Neuro-Ophthalmology: Afferent Visual System PDF
Document Details
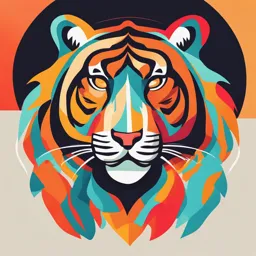
Uploaded by FinestScandium
Matthew J. Thurtell, Sashank Prasad, Robert L. Tomsak
Tags
Related
- Neuro Reading Summary Quiz 1 Semester 2, 23
- Neuro 1 Degeneration (PDF)
- Neurooftalmologi 2024 PDF
- Clinical Signs, Imaging, and Outcome of Internal Ophthalmoparesis/Ophthalmoplegia in Cats (PDF)
- Neuro-Ophthalmology: Ocular Motor System PDF
- Vascular Neurology Board Review Questions and Answers (2nd Edition) PDF
Summary
This document outlines the neuro-ophthalmology: afferent visual system. It covers anatomy, physiology, and examination techniques.
Full Transcript
16 Neuro-Ophthalmology: Afferent Visual System Matthew J. Thurtell, Sashank Prasad, Robert L. Tomsak OUTLINE Afferent Visual System Anatomy and Physiology, 164 Progressive Visual Loss, 177 Neuro-Ophthalmological Examination of the Afferent Visual...
16 Neuro-Ophthalmology: Afferent Visual System Matthew J. Thurtell, Sashank Prasad, Robert L. Tomsak OUTLINE Afferent Visual System Anatomy and Physiology, 164 Progressive Visual Loss, 177 Neuro-Ophthalmological Examination of the Afferent Visual Nonorganic (Functional) Visual Disturbances, 178 System, 165 Diagnostic Techniques, 178 Examination of Visual Acuity, 165 Prognosis, 178 Contrast Vision Testing, 165 Optic Nerve and Retinal Disorders, 178 Light-Stress Test, 165 Optic Disc Edema, 178 Color Vision Testing, 165 Unilateral Optic Disc Edema, 179 Examination of the Pupils, 166 Bilateral Optic Disc Edema, 184 Light Brightness Comparison, 168 Pseudopapilledema, 185 Visual Field Testing, 168 Optic Neuropathies with Normal-Appearing Optic Discs, 186 Interpretation of Visual Field Defects, 169 Unilateral Presentations, 186 Examination of the Ocular Fundus, 170 Bilateral Presentations, 186 Ancillary Diagnostic Techniques, 171 Optic Neuropathies with Optic Atrophy, 187 Perimetry, 171 Congenital Optic Disc Anomalies, 187 Ophthalmic Imaging, 171 Tilted Optic Disc, 187 Electrophysiology, 172 Optic Nerve Dysplasia, 187 Approach to the Patient With Visual Loss, 172 Retinal Disorders, 188 Pattern of Visual Loss, 172 Retinal Arterial Disease, 188 Central Visual Loss, 172 Retinal Vein Occlusion, 189 Peripheral Visual Loss, 173 Retinal Degenerations, 190 Temporal Profile of Visual Loss, 174 Phakomatoses, 190 Sudden-Onset Visual Loss, 174 AFFERENT VISUAL SYSTEM ANATOMY AND The signal from cone and rod photoreceptors reaches a ganglion cell after being modulated by bipolar, horizontal, and amacrine cells (Fig. PHYSIOLOGY 16.2). Two main types of retinal ganglion cell exist: parasol cells (M retinal From a conceptual standpoint, it is useful to consider vision as having ganglion cells, which project to the magnocellular pathway) and midget two components: central or macular vision (high acuity, color percep- cells (P retinal ganglion cells, which project to the parvocellular pathway). tion, and light-adapted) and peripheral or ambulatory vision (low acuity, Retinal nerve fibers form arcuate bundles respecting the midline hori- poor color perception, and dark-adapted). Light, refracted by the cornea zontal raphe and enter the optic disc superiorly and inferiorly (Fig. 16.3). and lens, is focused on the retina. For the best possible vision, the image Nerve fibers exit the globe via the scleral canal, where they receive physical of the object of regard must fall onto the fovea, which is the most sensi- support from the lamina cribrosa and metabolic support from intertwin- tive part of the macula. The cone photoreceptors, which mediate central ing astrocytes. Once nerve fibers pass through the lamina cribrosa, they are and color vision, are the greatest in density at the fovea. The cone system supported by oligodendrocytes and become myelinated. Nerve fibers that functions optimally in conditions of light adaptation. Visual acuity and arise from the ganglion cells of the nasal retina of each eye decussate in the cone density fall off rapidly as eccentricity from the fovea increases. For optic chiasm to the contralateral optic tract, while those from the temporal example, the retina 20 degrees eccentric to the fovea can only resolve retina do not decussate. The percentages of crossed and uncrossed fibers objects equivalent to Snellen 20/200 (6/60 metric) optotypes or larger. in the human optic chiasm are approximately 53% and 47%, respectively. Rod photoreceptors are present in the highest numbers approximately Visual information stratifies further in the lateral geniculate 20 degrees from the fovea and are more abundant than cones in the nucleus (LGN), which is the only way station between the retinal more peripheral retina; rods function best in dim illumination. The total ganglion cells and the primary visual cortex. The LGN, a portion extent of the normal peripheral visual field in each eye is approximately of the thalamus, has six layers. Axons from ipsilateral retinal gan- 60 degrees superior, 60 degrees nasal, 70–75 degrees inferior, and 100 glion cells synapse in layers 2, 3, and 5; contralateral axons synapse degrees temporal to fixation (Fig. 16.1). Because of the optical properties in layers 1, 4, and 6. Layers 1 and 2 of the LGN are the magnocel- of the eye, the nasal retina receives visual information from the temporal lular layers, and these receive input from M retinal ganglion cells. visual field, while the temporal retina receives visual information from The magnocellular pathway is concerned mainly with movement the nasal visual field (see Fig. 16.1). Similarly, the superior retina receives detection, detection of low contrast, and dynamic form perception. visual information from the inferior visual field and vice versa. These After projecting to the primary visual cortex (visual area 1, V1, or points are clinically important when evaluating visual loss. Brodmann area 17), information from the M pathway is distributed 164 #*" ##%%"'"' "' %"' ! #!'")%&'+#&#"&"&#"%#! " +#!+ &)% CHAPTER 16 Neuro-Ophthalmology: Afferent Visual System 165 Examination of Visual Acuity Visual acuity is the spatial resolution of vision. Visual acuity should always be measured in each eye individually and with the best pos- sible optical correction (i.e., with the patient’s glasses); other optical Temporal means such as a pinhole device or refraction may be needed if optical field correction is not available. The resulting measure, called best-cor- rected visual acuity, is the only universally interpretable measurement of central visual function. Ideally, visual acuity should be measured both at distance (usually 20 feet or 6 m) and near (usually 14 inches or 0.33 m). The notation 20/20 (6/6) indicates that the patient (numerator) is able to see the optotypes seen by a normal person at Left Right 20 feet (denominator). A visual acuity of 20/60 (6/18) indicates that the patient sees an optotype at 20 feet that a normal person would see at 60 feet. Optic nerve A disparity between the distance and near visual acuities is often indicative of a specific problem. For example, the most common Chiasm cause of better distance than near acuity is uncorrected presbyopia. Common causes of better near than distance acuity include myopia Optic tract and congenital nystagmus. In the latter disorder, convergence needed for near vision dampens the nystagmus. Meyer loop When measuring near vision, the reading card should be held at Lateral the specified distance of 14 inches (or 0.33 m) to control for variation geniculate in image size on the retina. The medical record should clearly specify nucleus if a nonstandard distance is used. Two types of near cards are readily available; one has numbers, and the other has written text (Fig. 16.4). Visual In neurological practice, a near card with text measures visual acu- radiations ity as well as reading ability to some degree. A disparity between the measurements from the two types of near card might suggest a distur- Visual cortex bance of some other cortical function, such as language function (see Chapter 13). Fig. 16.1 Visual Pathways. Contrast Vision Testing Contrast vision, the ability to distinguish adjacent areas of differing to V2 (part of area 18) and V5 (junction of areas 19 and 37). Layers luminance, can be evaluated by assessing the perception of lines or 3–6 of the LGN are the parvocellular layers and receive input from optotypes of different sizes (spatial frequencies) with varying degrees P retinal ganglion cells, which are color selective and responsive of contrast. Contrast vision can be impaired in numerous diseases of to high contrast. Information from the P pathway is distributed to the eye (e.g., cataract) and retrobulbar visual pathways (e.g., optic neu- V2 and V4 (fusiform gyrus). Superior fibers that leave the LGN go ropathies). Special charts—the Pelli–Robson chart (sensitivity) and straight back to the primary visual cortex, while inferior fibers loop Sloan chart (acuity)—are required to assess contrast vision. anteriorly around the temporal horn of the lateral ventricle (Meyer loop). Because these fibers pass close to the tip of the temporal lobe, Light-Stress Test temporal lobectomy sometimes damages these fibers, causing a “pie- In some disorders of the macula, abnormalities are not apparent with in-the-sky” homonymous visual field defect. the ophthalmoscope. The light-stress (or photo-stress) test is a useful The primary visual cortex (striate cortex, V1, or Brodmann area 17) method for determining whether reduced central vision is a conse- is in the occipital lobe. Fibers from the macula project to the portion quence of macular dysfunction. Prior to the test, the best-corrected of the visual cortex closest to the occipital poles, while fibers from the visual acuity is measured in each eye. Then, with the eye with decreased peripheral retina project to the visual cortex lying more anteriorly. The vision occluded, the other eye is exposed to a bright light for 10 sec- nonoverlapping part of the most peripheral temporal visual field (mon- onds. Immediately thereafter, the patient is instructed to read the next ocular temporal crescent) arises from unpaired crossed axons from the largest line on the eye chart, and the recovery period is timed. The nasal retina that project to the most anterior portion of the visual cor- same procedure is followed for the eye with decreased vision, and the tex. The primary visual cortex has interconnections with visual asso- results are compared. Fifty seconds is the upper limit of normal for ciation areas concerned with color, motion, and object recognition. visual recovery, although most normal subjects recover within several seconds. In patients with macular disease, the recovery period often NEURO-OPHTHALMOLOGICAL EXAMINATION OF takes several minutes. THE AFFERENT VISUAL SYSTEM Color Vision Testing The neuro-ophthalmological examination makes use of ophthalmic Dyschromatopsia, especially if asymmetrical between the eyes, is an tools and techniques but aims at neurological diagnosis. Because many indication of optic nerve dysfunction but can also occur with retinal neurologists are not familiar with ophthalmic examination techniques, disease (Almog and Nemet, 2010). Symmetrical acquired dyschroma- and ophthalmologists are often not experienced with neurological topsia might indicate a retinal degeneration, such as a cone–rod dys- localization, the neuro-ophthalmological subspecialty provides a trophy. Congenital dyschromatopsia occurs in about 8% of men and bridge between the two disciplines. 0.5% of women. #*" ##%%"'"' "' %"' ! #!'")%&'+#&#"&"&#"%#! " +#!+ &)% 166 PART I Common Neurological Problems Temporal Parafovea Fovea centralis Parafovea Inner limiting membrane/nerve fiber layer Foveola Ganglion cell layer Inner nuclear layer Outer nuclear layer Photoreceptor layer Retinal pigment epithelium Choriocapillaris Choroid Inner limiting membrane/nerve fiber layer 250 µm Ganglion cell layer Inner nuclear layer Outer nuclear layer Photoreceptor layer Retinal pigment epithelium Choriocapillaris Choroid Inner limiting membrane Nerve fiber layer Ganglion cell layer Amacrine cells (inner nuclear layer) Bipolar cells (inner nuclear layer) Horizontal cells (inner nuclear layer) Müller cells Outer limiting membrane Cones Rods Retinal pigment epithelium Fig. 16.2 Structures of the Neurosensory Retina. Top, high-resolution optical coherence tomography. Mid- dle, histological section. Bottom, schematic depiction of retinal layers. (Adapted and reprinted with permis- sion from Jaffe, G., Caprioli, J., 2004. Optical coherence tomography to detect and manage retinal disease and glaucoma. Am J Ophthalmol 137, 156–169 and http://www.webvision.med.utah.edu.) Papillomacular Arcuate Optic Techniques for assessing color vision range from the simple to the bundle bundles disc sophisticated. A gross color vision defect is identifiable at the bedside by assessing for red desaturation. The clinician holds a bright red object in front of each of the patient’s eyes individually and asks for a compar- ison of both brightness and color intensity. Asking for a comparison of red saturation on each side of fixation sometimes detects a subtle hemianopia. Formal measurements of color vision can be obtained with pseudoisochromatic color plates (e.g., Ishihara or Hardy–Rand– Rittler plates) or with sorting tests (e.g., Farnsworth–Munsell test). Fovea T N Examination of the Pupils Examination of the pupils involves assessing pupil size and shape, the direct and consensual reactions to light, and the near response. The examination should also include an assessment for a relative afferent pupillary defect (RAPD). If a difference in pupil size (anisocoria) is noted, look for ptosis and ocular motility deficits, keeping in mind the possibility of Horner syndrome or third cranial nerve palsy. Record findings in an easily understood format (Table 16.1). Measurements of pupil size and light reaction are made in dim illu- Horizontal raphe mination with the patient fixating on an immobile distant target. If there is anisocoria, it is useful to measure pupil size in both darkness Fig. 16.3 Arrangement of Retinal Nerve Fiber Layer, Composed of Ganglion Cell Axons. The papillomacular bundle conveys axons from and bright light. Anisocoria due to oculosympathetic paresis (Horner the fovea directly to the temporal margin (T) of the optic disc. The remain- syndrome) is greater in the dark because the affected pupil does not der of temporal ganglion cell axons is arranged in arcuate bundles above dilate well. Conversely, anisocoria due to parasympathetic denerva- and below the fovea, arriving at the superior and inferior disc margins. tion (e.g., Adie tonic pupil) is more evident in bright light because the Finally, axons originating nasal to the disc arrive at its nasal border (N). affected pupil does not constrict well (see Chapter 17). #*" ##%%"'"' "' %"' ! #!'")%&'+#&#"&"&#"%#! " +#!+ &)% CHAPTER 16 Neuro-Ophthalmology: Afferent Visual System 167 A B Fig. 16.4 A, Rosenbaum-style near vision card. B, Near vision card with written text. TABLE 16.1 Simple Method of Recording The presence of an RAPD (formerly called a Marcus Gunn pupil) is Pupillary Examination an invaluable sign of a unilateral or asymmetric optic neuropathy. An RAPD is best detected by alternately illuminating the pupils by swing- Size Direct Light Consensual Near ing a flashlight between them at a frequency of about once per sec- (mm) Reaction Light Reaction Reaction ond—hence the name, swinging flashlight test. The swinging flashlight Right eye 4.0 4+ 2+ 4+ test compares the direct and consensual light reactions in the same eye. Left eye 4.0 2+ 4+ 4+ Normally, these reactions are equal. However, in patients with a unilat- eral or asymmetric optic neuropathy, because of reduction in the direct When measuring light reactions or assessing for an RAPD, the reaction as compared with the consensual reaction, the pupil of the eye brightest light available should be used. The near reaction can be with decreased vision dilates when reilluminated (both pupils are actu- elicited by having the patient look at his or her thumb, positioned ally “dilating” in that they are both resetting at the size commensurate at a distance of 15–30 cm. With this method, a near reaction can be with the amount of light transmitted back to the brain by the damaged elicited even in a completely blind patient, owing to proprioceptive optic nerve). Box 16.1 and Fig. 16.5 describe the method for detecting influences. The pupil shows light-near dissociation when the direct an RAPD. Two caveats exist. The test brings out an asymmetry of optic light reaction is less prominent than the near reaction. Light-near dis- nerve conduction, so an RAPD is not present when both optic nerves sociation can be seen with parasympathetic denervation of the pupil are injured to the same extent. In addition, severe inner retinal pathol- (e.g., Adie tonic pupil), with dorsal midbrain lesions (e.g., as part of ogy can produce an RAPD, but the abnormality is usually obvious on the dorsal midbrain syndrome), and in patients with severe bilateral funduscopic examination. In contrast, an optic neuropathy with mini- optic neuropathies. mal loss of visual acuity often gives an obvious RAPD. The magnitude #*" ##%%"'"' "' %"' ! #!'")%&'+#&#"&"&#"%#! " +#!+ &)% 168 PART I Common Neurological Problems BOX 16.1 Testing for a Relative Afferent Pupillary Defect 1. The patient should fixate on an immobile distant target to minimize fluctu- ations in pupillary size and accommodative miosis. 2. A light bright enough to cause maximum pupillary constriction should be A used. 3. Each pupil should be checked individually for its direct light response, which can be graded on a scale of 1–4 (see Table 16.1). 4. The light should be moved quickly to illuminate each eye alternately every 1 second (the swinging flashlight test). 5. The pupil should be observed for initial constriction or dilation. 6. Only three or four swings of the light should be made, to minimize bleach- B ing of the retina, and subsequent slowing of the pupillary reactions. of an RAPD can be quantified using neutral density filters. See Chapter 17 for further discussion of pupillary abnormalities. Light Brightness Comparison Light brightness comparison is a subjective swinging flashlight test. C The subjective appreciation of light intensity is often impaired in Fig. 16.5 Right Relative Afferent Pupillary Defect from a Right Optic patients with optic neuropathies, but not in macular disease. The cli- Nerve Lesion. A, Poor direct and consensual reaction with illumination nician shines a bright light into both eyes in succession and asks the of the right eye. B, Excellent direct and consensual reaction with illumi- patient to estimate the difference in brightness. For example, the cli- nation of the left eye. C, Poor direct and consensual reaction, manifest nician could ask, “If this light (normal eye illuminated) were worth $1 as redilation of both pupils when the light is swung back to the right eye. in terms of light brightness or intensity, what would this one be worth (abnormal eye illuminated)?” 4. Simultaneous hand comparison: Finally, the clinician holds both hands open, first in both upper quadrants and then both lower Visual Field Testing quadrants, and asks the patient to compare the quality of the Evaluation of the visual fields is vital in patients with visual loss. Several images. For example, when shown hands on either side of the mid- techniques can be used for visual field examination, ranging from sim- line, a patient with a subtle bitemporal hemianopia may state that ple confrontation testing to sophisticated threshold static perimetry. the hands held in the temporal hemifields are not as clear as those Confrontation testing should be part of the routine neurological exam- held in the nasal hemifields. ination, although it is insensitive for detection of mild visual field loss A potential advantage of the finger counting method over kinetic (Kerr et al., 2010). For the purposes of this discussion, the emphasis is methods (e.g., wiggling fingers) is that it minimizes the potential for on simple and practical techniques, while more sophisticated methods confounding by the Riddoch phenomenon, which refers to a dissocia- are briefly summarized. tion between the visual perception of form and movement such that In the first assessment, the patient is asked to observe the clinician’s the patient can perceive moving but not stationary targets in half of face with each eye in turn and to report if any part of the clinician’s the visual field (Zeki and Ffytche, 1998). The Riddoch phenomenon face is missing, blurred, or distorted when the patient’s line of sight is can occur when homonymous hemianopia results from visual cortex directed to the nose. For example, a patient with a central scotoma may lesions. Accordingly, the clinician may miss a hemianopia when using report that the eyes and nose are missing, a patient with an inferior only a moving target, such as wiggling fingers, in the far periphery. altitudinal visual field defect may report that the lower half of the face Confrontation methods using colored (e.g., red) objects can be is missing, while a patient with homonymous hemianopia may report effective in detecting subtle visual field defects (Kerr et al., 2010). that one side of the face is missing. Confrontation testing is also useful for assessing patients with con- Confrontation testing should follow. Although many methods stricted visual fields. As the distance between the clinician and the are available, a simple, thorough examination can be done by finger patient increases, the visual field should expand, producing a funnel. counting in all four quadrants, coupled with hand comparison. The However, with nonorganic (functional) visual field constriction, the steps are as follows: visual field often does not expand as the distance between the clinician 1. The clinician has the patient occlude one eye and maintain fixation and the patient increases, thereby producing a tunnel (Fig. 16.6). on the clinician’s nose. The central 20 degrees of the visual field can be assessed in each eye 2. Finger counting in the quadrants: The clinician holds up fingers separately using the Amsler grid (Fig. 16.7). With the grid held in good sequentially in each of the four quadrants of the visual field and light at a distance of 30 cm from the eye and the patient wearing his or asks the patient to count the number seen. her reading glasses, if needed, the following questions are asked: 3. Simultaneous finger counting using both hands: If step 2 is completed 1. Can you see the spot in the center of the grid? normally, the clinician asks the patient to count the number of fin- 2. While looking at the center spot, can you see the entire grid or are gers displayed with both hands, first in both of the upper quadrants any sides or corners missing? of the patient’s visual field and then in the lower quadrants. Then, 3. While you are looking at the center spot, are any of the lines in the the patient is asked to add the total number of fingers shown with grid missing, blurred, or distorted? both hands. Visual inattention is often identifiable during this step If the patient indicates an abnormality, the clinician should ask the of confrontation testing. patient to draw the abnormal areas on the grid. The grid can then be kept #*" ##%%"'"' "' %"' ! #!'")%&'+#&#"&"&#"%#! " +#!+ &)% CHAPTER 16 Neuro-Ophthalmology: Afferent Visual System 169 2x 2x 2x x x x A B C Fig. 16.6 A, The normal visual field enlarges with an increase in testing distance; it “funnels.” B, The con- stricted visual field from organic disease also proportionally enlarges. C, The constricted visual field from nonorganic disease does not usually enlarge as testing distance increases; rather, it “tunnels.” (Adapted from Trobe, J.D., Glaser, J.S., 1983. The Visual Fields Manual: a Practical Guide to Testing and Interpretation. Gainesville, Triad, p. 135.) optic neuropathy often produces abnormalities in both the peripheral and the central portions of the visual field (Fig. 16.8). A lesion at the junction of the optic nerve and chiasm produces a junc- tional scotoma (i.e., ipsilateral cecocentral scotoma and contralateral tem- poral defect) due to involvement of both ipsilateral fibers and crossing fibers from the contralateral nasal retina. Binasal field defects (Fig. 16.9) can result from papilledema, anterior ischemic optic neuropathy, glaucoma, optic nerve head drusen, optic nerve pits, optic nerve hypoplasia, and sectoral ret- initis pigmentosa (RP). Binasal field defects that are organic in etiology do not respect the vertical meridian, whereas nonorganic binasal defects may. Rule 2 True bitemporal hemianopias are the hallmark of chiasmal disease. Bitemporal field defects that do not respect the vertical meridian (pseudo- bitemporal hemianopias) are almost always due to congenital rotation or tilting of the optic discs (Fig. 16.10). Bilateral cecocentral scotomas can masquerade as bitemporal field defects, and the distinguishing feature is whether the defect respects the vertical meridian of the visual field. Rule 3 Fig. 16.7 Amsler Grid. Upper left, Paracentral scotoma. Lower right, A homonymous visual field defect is present in the same hemifield (i.e., Metamorphopsia (straight lines appear wavy). right or left) or visual quadrant (i.e., upper or lower) of each eye. The only exception to this rule is with the monocular temporal crescent in the patient’s medical record. Patients with a central scotoma may report syndrome, in which only unpaired visual fibers residing in the contra- that the center of the grid is missing, those with a hemianopic defect may lateral anteromedial occipital lobe are affected. report that half of the grid is missing, and those with macular disease may report that the lines are wavy or distorted (i.e., metamorphopsia). Rule 4 Incongruent hemianopias tend to result from more anterior retrochi- Interpretation of Visual Field Defects asmal lesions (e.g., those affecting the optic tract or temporal lobe; Fig. Eight general rules for visual field interpretation are summarized in 16.11; Kedar et al., 2007). Optic tract lesions often produce a contralat- Box 16.2. Comments relating to six of these general rules are presented eral RAPD (i.e., in the eye with the temporal visual field defect), which here. is helpful for clinical localization. Rule 1 Rule 5 Optic nerve lesions can produce prechiasmal visual field abnormalities Congruent homonymous hemianopias have patterns that are very sim- that are characteristic. Nonarteritic anterior ischemic optic neuropa- ilar or identical in the two eyes. Highly congruent hemianopias usually thy (NAION) often produces an inferior altitudinal defect, optic neu- result from occipital lobe infarcts (Fig. 16.12) but can sometimes occur ritis often produces a central or cecocentral scotoma, and compressive with more anterior retrochiasmal lesions (Kedar et al., 2007). #*" ##%%"'"' "' %"' ! #!'")%&'+#&#"&"&#"%#! " +#!+ &)% 170 PART I Common Neurological Problems BOX 16.2 General Rules of Visual Field Interpretation 1. Lesions of the retina and optic nerve produce visual field defects in the ipsilateral eye only, unless the lesions are bilateral. 2. Only a lesion of the optic chiasm causes true bitemporal hemianopia. 3. Retrochiasmal lesions produce homonymous visual field defects. 4. Anterior retrochiasmal lesions produce incongruent homonymous visual field defects. 5. Posterior retrochiasmal lesions produce congruent homonymous visual field defects. 6. Temporal lobe lesions give slightly incongruent homonymous hemianopias Left Right involving the upper quadrant. Fig. 16.10 Pseudobitemporal hemianopia (e.g., due to tilted optic discs). 7. No localizing value can be assigned to a complete homonymous hemiano- Note that the vertical meridian is not respected. pia, except that the lesion is retrochiasmal and contralateral to the visual field defect. 8. A unilateral homonymous hemianopia does not reduce visual acuity. Left Right Left Right Fig. 16.8 Constriction of the left visual field with a cecocentral scotoma Lesion due to compressive optic neuropathy, with a normal right visual field. Fig. 16.11 Incongruent left homonymous hemianopia from a right optic tract lesion. Left Right Examination of the Ocular Fundus Fig. 16.9 Binasal visual field defects (e.g., due to glaucoma). Note that Examination of the ocular fundus, to evaluate for abnormalities in the the vertical meridian is not respected. appearance of the optic disc, retinal vasculature, and macula, is man- datory in patients with visual loss. The steps, when using the direct ophthalmoscope, are as follows: Rule 8 1. The room lights are dimmed. Even a complete unilateral homonymous hemianopia does not 2. The clinician has the patient maintain fixation on a distant target decrease visual acuity because the macular cortex in the opposite to minimize miosis due to accommodation. hemisphere is intact. If the input to both macular cortices is impaired, 3. The clinician holds the ophthalmoscope with their right hand and central acuity is often diminished (cortical blindness), but the visual looks through their right eye when evaluating the patient’s right acuities should be equally diminished. If the visual acuities are not sim- eye, and vice versa when evaluating the left eye. ilar, the clinician should search for another (or additional) explanation 4. The clinician begins the examination positioned temporally to the for the asymmetry. patient at arm’s length. #*" ##%%"'"' "' %"' ! #!'")%&'+#&#"&"&#"%#! " +#!+ &)% CHAPTER 16 Neuro-Ophthalmology: Afferent Visual System 171 detection of subtle architectural abnormalities. Electrophysiological techniques may be used to evaluate objectively the function of the ret- ina and optic nerves. Imaging techniques for evaluating the afferent visual pathways and cortical areas involved in visual processing are discussed in Chapter 40. Perimetry Numerous techniques for examining the visual fields are available (Sample et al., 2011), but a detailed discussion of these is beyond the scope of this chapter. Examination of the entire visual field requires Left Right a perimeter; the tangent screen measures only the central 30 degrees of the visual field at a distance of 1 m. Perimeters can be divided into those that use a moving (kinetic) stimulus and those that use a static stimulus. Most static perimeters are automated and driven by computer. Static perimeters can determine the visual threshold at defined points in the visual field (threshold static perimetry) or may evaluate these points using stimuli of set luminance (suprathreshold static perimetry). The Goldmann perimeter is the most commonly used kinetic perimeter (see Fig. 16.13, A for a normal visual field obtained using the Goldmann perimeter), although kinetic perimetry can also be performed with the Humphrey and Octopus perimeters. The Humphrey and Octopus perimeters are the most commonly used static perimeters (see Fig. 16.13, B for a normal visual field obtained using the Humphrey perimeter). Threshold static perimeters are the most sensitive and quantitative, allowing for a comparison of the patient’s responses with those of age-matched normal controls, but testing can be time consuming and tiring for the patient. To gain useful Lesion information from static perimetry, the patient must be alert, cooper- ative, and able to maintain steady central fixation. Many patients with neurological disorders are unable to concentrate for an examination Fig. 16.12 Congruent paracentral right homonymous hemianopia from that can take as long as 15 minutes per eye, and, thus, static perimetry a left occipital pole lesion. findings may be unreliable in such patients. Recent refinements in test- ing strategy have made it possible to reduce testing time and thereby 5. The clinician evaluates for the red reflex, which may be absent increase reliability, but many neuro-ophthalmologists continue to use when there is a media opacity (e.g., dense cataract or vitreous Goldmann perimetry to assess the visual fields in selected patients. hemorrhage). 6. The clinician moves closer to the patient while adjusting the focus Ophthalmic Imaging of the ophthalmoscope. Photographs of the ocular fundus may be obtained to identify and 7. When the clinician is close to the patient and the retina is in focus, document ophthalmoscopic findings. Retinal vascular abnormali- a retinal vessel is followed until the optic disc is found. ties, such as occlusions (e.g., central retinal artery occlusion [CRAO]) 8. The clinician evaluates the appearance of the optic disc for edema, and microvascular disease (e.g., diabetic retinopathy), may be evalu- pallor, and cupping, and then the peripapillary region for the ated when red-free fundus photographs are taken following intrave- presence of hemorrhages, cotton-wool spots, exudates, and reti- nous (IV) injection of fluorescein (fluorescein angiography). Fundus nal folds. autofluorescence photography allows for topographical mapping of 9. The clinician evaluates the appearance of the retinal vasculature lipofuscin in the retinal pigment epithelium layer. Lipofuscin is a flu- arising from the optic disc. orescent pigment that accumulates in retinal pigment epithelial cells 10. Lastly, the clinician evaluates the appearance of the macula, which following photoreceptor degradation, and, thus, autofluorescence may is located temporal to the optic disc. be used to detect subtle abnormalities in patients with retinal diseases Examination with the direct ophthalmoscope can be difficult in (Schmitz-Valckenberg et al., 2008). Because optic nerve head drusen patients who have small pupils. In such patients, pharmacological dila- exhibit autofluorescence, they may be detected on fundus autofluores- tion of the pupils should be considered; there is minimal risk of induc- cence even when they are not visible on ophthalmoscopy (Kurz-Levin ing angle-closure glaucoma in patients with normal anterior chamber and Landau, 1999). anatomy (Patel et al., 1995). Optical coherence tomography (OCT) uses light waves to generate high-resolution cross-sectional images of the optic nerve and retina. As the retinal layers have differing optical reflectivity, they can be distin- ANCILLARY DIAGNOSTIC TECHNIQUES guished using OCT. The thickness of the layers can be determined from Ancillary diagnostic tests may be obtained to further characterize and OCT and compared with age-matched normal controls. Measurement determine the cause of visual loss (see Chapter 43). Formal visual field of the peripapillary retinal nerve fiber layer and macular ganglion cell testing (perimetry) allows for characterization and quantification of layer thicknesses with OCT may help with the detection of mild optic visual field defects. Ophthalmic imaging techniques may not only neuropathy (Fig. 16.14). Measurement of the thickness of retinal layers be used to image the ocular fundus and blood vessels but also now or identification of architectural changes on OCT can aid the diagnosis allow for measurement of the thickness of individual retinal layers and and management of retinal disease. #*" ##%%"'"' "' %"' ! #!'")%&'+#&#"&"&#"%#! " +#!+ &)% 172 PART I Common Neurological Problems 120 105 90 75 60 70 135 45 60 50 150 30 40 30 165 15 20 10 180 90 8 0 70 60 50 40 30 20 10 10 20 30 40 50 60 70 80 90 0 10 I1e 20 195 345 30 12e 40 210 330 50 I4e 60 225 315 70 A 240 255 270 285 300 Fig. 16.13 Kinetic and Automated Static Perimetry. A, Kinetic perimetry of the right visual field using the Goldmann perimeter demonstrates an intact visual field. The isopters (I1e, I2e, and I4e) indicate the locations in the visual field where the subject perceived each stimulus. The scotoma 10–20 degrees right of center is the physiological blind spot. Electrophysiology Ophthalmic causes of visual loss are often not readily apparent to the Electrophysiology may help in the investigation of unexplained visual loss neurologist, whereas neurological causes of visual loss often confuse or in identification of subclinical optic nerve dysfunction. Measurement ophthalmologists. Thus, the approach to evaluating visual loss must be of visual-evoked potentials (VEPs) has long been used for the evaluation systematic, so that sinister causes are not missed, and simple causes are of demyelinating optic neuropathies, which produce a delayed P-100. not overinvestigated. The localization and cause of visual loss can often However, VEP findings can be misleading; a low-amplitude VEP could be inferred from the pattern and temporal profile of visual loss. Here, be misinterpreted as indicating optic neuropathy in a patient with reti- we briefly discuss the differential diagnosis of visual loss based on its nal disease. Electroretinography (ERG) is useful for the evaluation of sus- pattern and temporal profile, as well as nonorganic (functional) visual pected retinal dysfunction, especially when ophthalmoscopic findings disturbances. A comprehensive description of optic nerve and retinal are subtle or absent. Full-field ERG evaluates the response of the entire disorders is presented in the final section of the chapter. retina to flashes of light. A variety of stimuli are presented in differing states of light adaptation, allowing for evaluation of different retinal PATTERN OF VISUAL LOSS elements, including the rod and cone photoreceptors. Because full-field ERG evaluates the response of the entire retina, it may not be abnor- Central Visual Loss mal in patients with focal retinal dysfunction (e.g., macular dysfunc- A defect in the visual field surrounded by normal vision is called a sco- tion). Multifocal ERG allows for the topographic evaluation of macula toma, from the Greek word meaning “darkness.” Loss of central vision, ERG responses and is more sensitive for detecting macular dysfunction resulting in a central or cecocentral scotoma, is usually quickly noticed, (Sutter and Tran, 1992); multifocal ERG findings can be grossly abnor- while peripheral visual field defects, such as homonymous hemiano- mal even when ophthalmoscopic changes are absent or subtle. pia, can be asymptomatic. However, when noticed, they are frequently referred to the eye with the greater extent of field loss (i.e., the eye with temporal field loss; Fig. 16.15). Central and cecocentral scotomas APPROACH TO THE PATIENT WITH VISUAL LOSS are usually due to lesions of the optic nerve or macula. A lesion at the Visual loss commonly accompanies neurological disease and is one of junction of the optic nerve and chiasm produces a junctional scotoma the most disturbing symptoms a patient may experience. While visual with an ipsilateral central scotoma, due to optic nerve involvement, loss is often due to a benign or treatable process, it can be the first sign and a contralateral temporal field defect, due to chiasmal involvement of a blinding or life-threatening disease. Common causes of visual loss (Fig. 16.16). Patients with junctional scotomas are often unaware of include uncorrected refractive error, corneal disease, cataract, glaucoma, the contralateral temporal field defect, emphasizing the importance of retinal disease (e.g., age-related macular degeneration), and amblyopia. assessing each eye separately during visual field evaluation. #*" ##%%"'"' "' %"' ! #!'")%&'+#&#"&"&#"%#! " +#!+ &)% CHAPTER 16 Neuro-Ophthalmology: Afferent Visual System 173 B Fig. 16.13, cont’d B, Automated static perimetry of the central 24 degrees of the right visual field using the Humphrey perimeter (24-2 SITA-standard program) demonstrates an intact visual field. Upper, The testing strategy, stimulus size, and stimulus color are indicated. Upper left, The reliability indices (number of fixation losses, false-positive rate, and false-negative rate) and the foveal visual threshold (in decibels) are displayed. The decibel (dB) is a logarithmic relative scale to quantify differential light sensitivity (1 dB = 0.1 log-unit of stimulus intensity). Upper center and right, Threshold sensitivity plot (displays raw threshold data for each test location) and grayscale plot (displays interpolated data; darker areas indicate areas of visual field loss). The physiological blind spot is 10–20 degrees right of center. Lower left and center, The total deviation plots indicate deviations from age-adjusted normal values at each test location (in dB and as a probability of being abnormal). The pattern deviation plots indicate the deviations with adjustment for generalized depression of the visual field (e.g., due to refractive error, media opacity, or pupillary miosis). Lower right, The mean devi- ation (MD, mean of all total deviation values) is a global indicator of the severity of visual field loss. Negative values indicate greater visual field loss. The pattern standard deviation (PSD) provides a measure of the uniformity of the visual field loss, such that diseases causing highly focal defects (e.g., glaucoma) will have a high PSD, whereas those causing diffuse visual field loss will have a low PSD. In general, scotomas caused by retinal disease are so-called positive which case they may be evoked by sound). Photopsias can also occur as scotomas because they are perceived as a black or gray spot in the visual part of migraine visual aura. Aside from ocular diseases, bilateral cen- field. Patients with macular pathology can also have metamorphop- tral visual loss can result from lesions involving both optic nerves, the sia; metamorphopsia is almost always caused by retinal disease (e.g., optic chiasm, or the visual cortex concerned with central vision. The age-related macular degeneration, macular edema, and epiretinal possibility of nonorganic (functional) visual loss must be considered, membrane). In contrast, optic nerve lesions characteristically produce but it remains a diagnosis of exclusion. negative scotomas, areas of absent vision that are otherwise not per- ceivable, in conjunction with decreased color vision, contrast vision, Peripheral Visual Loss and light brightness perception. On occasion, paradoxical photopho- For simplicity, visual field defects can be classified into one of three bia, especially with fluorescent lighting, can occur with optic nerve groups: prechiasmal, chiasmal, or retrochiasmal. Unilateral prechias- lesions. Photopsias (light flashes) can occur with vitreoretinal traction mal lesions affect the visual field of one eye only, chiasmal lesions affect (e.g., posterior vitreous detachment), retinal disease (e.g., cancer-asso- the visual fields of both eyes in a nonhomonymous bitemporal fashion, ciated retinopathy [CAR]), toxicity from certain drugs (e.g., digitalis), and retrochiasmal lesions cause homonymous visual field defects with or optic nerve disease (e.g., in the healing phase of optic neuritis, in varying degrees of congruity depending on their location (Fig. 16.17). #*" ##%%"'"' "' %"' ! #!'")%&'+#&#"&"&#"%#! " +#!+ &)% 174 PART I Common Neurological Problems ONH and RNFL OU Analysis: Optic Disc Cube 200!200 OD OS Ganglion Cell OU Analysis: Macular Cube 200!200 OD OS 225 150 75 0!m A B Fig. 16.14 Optical Coherence Tomography of the Optic Nerves and Macula. A, Optical coherence tomog- raphy of the optic nerve head (OHN) and retinal nerve fiber layer (RNFL) from the right eye (OD) and left eye (OS), obtained using the Cirrus optic disc cube protocol. The top panel shows the RNFL thickness map, with the average RNFL thickness (in micrometers), optic disc area, and the cup-to-disc (C/D) ratio for each eye. Neuro-retinal rim and RNFL thicknesses are plotted below for quadrants of the optic nerves (S, superior; N, nasal; I, inferior; T, temporal) compared with the distribution from age-matched normal controls. The RNFL thicknesses fall within the normal range in both eyes. B, Optical coherence tomography showing the macular ganglion cell analysis from the right eye and left eye, obtained using the Cirrus macular cube protocol. The top panel shows the ganglion cell thickness map for each eye. The ganglion cell layer thicknesses are plotted below for the six sectors of the macula compared with the distribution from age-matched normal controls. The ganglion cell layer thicknesses fall within the normal range for both eyes (OU). TEMPORAL PROFILE OF VISUAL LOSS Visual loss in bright light. Some patients with reduced blood supply to the eye due to a high-grade stenosis or occlusion of the Sudden-Onset Visual Loss internal carotid artery report TMVL in bright light, which is thought Visual loss of sudden onset can be divided into three temporal pat- to be due to impaired regeneration of photopigments secondary to terns: transient (Box 16.3; Thurtell and Rucker, 2009), nonprogressive, ocular ischemia (Kaiboriboon et al., 2001). The TMVL can also occur and progressive. following meals or with postural changes. A variety of ophthalmic abnormalities can be present and collectively comprise the ocular Transient Monocular Visual Loss ischemic syndrome (Chen and Miller, 2007). Other retinal diseases, Amaurosis fugax. The term amaurosis fugax is often used to such as cone dystrophies and age-related macular degeneration, can describe the transient monocular visual loss (TMVL) caused by emboli cause evanescent visual loss in bright light, also known as hemeralopia from the carotid arteries, aorta, or heart to the retinal circulation. or day blindness. The visual loss in these diseases is usually bilateral, Typically, these attacks are sudden in onset, last for several minutes, and whereas it is unilateral in patients with unilateral carotid disease. are characterized by altitudinal visual field loss that is often described Uhthoff phenomenon. TMVL with increases in body temperature as being similar to a curtain descending over the eye (Donders, 2001). is known as the Uhthoff phenomenon and most commonly occurs in Patients may also describe having separate attacks with hemispheric patients with a history of optic neuritis, but it can also occur in patients symptoms, such as weakness and aphasia, rather than visual loss. with other optic neuropathies. The phenomenon is thought to arise as Retinal artery vasospasm. TMVL can be caused by retinal artery a result of transient conduction block within the optic nerve. Vision vasospasm and is called retinal migraine when accompanied by migraine returns to baseline when the body temperature returns to normal. headache (Hill et al., 2007). Vasospastic TMVL is usually benign and Transient visual obscurations. Transient visual obscurations often responds to calcium channel blockers (Winterkorn et al., 1993). are brief episodes of monocular or binocular visual loss in patients Angle-closure glaucoma. Attacks of angle-closure glaucoma with optic disc edema due to increased intracranial pressure (ICP). should also be considered in the differential diagnosis of TMVL, The visual loss is often precipitated by postural changes or Valsalva- especially if the patient reports seeing halos around lights or has like maneuvers (e.g., coughing and straining) and probably occurs associated eye pain, injection, or vomiting. Urgent ophthalmic secondary to transient hypoperfusion of the edematous optic nerve consultation should be obtained to prevent irreversible visual loss. head. The visual loss lasts for only a few seconds, with vision rapidly #*" ##%%"'"' "' %"' ! #!'")%&'+#&#"&"&#"%#! " +#!+ &)% CHAPTER 16 Neuro-Ophthalmology: Afferent Visual System 175 60 60 100 Left Right 75 Left Right Fig. 16.15 Right Homonymous Hemianopia. The visual loss is often referred to the right eye because the right temporal visual field is larger than the left nasal visual field. Numbers refer to the normal extent of the visual field in degrees. returning to baseline thereafter. Similar episodes of visual loss can occur with systemic hypotension, giant cell arteritis (GCA), or retinal venous stasis. Gaze-evoked transient visual loss has been reported with orbital tumors but can occasionally occur with optic disc edema. Fig. 16.16 Junctional Scotoma from a Lesion at the Junction of the Other causes of transient visual loss. Transient visual loss can Optic Nerve and Chiasm. The lesion affects both the right optic nerve, also occur as a result of transient optic nerve compression by cystic producing a cecocentral scotoma in the right eye, and crossing fibers lesions, such as paranasal sinus mucoceles and craniopharyngiomas. in the optic chiasm, producing a temporal visual field defect in the left Other ophthalmic causes of TMVL include impending central retinal eye. The temporal visual field defect of a junctional scotoma often goes vein occlusion and recurrent hyphema, although it is important to unnoticed by the patient and may only be detected with visual field note that some causes (e.g., corneal basement membrane dystrophy testing. and tear film dysfunction) produce visual blurring rather than actual visual loss. (PION), occurring due to loss of blood supply to the retrobulbar Transient Binocular Visual Loss portion of the optic nerve, is far less common but can occur in the Other than transient visual obscurations occurring in patients with perioperative period (e.g., during prolonged prone spine surgery or bilateral optic disc edema, simultaneous complete or incomplete tran- cardiac bypass surgery) or with hemodynamic shock (Rucker et al., sient binocular visual loss is almost always due to transient dysfunction 2004). GCA should be specifically considered in elderly patients of the visual cortex. Visual migraine aura is probably the most common with PION. cause of transient binocular visual loss (see Chapter 102). Transient Optic nerve ischemia almost never results from embolism. In con- binocular visual loss can also result from cerebral hypoperfusion due trast, central or branch retinal artery occlusions (BRAOs) are caused to vasospasm, thromboembolism, systemic hypotension, hyperviscos- mostly by embolic or thrombotic events. Opacification of the retinal ity, or vascular compression (Box 16.4; Thurtell and Rucker, 2009). nerve fiber layer with a cherry-red spot at the macula is the classic fun- Transient binocular visual loss can occur in association with seizures, duscopic appearance of acute CRAO. Retinal arterial occlusions can although they more commonly cause visual hallucinations, which can produce altitudinal, quadrantic, or complete monocular visual loss. be elementary or complex, depending on the location of the seizure The triad of BRAOs, hearing loss, and encephalopathy results from a focus (Bien et al., 2000). Transient cortical blindness can occur in asso- rare microangiopathy known as Susac syndrome (Susac et al., 2007). ciation with headache, altered mental status, and seizures in the poste- Occlusion of the central retinal vein can result in sudden visual loss rior reversible encephalopathy syndrome (PRES; Hinchey et al., 1996). and an unmistakable hemorrhagic retinopathy. It usually occurs in Transient cortical blindness can sometimes occur after head trauma, patients with risk factors for atherosclerosis and results from venous especially in children. Lastly, transient bilateral visual loss can occa- thrombosis at the level of the lamina cribrosa of the sclera. When sionally be nonorganic in etiology, but this should remain a diagnosis ischemic, it causes a dense central scotoma with sparing of peripheral of exclusion. vision. Idiopathic central serous retinopathy can manifest as a positive Sudden Monocular Visual Loss Without Progression central scotoma of sudden onset, often with metamorphopsia or Visual loss due to optic nerve or retinal ischemia is characteristically micropsia and a positive light-stress test result. It results from leak- sudden in onset (Box 16.5) and is usually nonprogressive, although age of fluid into the subretinal space and most often occurs in young a stepwise decline in vision may occur over several weeks in some adult men with type A personalities. The diagnosis can be difficult to patients with anterior ischemic optic neuropathy. Anterior ischemic make without the aid of fluorescein angiography or OCT, as the ret- optic neuropathy is a common cause of optic neuropathy and occurs inal findings are subtle. Spontaneous recovery usually occurs within due to loss of blood supply to the optic nerve head, resulting in weeks to months, but occasionally laser photocoagulation is required optic disc edema (Rucker et al., 2004). In affected patients younger to seal leaking vessels. than 50 years, it is usually nonarteritic in etiology, being caused by a Traumatic optic neuropathy (TON) usually results in sudden per- combination of factors that impair blood supply to the optic nerve manent optic nerve dysfunction. The trauma can be severe or decep- head. In patients older than 50 years, giant cell (temporal or cranial) tively minor, causing a contusion or laceration of the optic nerve or a arteritis must be considered. Posterior ischemic optic neuropathy shearing of its nutrient vessels with subsequent infarction. #*" ##%%"'"' "' %"' ! #!'")%&'+#&#"&"&#"%#! " +#!+ &)% 176 PART I Common Neurological Problems Normal blind spots (1) Lesion in left superior temporal retina causes a corresponding field defect in the left inferior nasal visual field. Left visual field Right visual field Left nasal (2) Total blindness right eye. retina (3) Chiasmal lesion causes Complete lesion of right bitemporal hemianopia. optic nerve. 1 2 Right 3 temporal retina Left optic nerve 4 Right optic tract Lateral (4) Right incongruous geniculate body 5 (5) Left homonymous hemianopia due to a superior quadrantanopia lesion of the left optic due to lesion of inferior tract (least common optic radiations in site for hemianopia). temporal lobe. Corpus colliculi 6 Geniculocalcarine tract Left occipital lobe 7 (6) Right homonymous inferior (7) Right congruous quadrantanopia due to incomplete homonymous involvement of optic radiations hemianopia. (upper-left optic radiation in this case). (8) Right homonymous hemianopia due to a lesion of the left hemisphere. The pupillary light reflex is not impaired if the lesion is beyond the tract. Fig. 16.17 Topographical Diagnosis of Visual Field Defects. (Reprinted with permission from Vaughn, C., Asbury, T., Tabbara, K.F., 1989. General Ophthalmology, twelfth ed. Appleton & Lange, Norwalk, CT, p. 244.) Sudden Binocular Visual Loss Without Progression Sudden Visual Loss with Progression Sudden, permanent, binocular visual loss most commonly results from Sudden-onset, painful monocular visual loss that subsequently wors- strokes involving the retrochiasmal visual pathways and causes hom- ens is commonly due to optic neuritis. The visual loss typically pro- onymous visual field defects (Box 16.6; Rizzo and Barton, 2005). In gresses over days before stabilizing and then improving. Optic neuritis patients who have no other neurological symptoms or signs, the lesion is well known to be associated with multiple sclerosis (MS) and may be is usually in the occipital lobe. Bilateral occipital lobe infarcts can the first sign of the disease. The prognosis for visual recovery without result in tubular visual field defects, checkerboard visual field defects, treatment is excellent in most patients, although there is a poor recov- or complete loss of vision in both eyes, a condition called cortical or ery in some, such as those with optic neuritis occurring in association cerebral blindness. Cortical blindness, especially from infarction, can be with neuromyelitis optica (NMO; Wingerchuk et al., 2007). accompanied by a denial of the visual loss and confabulation, a condi- Leber hereditary optic neuropathy (LHON), a maternally trans- tion known as Anton syndrome. mitted disease resulting from mutations in the mitochondrial deoxy- Sudden binocular visual loss can occasionally result from simulta- ribonucleic acid (DNA) genes encoding subunits of respiratory chain neous bilateral ischemic optic neuropathies (especially in the perioper- complex I, can also cause sudden painless central visual loss with sub- ative period) or from chiasmal compression due to pituitary apoplexy. sequent progression. The visual loss initially may be monocular or bin- Pituitary apoplexy can also cause headache, diplopia, ptosis, altered ocular, but the fellow eye is almost always affected within a few weeks mental status, and hemodynamic shock (Sibal et al., 2004), but the to months and certainly by 1 year. Visual recovery is variable and infre- presentation can be subtle such that the diagnosis is missed. quent and depends on the mitochondrial DNA mutation. #*" ##%%"'"' "' %"' ! #!'")%&'+#&#"&"&#"%#! " +#!+ &)% CHAPTER 16 Neuro-Ophthalmology: Afferent Visual System 177 BOX 16.3 Causes of Transient Monocular BOX 16.6 Causes of Sudden Binocular Visual Loss Visual Loss Without Progression Retinal circulation emboli Occipital lobe stroke Migraine/vasospasm Bilateral ischemic optic neuropathies Hypoperfusion (hypotension, hyperviscosity, hypercoagulability) Pituitary apoplexy Ocular (optic disc edema, intermittent angle-closure glaucoma, hyphema, Head trauma impending central retinal vein occlusion) Nonorganic (functional) visual loss Vasculitis (e.g., giant cell arteritis) Other (Uhthoff phenomenon, idiopathic, nonorganic) BOX 16.7 Causes of Progressive Visual Loss BOX 16.4 Causes of Transient Binocular Anterior visual pathway inflammation: Visual Loss Optic neuritis Migraine Sarcoidosis Cerebral hypoperfusion: Meningitis Thromboembolism Anterior visual pathway compression: Systemic hypotension Tumors Hyperviscosity Aneurysms Seizures Thyroid eye disease Posterior reversible encephalopathy syndrome Hereditary optic neuropathies: Head trauma Leber hereditary optic neuropathy Optic disc edema (transient visual obscurations) Dominant optic atrophy Optic nerve head drusen Glaucoma and normal-tension glaucoma Chronic papilledema BOX 16.5 Causes of Sudden Monocular Toxic (e.g., ethambutol) and nutritional optic neuropathies Visual Loss Without Progression Radiation damage to anterior visual pathways Central or branch retinal artery occlusion Paraneoplastic (cancer-associated) retinopathy or optic neuropathy Anterior ischemic optic neuropathy, arteritic or nonarteritic Posterior ischemic optic neuropathy Branch or central retinal vein occlusion there are central or cecocentral scotomas with sparing of the peripheral Traumatic optic neuropathy visual field and temporal pallor and cupping of the optic discs. Color Central serous retinopathy vision is usually abnormal. Other ophthalmic and neurological abnor- Retinal detachment malities may be present (Yu-Wai-Man et al., 2009). Vitreous hemorrhage Optic disc drusen are a common cause of pseudopapilledema and Nonorganic (functional) visual loss can produce visual field defects including enlargement of the physio- logical blind spot, arcuate defects, and generalized constriction (Lee and Zimmerman, 2005). Loss of visual acuity is atypical but can result Careful questioning of the patient with “sudden” visual loss may from development of a secondary choroidal neovascular membrane, reveal a long-standing deficit that has suddenly been noticed (e.g., with subsequent hemorrhage into the macula, or anterior ischemic when covering the fellow eye) or that has worsened over time. In such optic neuropathy. cases, the clinician should evaluate for a slow-growing compressive Glaucoma is a common cause of progressive visual field loss. The lesion (Box 16.7). visual field defects are arcuate, and central vision is spared until late. Glaucoma is usually bilateral and symmetric, with associated increased Progressive Visual Loss intraocular pressure and optic disc cupping. In normal-tension glau- Progressive visual loss is the hallmark of a lesion compressing the afferent coma, glaucomatous optic disc and visual field changes develop despite visual pathways. Common compressive lesions include pituitary tumors, normal intraocular pressure (Anderson et al., 2001). aneurysms, craniopharyngiomas, and meningiomas (see Box 16.7; Chronic papilledema from any cause of intracranial hypertension Gittinger, 2005; Glaser, 1999). Granulomatous disease of the optic nerve can produce progressive optic neuropathy (see Chapter 88). The visual from sarcoidosis or tuberculosis can cause chronic progressive visual loss. fields become constricted, with nasal defects occurring initially, followed Optic nerve compression at the orbital apex from thyroid eye disease can by gradual constriction, with central vision being spared until late. occur with minimal orbital signs or ocular motility disturbance. In each Toxic and nutritional optic neuropathies are bilateral and usually of these cases, the visual loss can be so insidious as to go unnoticed until progressive (Phillips, 2005; Tomsak, 1997). The nutritional variety it is fortuitously discovered during a routine examination. is characterized by gradual onset of painless visual loss over weeks to Hereditary or degenerative diseases of the optic nerves or retina months, prominent dyschromatopsia, cecocentral scotomas, and devel- must be included in the differential diagnosis of gradual-onset visual opment of optic atrophy late in the disease. Medications that are toxic to loss. The hereditary optic neuropathies are bilateral and are usually the optic nerves, including ethambutol, amiodarone, and linezolid, can diagnosed during the first two decades of life (Yu-Wai-Man et al., cause a gradual onset of painless visual loss (Phillips, 2005). Retinal tox- 2009). The most common inherited optic neuropathy is the autosomal ins, such as vigabatrin, digitalis, chloroquine, hydroxychloroquine, and dominant variety, known as dominant optic atrophy. Characteristically, phenothiazines, can also cause painless progressive binocular visual loss. #*" ##%%"'"' "' %"' ! #!'")%&'+#&#"&"&#"%#! " +#!+ &)% 178 PART I Common Neurological Problems BOX 16.8 Some Forms of Nonorganic Visual Disturbance Visual acuity loss (one or both eyes) Visual field loss (unilateral or bilateral) Color perception abnormalities Convergence/accommodative insufficiency Spasm of the near triad Loss of depth perception Diplopia Night blindness Left Right Photophobia Fig. 16.18 Two common visual field abnormalities with nonorganic Pharmacological pupils visual loss. Left, Concentric (tubular) constriction. Right, Spiraling of an Voluntary nystagmus isopter (seen only with kinetic visual field testing). Slowly progressive visual loss from radiation damage to the anterior cloverleaf constriction, spiraling of an isopter, crossing isopters, or visual pathways or retina can result from direct radiation therapy to inverted isopters (Fig. 16.18). Confrontation or tangent screen testing the eye or periocular irradiation. It can also occur after whole-brain done at different distances can be useful in evaluating a patient with visual irradiation or parasellar irradiation (Lessell, 2004). Its incidence field constriction, as the visual field area should increase as the distance relates to fraction size and total radiation dose. Radiation-induced between the patient and the clinician increases. Lack of expansion of capillary endothelial cell damage is the initial event that triggers the visual field area with increasing distance from the clinician suggests non- damage. Radiation retinopathy may be indistinguishable from diabetic organic visual field constriction (see Fig. 16.6). Cloverleaf constriction is retinopathy. best detected using automated static perimetry and cannot be produced Rapidly progressive bilateral visual loss can be caused by paraneo- by organic disease. Spiraling, crossing, and inversion of isopters can be plastic processes that affect the retina or, less commonly, optic nerves detected using kinetic perimetry and cannot be produced by organic dis- (Ko et al., 2008). Small-cell carcinoma of the lung is the most com- ease. Kinetic perimetry with both eyes opened in a patient with suspected monly associated tumor, but gynecological, endocrine, and breast nonorganic monocular visual loss may demonstrate nonphysiological tumors have been implicated. With CAR, features include photopsias, visual field constriction on the side of the reported visual loss. night blindness or nyctalopia, constricted visual fields, and an extin- The use of VEPs to diagnose nonorganic visual loss can be unre- guished electroretinogram. Combined treatment with chemotherapy liable. If the VEP is normal, useful information is gained, but facti- and immunosuppression may be effective in occasi