Protein Degradation Notes PDF
Document Details
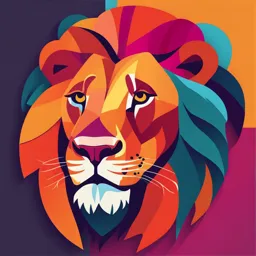
Uploaded by HonorableTsavorite
MUSC
Annamarie Dalton, PhD
Tags
Related
- Lecture 13: Protein Processing, Targeting, and Degradation PDF
- Biochemistry - Amino Acids Metabolism PDF
- BIOCHEMISTRY AMINO ACIDS: NITROGEN DISPOSAL (Part 1) PDF
- BIOCHEMISTRY AMINO ACIDS: NITROGEN DISPOSAL (Part 1) PDF
- Amino Acid Metabolism and Urea Cycle PDF
- Lecture 31, Amino Acid Degradation - PDF
Summary
These notes provide an overview of protein degradation, including dietary protein digestion and the recycling of intracellular proteins. The document covers nitrogen balance, essential and nonessential amino acids, and important pathways like the ubiquitin-proteasome and lysosomal pathways.
Full Transcript
Protein Degradation INSTRUCTOR: Annamarie Dalton, PhD [email protected] Department of Biochemistry and Molecular Biology BSB Room 535E, 843-792-5660 OUTLINE: I. Overview of protein degradation II. Degradation of dietary proteins a. Nitrogen balance b. Essential vs. nonessential amino acids c. Dietar...
Protein Degradation INSTRUCTOR: Annamarie Dalton, PhD [email protected] Department of Biochemistry and Molecular Biology BSB Room 535E, 843-792-5660 OUTLINE: I. Overview of protein degradation II. Degradation of dietary proteins a. Nitrogen balance b. Essential vs. nonessential amino acids c. Dietary protein digestion III. Recycling of intracellular proteins a. Purpose of intracellular protein degradation b. Ubiquitin-proteasome pathway i. Ubiquitin conjugation ii. Proteasomal degradation c. Lysosomal pathway IV. Summary LEARNING OBJECTIVES: After studying this unit, you should be able to: 1. 2. 3. 4. 5. 6. 7. 8. 9. List the sources of amino acids for cellular metabolism. Distinguish between nitrogen balance, negative nitrogen balance, and positive nitrogen balance. Explain why essential amino acids cannot be made in humans. Specify two methods by which the protein quality of foods can be assessed. Explain why serine and glycine are not considered essential amino acids. Explain the purpose of intracellular protein degradation and describe how this process regulates cholesterol biosynthesis. Describe the steps involved in protein ubiquitination and the subsequent degradation by proteasomes. Discuss the therapeutic role of proteasome inhibitors in the treatment of cancer. Provide examples of lysosomal degradation of proteins. READING REFERENCE: • Marks’ Basic Medical Chemistry, 6th edition–Chapter 35: Protein Digestion and Amino Acid Absorption ACKNOWLEDGEMENTS: Special thanks to Dr. Yi-Te Hsu for providing the syllabus template. Some of the figures featured in this lecture were generated using Biorender.com. Protein Degradation-Dalton 1 I. Overview of protein degradation Sources of amino acids 1 •degradation of dietary proteins 2 •normal recycling of cellular proteins Amino acids can be derived from the degradation of ingested proteins or from the normal breakdown (recycling) of cellular proteins. During starvation, breakdown of tissue and muscle proteins will also occur for energy generation. II. Degradation of dietary proteins a. Nitrogen balance Recommended dietary allowances Males Females 45-63 g of protein 46-50 g of protein •Daily requirement 0.8 g/kg of body weight •Vegetarians must ingest more plant proteins The amount of protein needed to meet the daily requirements differs with the source of dietary protein. The average adult requirement for high-quality protein is 0.8 g/kg of body weight daily. Protein Degradation-Dalton 2 States of nitrogen balance Nitrogen balance: The amount of nitrogen ingested is equal to the amount of nitrogen excreted Negative nitrogen balance: More nitrogen is excreted than ingested (starvation, injuries, certain diseases, and senescence) Positive nitrogen balance: Less nitrogen is excreted than ingested (in growing children and pregnant women) Nitrogen balance is a measure of nitrogen input versus nitrogen output. Blood urea nitrogen (BUN) is commonly used to measure nitrogen output. A person is said to be in nitrogen balance when the amount of nitrogen ingested equals the amount of nitrogen excreted. If a person does not ingest enough protein, he or she will experience negative nitrogen balance. This means that the excretion of nitrogen is greater than the amount of nitrogen being taken in by the body. It is interesting to note that physical trauma can cause a negative nitrogen balance. This would include surgery. The mechanism for this phenomenon is not well understood. A positive nitrogen balance occurs when less nitrogen is excreted than taken in. Such would take place during growth or when one is recovering from a protein-deficient diet. Positive nitrogen balance also occurs in pregnancy. Nitrogen balance, however, does not indicate whether a particular essential amino acid is lacking in the diet. b. Essential vs. nonessential amino acids Assessment of the protein quality of food Protein Eggs Milk Rice Beef Fish Corn Chemical score NPU 100 93 86 75 75 72 100 75 67 80 83 56 •Chemical score - based on the essential amino acid content of food •NPU (net protein utilization) - based on the ratio of amino acids retained vs. supplied Dietary proteins differ in their essential amino acid content. There are two ways to assess dietary protein quality. The first is by chemical score. Chemical score (amino acid score) is based on amino acid composition and is the ratio of the essential amino acids in particular foods to the reference diet (the whole-egg protein). NPU (net protein utilization), on the other hand, is an alternative method of evaluating protein quality by comparing the amount being retained in the body to the amount ingested. This value can be obtained by determining dietary protein intakes and then by measuring urea excretion. Protein Degradation-Dalton 3 Essential vs. nonessential amino acids Essential amino acids are those that a given organism cannot produce in sufficient quantities to meet its needs and must obtain from dietary sources. Nonessential amino acids are those that a given organism can produce in sufficient quantities to meet its needs for protein synthesis and to serve as metabolic precursors for hormones, for example. In humans, essential amino acids comprise about half of the twenty common amino acids found in proteins. Why can’t why? these amino acids be produced? In some cases, the pathway does not exist (we are unable to synthesize the corresponding a-keto acids), and in others, the amount produced is insufficient. Thus, we must obtain these essential amino acids from the diet. Requirements for amino acids are different for adults and children! Cysteine and arginine are essential for kids but not for adults: they can be synthesized from methionine and ornithine (urea cycle). The requirement for specific essential amino acids is different for adults and children. Cysteine and arginine are essential for children but not for adults (they can be synthesized from methionine and ornithine, respectively). Also, the requirement for essential amino acids is much higher in infants and children than in adults. Protein Degradation-Dalton 4 Serine is a nonessential amino acid that can be made from a glycolytic intermediate No intermediate No enzymes Nonessential amino acids are amino acids that we can produce in our body. For example, serine is a nonessential amino acid. The main pathway leading to the production of serine starts with the glycolytic intermediate 3-phosphoglycerate. 3-Phosphoglycerate is oxidized by a dehydrogenase to a keto acid, 3-phosphohydroxypyruvate, which is then transaminated to 3-phosphoserine. 3Phosphoserine is then converted to serine by phosphoserine phosphatase. Glycine, a nonessential amino acid, can be made from serine Glycine is another nonessential amino acid. The main pathway leading to glycine synthesis is a 1step reaction catalyzed by serine hydroxymethyltransferase. This reaction involves the transfer of the hydroxymethyl group from serine to the cofactor tetrahydrofolate (THF), producing glycine and N5,N10-methylene-THF. Glycine is involved in many anabolic reactions other than protein synthesis, including the synthesis of purine nucleotides, heme, glutathione, creatine, and serine. Protein Degradation-Dalton 5 c. Dietary protein digestion Dietary protein is a vital source of amino acids. Proteins ingested in the diet are digested in the small intestine by pancreatic proteases into amino acids and oligopeptides. Aminopeptidases associated with the intestinal epithelium further digest oligopeptides into amino acids and di- and tripeptides. Amino acids and di- and tripeptides are absorbed by intestinal mucosal cells via specific transporters. Within these cells, di- and tripeptides are converted to amino acids by peptidases. Free amino acids are then released into the blood for use by other tissues. The primary use of amino acids is as building blocks for protein synthesis and as a source of nitrogen for the synthesis of other amino acids and other nitrogenous compounds, such as nucleotide bases. Excess amino acids cannot be stored or excreted: surplus amino acids are used as metabolic fuel. Degradation of dietary proteins into amino acids by intestinal proteases 1 2 3 III. Recycling of intracellular proteins a. Purpose of intracellular protein degradation Biochemistry 7th Ed. ã 2012 W.H. Freeman and Company 1 •Removal of damaged proteins 2 •Energy production during fasting 3 •Regulation of biological/metabolic processes Intracellular protein degradation plays an important role in the removal of defective or damaged cellular proteins, the generation of energy during fasting (via degradation of muscle proteins to produce glucogenic amino acids needed for gluconeogenesis), and regulation of various biological processes. All proteins have a half-life that is determined based on intrinsic and environmental factors. The half-life of a protein is the time at which 50% of a simultaneously synthesized protein pool will be degraded. It determines the ’stability’ of the protein. Each protein has a unique half-life. Some are short (5-30 minutes) and some are long (hours-days, maybe even years). The half-life of each protein has evolved based on its function and regulation. Protein Degradation-Dalton 6 How can degrading proteins regulate biological and metabolic processes? i.e. change of cell fate; differentiation or cell cycle progression 1. Differentiation 2. Cell cycle 3. Maintain responsiveness i.e. Maintain responsiveness of cells to various biological signals Many biological scenarios use protein degradation There are many biological scenarios that benefit from controlled degradation of proteins. Here are a few examples to think about. If a cell is undergoing major changes in cell fate, it is logical that it would require significant changes to its proteomic composition to perform new functions and adapt structurally. This could be true during differentiation, the cell cycle or to support circadian rhythms. Alternatively, protein degradation may keep cells responsive to important signaling pathways. Signaling pathways typically result in transcription of target genes. This transcription will of course result in a build up of that target protein to perform the required function dictated by the change in the cell’s environment (the stimulus). If the target protein is not degraded in a reasonable time, the cell may not be able to sense future signaling events and transcriptional activation because the target protein is already abundant. Protein turnover and degradation allows the cell to maintain sensitivity to cellular signals. Protein Degradation-Dalton 7 Many biological processes are regulated by protein degradation Biochemistry 7th Ed. ã 2012 W.H. Freeman and Company * Many biological processes are controlled, in part, by protein degradation. The proteins being degraded are normally regulatory proteins. This allows the execution of a particular biological process in a timely fashion. Regulation of HMG-CoA reductase degradation The rate of degradation of HMG-CoA reductase is regulated by cellular cholesterol levels. The ubiquitin-proteasome pathway is involved. High cellular cholesterol • HMG CoA reductase is degraded faster. Cholesterol synthesis decreases Low cellular cholesterol • HMG CoA reductase is more stable. Cholesterol synthesis continues An example of how protein degradation controls a biological process is exemplified in the synthesis of cholesterol (Block 3). When cellular cholesterol levels rise, degradation of HMG-CoA reductase is facilitated to reduce cholesterol synthesis. Conversely, when cellular cholesterol levels are low, the rate of HMG-CoA reductase degradation is reduced to enable the continued production of cholesterol. Protein Degradation-Dalton 8 Intracellular proteins are degraded via 2 pathways: 1. Ubiquitin-proteasome pathway 2. Lysosomal pathway Intracellular proteins are degraded via two pathways: an ATP-dependent ubiquitin-proteasome pathway and the lysosomal pathway. The first pathway involves the conjugation of target proteins with ubiquitin and the subsequent degradation of the conjugated proteins by proteasomes. On the other hand, the second pathway involves the degradation of cellular proteins by lysosomal hydrolases. b. Ubiquitin-proteasome pathway i. Ubiquitin conjugation Ubiquitin tags cellular proteins for degradation Targeting of proteins to a proteasome requires ubiquitin, a highly conserved 76-amino acid protein. Ubiquitin is a tag that marks proteins for destruction. It has reactive lysine residues that are essential for the ubiquitination of target proteins. Lysine-48 is one of several lysine residues within ubiquitin that can be reactive, but we will primarily focus on lysine-48 here. Gly 76 Biochemistry 7th Ed. ã 2012 W.H. Freeman and Company Gly 76 Ubiquitin forms an isopeptide bond with proteins targeted for degradation The carboxyl terminal glycine (Gly 76) of ubiquitin can be covalently linked by an isopeptide bond to the e-amino group of a specific lysine residue on a protein that is destined for degradation. Biochemistry 7th Ed. ã 2012 W.H. Freeman and Company Protein Degradation-Dalton 9 Formation of ubiquitin-protein complex involves three steps (1) (2) E1 Ubiquitin-activating enzyme E2 Ubiquitin-conjugating enzyme E3 Ubiquitin-protein ligase (3) Biochemistry 7th Ed. ã 2012 W.H. Freeman and Company The conjugation of ubiquitin to a target protein involves a 3-step cascade pathway. (1) Ubiquitin is first activated by adenylation with ATP via E1 (ubiquitin-activating enzyme). Adenylated ubiquitin is then bound to a cysteine residue in E1. (2) Ubiquitin is then transferred to a cysteine residue in E2 (ubiquitin-conjugating enzyme). (3) E3 (ubiquitin-protein ligase) then transfers ubiquitin from E2 to a lysine residue in the target protein. Polyubiquitination of protein is required for degradation Gly 76 Gly 76 Gly 76 Lys 48 Lys 48 Biochemistry 7th Ed. ã 2012 W.H. Freeman and Company The attachment of a single molecule of ubiquitin is a weak signal for degradation. A stronger signal can be generated by the polyubiquitination of the target protein. A chain of ubiquitin can be generated through the formation of an isopeptide bond between the e-amino group of lysine-48 from one ubiquitin molecule with the carboxyl terminal glycine residue (Gly 76) from another ubiquitin molecule. Protein Degradation-Dalton 10 Fates of ubiquitinated protein E2 Endocytosis Transport Degradation Signaling Cell Div 5, 19 (2010). https://doi.org/10.1186/1747-1028-5-19 There are numerous linkage possibilities for ubiquitin tagging of proteins. Mono-ubiquitination tends to lead to functional changes while poly-ubiquitination using K48 linkages within the ubiquitin protein signals degradation by the proteasome. ii. Proteasomal degradation Proteasome degrades ubiquitin-tagged proteins a b Cap b Barrel a Cap Biochemistry 7th Ed. ã 2012 W.H. Freeman and Company A human cell contains about 30,000 proteasomes. A proteasome is a proteolytic complex made up of 28 polypeptides. These barrel-shaped structures can break down practically all proteins to peptides of 7-9 amino acids in length. The active surface of the proteasome is within the barrel where it is shielded from the rest of the cell. Access to the proteasomal interior is controlled by a regulatory cap complex. This cap complex binds the ubiquitin chain and allows the incoming protein to enter and get degraded by the proteasome. Video references: • https://www.youtube.com/watch?v=hvNJ3yWZQbE • https://www.youtube.com/watch?v=2ubaTVoQ6oU • https://youtu.be/ILdEOXCfgUc (Minute 14-18 for proteasome degradation) Protein Degradation-Dalton 11 Proteasome inhibitors in cancer therapy Proteasome Proteasome inhibitor Protein marked for degradation Ubiquitins Millenium Pharmaceuticals, Inc. Drugs inhibiting proteasomes cause cancer cell death by preventing the breakdown of certain proteins known to hinder cancer cell growth or by overloading the cell with conflicting regulatory molecules that send misleading signals. VELCADE (bortezomib) is the first therapeutic proteasome inhibitor Multiple myeloma VELCADE (bortezomib) is the first therapeutic proteasome inhibitor. It is approved in the US for the treatment of patients with relapsed multiple myeloma (a cancer of the plasma cells) and mantle cell lymphoma (a cancer of lymph nodes). This drug is an N-protected dipeptide with a boronic acid instead of a carboxylic acid on one end. The boron atom binds to the catalytic site of the proteasome. Deubiquitinating enzymes (DUBs) are another type of ubiquitin regulatory enzyme which exist to remove ubiquitin from target proteins. Inhibiting DUBs that target oncogenes is another avenue of therapeutic potential currently being explored. Inhibition of a DUB that targets a vital oncogene would increase degradation of that oncogenic protein and hopefully limit cancer progression. Many proteins within the ubiquitin conjugating pathway are implicated in disease. A few examples are BRCA1 (E3) in breast cancer, VHL (E3) in kidney cancer and Parkin (E3) in ALS and IBMPFD. Protein Degradation-Dalton 12 c. Lysosomal pathway Lysosomal pathway of protein degradation J. Cell Biol., 2012 Vol. 199, pg. 725 A lysosome is a membrane-bound organelle found in animal cells. They contain hydrolases that can degrade various biomolecules including lipids (e.g., sphingolipids and cholesterol ester), carbohydrates (i.e., glycogen), nucleic acids, and proteins. Lysosomes can digest obsolete or unused biomolecules in the cytoplasm that come from both inside and outside of cells. The above figure shows four distinct processes involving in the degradation of cellular materials by lysosomes. Lysosomes are involved in autophagy by fusing with autophagosomes containing intracellular components such as denatured proteins, lipids, carbohydrates, and old/damaged organelles (A). They can fuse with late endosomes and degrade the encapsulated contents (B). A good example of this is the degradation of LDL particles internalized by endocytosis (Block 3). Lysosomes can also carry out pinocytosis of cytosolic regions surrounding lysosomes (C). Lastly, lysosomes can degrade selective target proteins delivered to them by heat-shock protein Hsc-70 (D). Lysosomes have a luminal pH of approximately 4.5-5. Many lysosomal degradative enzymes are pH sensitive and they function optimally under this condition. Cathepsins are the major class of proteases found in lysosomes and they operate under low pH. These proteases can degrade the membrane encapsulated protein contents into amino acids, which can then exit lysosomes and join the intracellular amino acid pool. Protein Degradation-Dalton 13 IV. Summary • Degradation of dietary proteins and recycling of cellular proteins represent sources of amino acids. • Chemical score and NPU can be used to determine protein quality in foods. • Nonessential amino acids are amino acids whose precursors we can synthesize in our body. • Intracellular protein degradation is necessary for the removal of defective or damaged proteins, generation of energy during fasting, and regulation of various biological processes. • There are two pathways of intracellular protein degradation: ubiquitin-proteasome pathway and lysosomal pathway. • Prior to degradation by proteasomes, intracellular proteins are tagged with ubiquitin. • The lysosomal pathway uses cathepsins to digest obsolete or unused proteins found in the cytoplasm. Protein Degradation-Dalton 14