role in the nervous system, ensuring that neurons function optimally, with oligodendrocytes and Schwann cells being key players in the myelination process. Connective tissue (lots of cell types) Connective Tissue Function: Connective tissue has a broad range of functions, including: 1. Binding: It binds structures together, providing support and structure to organs and tissues. 2. Support: It supports and strengthens other tissues and organs in the body. 3. Protection: It provides a cushioning and protective layer around organs (e.g., the fat around kidneys). 4. Filling Spaces: Connective tissue fills the spaces between other tissues and organs. 5. Fat Storage: It stores fat in specialized cells (adipocytes), which can act as an energy reserve. 6. Blood Cell Production: Bone marrow, a type of connective tissue, produces blood cells. Location: Connective tissue is widely distributed throughout the body and can be found in nearly every organ. It serves as the "glue" that holds different parts of the body together, and it is located between and connected to other tissues. Distinguishing Characteristics: 1. Blood Vessels: Unlike other tissue types such as epithelial tissue, connective tissue has blood vessels (except in cartilage). These blood vessels supply nutrients and oxygen to the tissue and remove waste products. 2. Cell-Spaced Apart with Matrix: o Connective tissue cells are usually spread out with a matrix (a mixture of extracellular proteins and fluids) between them. The matrix gives the tissue its strength and rigidity. o The matrix can vary depending on the type of connective tissue (e.g., cartilage has a gel-like matrix, whereas bone has a hard, mineralized matrix). 3. Matrix Components: o The extracellular matrix (ECM) contains various proteins like collagen, elastin, and fibronectin, which provide structural support, elasticity, and cell adhesion. o The ECM can also include ground substance, which is a gel-like material composed of water, ions, proteins, and carbohydrates that helps support the cells and bind them together. 4. Types of Connective Tissue: o Loose Connective Tissue (e.g., areolar tissue, adipose tissue) o Dense Connective Tissue (e.g., tendons, ligaments) o Cartilage o Bone o Blood In Summary: Connective tissue is essential for structural integrity, storage, and protection within the body. Its flexibility in function and structure allows it to serve as a supportive framework, facilitating movement and providing metabolic support to organs and tissues. Dermis: Collagen Matrix and Cellular Components The dermis is the middle layer of the skin, located between the epidermis (outer layer) and the hypodermis (inner layer). It plays a vital role in supporting the skin and allowing it to perform its functions. Key Features of the Dermis: 1. Collagen Matrix: o The dermis is primarily composed of collagen, a fibrous protein that provides strength, structure, and flexibility to the skin. Collagen fibers form a dense network, creating a supportive matrix. o Elastin fibers, which provide elasticity, are also present, allowing the skin to stretch and return to its original shape. 2. Cell Types in the Dermis: o The dermis contains various cell types, each with specific functions: 1. Fibroblasts: These cells produce collagen, elastin, and other components of the extracellular matrix. They are crucial for tissue repair and regeneration. 2. Mast Cells: Involved in inflammatory responses, particularly in allergic reactions. 3. Macrophages: Part of the immune system, these cells help remove dead cells and pathogens through phagocytosis. 4. Adipocytes (Fat Cells): Found in the deeper layers of the dermis, they store fat and contribute to insulation and energy storage. 3. Blood Vessels: o The dermis contains a network of blood vessels that supply nutrients and oxygen to the skin cells. These vessels also help regulate body temperature by dilating or constricting based on environmental conditions. 4. Nerve Cells: o Nerve endings in the dermis allow for sensory functions, such as detecting pain, temperature, pressure, and touch. o Specialized structures such as Meissner's corpuscles (for light touch) and Pacinian corpuscles (for deep pressure and vibration) are located here. o These nerve cells are essential for the body's somatosensory system, enabling interaction with the external environment. 5. Hair Follicles: o The dermis also contains hair follicles, which are responsible for hair growth. These follicles are surrounded by sebaceous glands that secrete oil to lubricate the hair and skin. 6. Sweat Glands: o Sweat glands, located in the dermis, play a role in thermoregulation by secreting sweat to cool the body down. Functions of the Dermis: Support: The collagen matrix provides structural integrity, ensuring that the skin can withstand physical stress and prevent injury. Sensation: The nerve endings detect various stimuli and send signals to the brain, allowing the body to respond to the environment. Temperature Regulation: Blood vessels in the dermis help control body temperature by constricting (to conserve heat) or dilating (to release heat). Immune Defense: Cells like macrophages and mast cells help protect the body from infections and foreign invaders. In summary, the dermis plays a critical role in the structure and function of the skin, supporting cells and tissues, aiding in sensory perception, and contributing to the body's immune response and temperature regulation. The collagen matrix is foundational to the dermis, providing both strength and elasticity, while the blood vessels and nerves enable essential physiological processes. The depth at which a cut causes bleeding depends on the layers of tissue it affects, as well as the blood vessels located in those tissues. Here's a breakdown of what happens when a cut reaches different depths: 1. Epidermis (outer skin layer): o The epidermis is the outermost layer of the skin, composed of dead skin cells and a protective barrier. A cut that only affects the epidermis does not typically cause bleeding, as there are no blood vessels in this layer. However, it may cause minor irritation or pain. 2. Dermis (middle skin layer): o The dermis contains blood vessels, nerve endings, hair follicles, and connective tissue. If a cut reaches the dermis, it will likely cause bleeding, as blood vessels (such as capillaries) in this layer are cut open. The depth of the cut needed to reach the dermis is typically around 1–2 millimeters, depending on the individual and the area of the skin. 3. Hypodermis (subcutaneous layer): o The hypodermis is the deepest layer of skin, containing larger blood vessels (such as veins and arteries) and fatty tissue. Cuts that penetrate deeper into the hypodermis may cause more significant bleeding, as larger blood vessels are damaged. These cuts typically occur when the injury is deeper than 2 millimeters, depending on the area (for example, on the face, this layer is closer to the surface). 4. Muscle or bone: o If the cut reaches deeper layers, such as muscle tissue or bone, it will result in more severe bleeding. The muscles contain larger blood vessels (arteries and veins), and bone fractures can cause significant bleeding from blood vessels in the bone marrow or periosteum. Summary: A cut typically needs to reach the dermis (around 1–2 millimeters deep) before you start to bleed, as the dermis contains the capillaries responsible for bleeding. Cuts that go deeper into the hypodermis or muscle tissue cause more significant bleeding due to the larger blood vessels present. Adipose tissue Adipocytes (fat cells) are specialized cells that store fat in the body. They play a crucial role in energy storage, insulation, and cushioning organs. Here's an overview of their structure and function: 1. Structure of Adipocytes: o Fat Globules: Adipocytes store lipids (primarily in the form of triglycerides) within large fat globules. These globules occupy most of the cell's volume and are the primary energy reservoir of the body. o Cytoplasm: The cytoplasm of adipocytes contains enzymes that regulate the size of the fat globules by controlling the storage and breakdown of lipids. o Nucleus: The nucleus is usually pushed to the periphery of the cell due to the large size of the fat globule. 2. Size of Fat Globules: o The size of the fat globule within the adipocyte can vary depending on the body’s energy needs. When the body has excess energy (calories), the fat globules increase in size as more triglycerides are stored. Conversely, during periods of energy deficit (such as fasting or exercise), the fat globules shrink as triglycerides are broken down and released as fatty acids into the bloodstream for energy. o The size of these fat globules is regulated by enzymes in the cytoplasm of adipocytes. Key enzymes include: ▪ Lipoprotein lipase (LPL): This enzyme is involved in the uptake of fatty acids into the adipocyte from the blood. It helps in storing fat. ▪ Hormone-sensitive lipase (HSL): HSL plays a role in breaking down triglycerides within the adipocyte when the body needs to mobilize stored fat for energy. 3. Regulation: o The enzymes in the adipocyte cytoplasm are influenced by hormonal signals. For example, insulin promotes fat storage by stimulating LPL activity and inhibiting HSL, whereas hormones like adrenaline and cortisol promote the breakdown of stored fat by activating HSL and inhibiting LPL. 4. Function of Adipocytes: o Energy Storage: Adipocytes store excess calories in the form of fat for later use. This stored energy is crucial during periods o