Glycolysis Biochemistry II (2022) PDF
Document Details
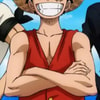
Uploaded by SmartScandium
Jordan University of Science and Technology
2022
Mera Masalmeh
Tags
Summary
These are notes on metabolism and biochemistry, specifically glycolysis. The document describes the process of glycolysis through a series of steps, mentioning various enzymes and molecules involved in the process. It also touches upon the different types of regulation regarding glycolysis.
Full Transcript
12 Mera Masalmeh Farah Saleh Diala Abu-Hassan Glycolysis Communication between Cells through Receptors GPCR #GPCR= G protein-coupled receptor of plasma membrane. - the most common type of receptor in our bodies is the G protein-coupled receptor (GPCR), GPCRs are a diverse family of cell surface rece...
12 Mera Masalmeh Farah Saleh Diala Abu-Hassan Glycolysis Communication between Cells through Receptors GPCR #GPCR= G protein-coupled receptor of plasma membrane. - the most common type of receptor in our bodies is the G protein-coupled receptor (GPCR), GPCRs are a diverse family of cell surface receptors involved in transmitting signals from outside the cell to the inside, they play crucial roles in various physiological processes such as neurotransmission, hormone signaling, and sensory perception. - GPCRs characterized by their structure, they are composed of 7 transmembrane helices that are shared by different types of GPCRs but they have different abilities of binding. - These helices form a transmembrane domain responsible for recognizing and binding specific signal molecules, such as neurotransmitters and hormones. - Despite their structural similarities, different types of GPCRs have distinct binding capacities due to variations in their amino acid sequences and functional domains. This diversity allows GPCRs to interact with a wide range of ligands, leading to specific cellular responses and signaling pathways. → How it is activated?? 1) Ligand Binding: - The activation process begins when a specific ligand (such as a neurotransmitter, hormone, or drug) binds to the extracellular domain of the GPCR. - This ligand binding induces a conformational change in the receptor, causing it to transition from an inactive state to an active state. 2) G Protein Activation: - The conformational change in the activated GPCR allows it to interact with and activate a G protein, which is a complex composed of three subunits: α, β, and γ. - When activated, the GPCR acts as a guanine nucleotide exchange factor (GEF), facilitating the exchange of GDP for GTP on the α subunit of the G protein. - Upon GTP binding, the α subunit of the G protein dissociates from the β and γ subunits. - Both the GTP-bound α subunit (active) and the βγ subunit complex (also active) can now interact with downstream effector molecules to initiate signaling cascades. 3) Activation of Effector Molecules and Cellular Response: - the α subunit activate adenylyl cyclase (membrane protein) to produce cyclic AMP (cAMP)- as a second messenger by convert ATP to PPi and cAMP while the βγ subunit complex may directly modulate ion channel activity. 4) Termination of Signaling: - The GPCR signaling cascade is terminated through several mechanisms, including the hydrolysis of GTP bound to the α subunit by GTPase activity. - GTP hydrolysis converts the α subunit back to its inactive GDPbound state, allowing it to reassociate with the βγ subunits and the GPCR, thereby terminating the signaling cascade. - cAMP binds to protein kinase A ▪ cAMP journey: Before we start, you should know that protein kinase A (PKA) have 4 subunits: - 2 regulatory, responsible to activate or inactivate (R) - 2 catalytic subunits (C) Remember: - Kinase: add phosphate group - Phosphatase: remove phosphate group 1) The G protein activates adenylyl cyclase, an enzyme that converts ATP (adenosine triphosphate) into cAMP (cyclic adenosine monophosphate). 2) cAMP is a second messenger that diffuses freely within the cell and activates downstream signaling molecules. 3) cAMP binds to the regulatory (R) subunits of PKA (2 cAMP for each R) 4) Binding of cAMP induces a conformational change in the PKA regulatory subunits, causing them to release the catalytic subunits (Active sites exposed). 5) The catalytic subunits of PKA are now active and capable of phosphorylating target proteins. 6) Active PKA catalytic subunits phosphorylate specific target proteins by transferring phosphate groups from ATP on the target proteins. 7) This phosphorylation modulates the activity, localization, or stability of the target proteins, leading to changes in cellular processes such as Activated enzymes, Inhibited Enzymes, Cell’s ion channels, bind to promoter and activation of transcription factors (gene expression) 8) The PKA signaling pathway is tightly regulated to ensure proper cellular responses. Phosphodiesterases (PDEs) degrade cAMP, terminating the signaling cascade. 9) Additionally, phosphatases can dephosphorylate and inactivate phosphorylated proteins, contributing to the regulation of cellular responses. ▪ Some general information related to glycolysis: ()عشوائيات - Glycolysis is a linear pathway - The product of the previous step is a reactant for the subsequent step. - Breakdown of glucose into pyruvate. - Universal pathway in all cell types Even in RBCs because it occurs in cytosol not in the mitochondria. - With or without O2 Which means it is not a requires O2. In pathways, irreversible steps usually have more regulatory processes than reversible steps. The rate-limiting step, which is the slowest and irreversible, controls the speed of the pathway. - It composed of 10 steps: Seven of them are reversible and three are irreversible. - This pathway for glucose is catabolic, but for other molecules it is anabolic because some intermediate within the glycolytic pathway can be used as processing to synthesize other molecules (Biosynthesis precursors). - Generation of ATP, we'll explain that in a moment. - After meals glucose level in the blood get high, so the insulin is secreted to increase the movement of these sugar from the bloodstream to the cell and the transporter which are manually GLUTs. The Two Phases of the glycolytic Pathway: Types of Glycolytic Reactions: - Phosphoryl transfer - Phosphoryl shift - Isomerization - Dehydration - Cleavage -Oxidation reduction Steps of Glycolysis Step 1: ▪ Glucose Phosphorylation: - Glucose is phosphorylated to glucose-6-phosphate by hexokinase (mainly) or glucokinase. - This step requires ATP and traps glucose inside the cell. - Irreversible step → The difference between hexokinase and glucokinase: Some references call it hexokinase IV Need higher insulin Remember: - Higher Km lower affinity - Km is the substrate concentration needed to reach half the maximum velocity. Step 2: Rearrange of bonds and electrons ▪ Isomerization: We will add phosphate group to make the two halves identical - Glucose-6-phosphate is converted to fructose-6-phosphate by phosphoglucose isomerase. - This is an isomerization reaction that prepares the molecule for further metabolism. - It is a Reversible step Step 3: Note: - Irreversible → we use different enzyme to convert the rxn - Reversible → the same enzyme is used to convert the rxn ▪ Second Phosphorylation: - Fructose-6-phosphate is phosphorylated to fructose-1,6-bisphosphate by phosphofructokinase-1 (PFK-1). - This step also requires ATP and is a key regulatory step in glycolysis. - it is an Irreversible step Step 4: It can’t continue with the remaining steps so we need isomerization (next step) ▪ Cleavage: - Fructose-1,6-bisphosphate is cleaved into two three-carbon molecules: dihydroxyacetone phosphate (DHAP) and glyceraldehyde-3phosphate (GAP) by aldolase. - Catalyzed by aldolase - Reversible step It can continue with the remaining steps. Step 5: ▪ Isomerization: - DHAP is converted to GAP by triose phosphate isomerase, ensuring that both molecules are in the same form. - This results in two molecules of GAP. - Reversible step → According to equilibrium it prefers going forward Step 6: ▪ Oxidative -reduction reaction: High energy bond It will be oxidized It will be reduced - GAP is oxidized to 1,3bisphosphoglycerate (1,3BPG) by glyceraldehyde-3phosphate dehydrogenase (GAPDH). - This reaction also generates NADH, capturing energy in the form of reducing equivalents. Is an inorganic phosphate (Pi). There is a phosphorylation in this step, but the source of Pi is not ATP Rather, it is free phosphate → Why don’t we use ATP here? Because we don’t need it :) (ΔG is negative) - Reversible step Step 7: Substrate-Level Phosphorylation: - 1,3-BPG is converted to 3-phosphoglycerate (3-PG) by glycerate kinase (GK), generating ATP via substrate-level phosphorylation. - This step directly produces ATP from ADP. - Reversible step Step (8-10): We have to change its position, if we remove it when it’s in C #3 we won’t get pyruvate at the end STEP 10 STEP 9 STEP 8 ▪ Isomerization-step8: Mutase: Isomerase specifically transfer phosphate group - 3-PG is isomerized to 2-phosphoglycerate (2-PG) by phosphoglycerate mutase. - P on carbon 3 - Reversible step ▪ Dehydration-step9: - 2-PG loses water to form phosphoenolpyruvate (PEP) catalyzed by enolase. - P on carbon 2 - Reversible step - Dehydration reaction ▪ Substrate-Level Phosphorylation and Formation of Pyruvate-step10: - PEP is converted to pyruvate by pyruvate kinase (PK), generating ATP via substrate-level phosphorylation. - This final step produces ATP and pyruvate, a crucial intermediate in various metabolic pathways. - The last irreversible step and the last step in the pathways → watch this video: https://youtu.be/YIw6ctErDHY?si=KHPI-zsePrCkt28N Summary : THE END OF SHEET #12 َ ،"يا ابن آدم "سقطتَ من بطن أ ُ ِ ِّمك َ َدم عُ ُم ِركَ منذ ِ وإنك لم تزل في ه، فإنها عن قليل قَب ُْرك،ط ِأ األرضَ بقدمك 13 Abdullah Al-Rawashdeh Dua’ Al-Shrouf Diala Abu Hassan Glycolysis Synthesis of 2,3-bisphophoglycerate in RBCs ▪ This process doesn’t need oxygen and it occurs in the cytoplasm so even RBCs (that don’t have mitochondria in them) mainly depends on glycolysis to synthesize its energy, although glycolysis creates little energy compared to aerobic but it’s enough for the RBC because it has one function. ▪ Sometimes in the step where 1,3-bisphophoglycerate is converted into 3-phosphoglyecrate, an RBC that reached the site for gas exchange (peripheral tissues where it needs to release the oxygen attached to it ) another pathway is used, instead of one direct conversion it becomes a two steps pathway using 2 enzymes (same product) ▪ In the first step the mutase enzyme transport the phosphate from C1 to C2 and the product is 2,3-bisphosphoglycerate, then by using an H2O molecule the phosphatase enzyme removes the phosphate on C2 producing the same product (3Phosphoglycerate). It releases it as an inorganic phosphate instead of the usual ATP. This pathway (glycolysis in this case) net ATP is zero (2ATP are used and 2 are produced) but we must ask why a cell would waste 2 ATP molecules to synthesize 2,3-bisphosphoglycerate? ▪ The molecule 2,3-BPG is important because it binds to hemoglobin after it releases its oxygens (T state), to reduce the affinity of the hemoglobin to rebind oxygen (since the oxygen is a gas it diffuses but it can rebind, so it alternates the hemoglobin btw the R and T states so to prevent this, this molecule binds to the hemoglobin) Energy Need and Production ▪ We can see each step and it’s energy usage or Production, the net ATP is 2, we don’t consider the NADHs as a part of the energy production because they are produced in the cytoplasm and they may not enter the mitochondria because they don’t have a carrier in the membrane so we cannot guarantee that they are going to be used in the ETC and participate in energy production ▪ In glycolysis NADH is no energy molecule, but it’s an electron carrying molecule, so it has nothing to do with creating energy in anaerobic -ATP -ATP 2NADH 2ATP 2ATP respiration (so the main role of NADH is to carry electrons in redox rxns even though they have the high energy phosphate bond but sometimes I can extract energy from them) Pyruvate fate ▪ We know that pyruvate loses one carbon and becomes acetyl CoA to enter krebs cycle ▪ But it could gain CO2 and become oxaloacetate to either supply TCA cycle or to create amino acids (aspartate). ▪ Under anaerobic conditions it can be converted to lactate. ▪ In non-human cells it can also be converted into acetaldehyde by decarboxylation and then into ethanol. ▪ The conversion of pyruvate to ethanol reaction happens in the yeast, so the decarboxylation of pyruvate removes a CO2 and it becomes acetaldehyde, then it will be reduced to ethanol by alcohol dehydrogenase (NADH is oxidized), so when we use the yeast for baking we put some sugar and hot water with it so it feeds on these sugars and glycolysis will take place, which results in the production of pyruvate and this process happens, and because CO2 is a gas it will try to diffuse and move away which makes the food fluffy. ▪ In anaerobic conditions the pyruvate is reduced to lactate by accepting a proton(a carbonyl -ketone- is converted to a hydroxyl group -secondary alcohol-) using NADH as a coenzyme and the enzyme lactate dehydrogenase, this enzyme can reconvert lactate to pyruvate so it works in both directions based on equilibrium and concentrations, it oxidizes NADH in the forward rxn but reduces it in the backward rxn, the NADH and NAD+ are the same but it alternates between these two states (they supply the same pool) but there’s a ratio between these two states depending on the oxidative status. ▪ The point of the forward being is to regenerate the NAD+ to use it again in glycolysis under anaerobic conditions. And remember this is a reversible reaction. When is lactate produced? 1)To cope with increased energy demands like in a rigorously muscles activation (exercising), when a muscle is being activated for too long the amount of oxygen reaching it will become less and less, that causes the cells to rely on anaerobic respiration for energy instead of aerobic even though it produces a much less energy, so after a while lactate accumulates and results on fatigue(lactate level is increased 5 to 10 folds). 2) Low energy demanding cells like RBCs also contribute to lactate production because it doesn’t have a mitochondrion and depends on glycolysis (there’s no fatigue here it occurs only in exercising muscles because muscle cells need much higher energy, so they produce more lactate) 3) During hypoxia (wether it’s generalized or localized) to survive brief episodes of hypoxia, the lactate production also increases. ▪ Climbing high mountains causes generalized hypoxia, also during corona viruse there was a big problem in the respiratory system, so a low amount of oxygen entered the body and the whole body suffered from hypoxia ▪ The body will try to recover and survive by depending more and more on the anaerobic respiration, so more lactate production ▪ While localized hypoxia occurs in different types of thrombosis, which results in partial or complete loss of blood supply to a certain region in your body which reduces the amount of oxygen that reaches this region, this causes the death of the cells in this region especially if these cells are very active (heart, brain and stomach cells) which need a high amount of energy and cannot live without oxygen and blood supply for a long period of time Clinical Hint:Lactic Acidosis ▪ High amounts of lactic acid may cause lactic acidosis, and it contains a hydroxyl group and a carboxylic group which are acidic groups, and it leads to: 1)low pH of plasma, it may be caused by high production of lactate or low breaking down of it. 2)it is the most common cause of metabolic acidosis, because this lactate shouldn’t stay in the cells, the body can reduce its concentration by using it to reproduce pyruvate using lactate dehydrogenase, but if it stays it will cause metabolic acidosis by: ↑Production of lactic acid ↓Utilization of lactic acid Pyruvate + NADH ↔Lactate + NAD+ ▪ Most common cause of this acidosis: Impairment of oxidative metabolism due to collapse of circulatory system ▪ Other causes: - Impaired O2 transport (if someone has a blood disease that affects the structure of hemoglobin, and it interferes with its ability to transport oxygen) - Respiratory failure - Uncontrolled hemorrhage (losing a big amount of blood leads to the loss of the RBCs and the oxygen attached to them, so the transport of oxygen is reduced leading to more dependence on the anaerobic respiration) - Direct inhibition of oxidative phosphorylation (even if Krebs cycle is still working, I cannot produce energy so there will be more shifting towards the anaerobic respiration) - Hypoxia in any tissue (generalized or localized) - Alcohol intoxication (high NADH/ NAD+) (in people who drinks large quantities of alcohol, the metabolism of this alcohol increases the ratio of NADH/NAD+, so most of the available coenzyme in the previous rxn will be NADH, which results in the inhibition of the Krebs cycle because it needs NAD+, so even if we have enough concentrations of the reactant, product and enzyme the rxn won’t move forward, more shifting towards anaerobic respiration) -↓ gluconeogenesis (it’s the pathway in which glucose is produced from non carbohydrate precursors, this occurs in the case of long periods of fasting, oxaloacetate is used in this process so if it was activated at low levels this means there is less oxaloacetate and more pyruvate, so more production of lactate) -↓ pyruvate dehydrogenase (this enzyme converts the pyruvate to Acytel-CoA, so if this conversion decreases, we won’t be able to supply enough Acyrel-CoA to the Krebs cycle, so again the cells will depend more on the anaerobic respiration) -↓ TCA cycle activity (if any enzyme in the TCA cycle has a mutation or a problem it will be reduced, and the cells will depend more on anaerobic respiration) -↓ pyruvate carboxylase (this enzyme converts the pyruvate to oxaloacetate, so again less supple to the Krebs cycle or we can say that we will have more pyruvate that will undergo anaerobic respiration instead of aerobic because it is limited by the oxygen level and the availability of the coenzyme NAD+) Regulation of Glycolysis ▪ Regulation usually occurs at the irreversible steps; it happens on 3 steps. Regulators of PFK and PK ▪ On the left side of this picture, we can see the regulatory molecules of glycolysis, while we can see the regulators of gluconeogenesis on the right side (which is the opposite rxn of glycolysis) ▪ Glycolysis is regulated at the hexokinase, phosphofructo-kinase and pyruvate kinase steps ▪ The first regulation happens at the phosphofructokinase, where it can be activated by fructose 2,6-bisphosphofructose (not fructose 1,6bisphosphate which is the product of this enzyme) which is a regulatory molecule that acts as a switch because it activates the glycolysis and inhibits the gluconeogenesis to make sure that the two opposite pathways aren’t on simultaneously (because if they worked together it’ll be a waste of energy) ▪ We can also activate this enzyme using the AMP, because this molecule indicates a low energy state in the cells ▪ While the ATP is an inhibitor because it indicates a high energy state in the cells , so there’s no need to break down glucose ▪ The citrate also works as an inhibitor, which is an intermediate of the Krebs cycle so if we have a high concentration of citrate this indicates that the Krebs cycle is working enough so there’s production of energy so there’s no need to break down the glucose ▪ The proton inhibits this enzyme, because the proton is a byproduct of the ETC so if we have a high concentration of H+ this means we have a high concentration of ATP because the ETC is working ▪ We can also regulate glycolysis through the pyruvate kinase which is the last enzyme in this process, which is activated by fructose 1,6All of these regulators are allosteric phosphate which is ྀྀྀྀྀྀthe product of the regulators, so they have binding sites on previous regulated step, this is called feed forward because a product from one step the enzyme, so they either convert it to activates the enzyme of a step that’s far ahead the active or the inactive form in the cycle (this activator is produced in step no.3 and activates the enzyme of step no.10) ▪ This enzyme is inhibited by the ATP, because it indicates a high energy state in the cells, so there’s no need to break down glucose ▪ Alanine amino acid also inhibits this enzyme, because if you removed the amine group from its structure it will become pyruvate, so it’s a source of pyruvate, so if it’s in high concentration there’s no need to synthesize pyruvate out of this step Glucokinase and hexokinase activity ▪ On the X axis we can see the substrate concentration, while on the Y axis the enzyme activity is shown ▪ Notice how the curve of the hexokinase is all down compared to the glucokinase, so the Vmax of the hexokinase is much less than that of glucokinase ▪ The Vmax of the hexokinase can be reached at a very low concentration of glucose, whereas the Vmax of glucokinase needs a really high concentration of glucose to be reached ▪ The orange region represents the concentration of glucose at fasting conditions, it takes 2 hours for the levels of the sugar in the blood to go down to fasting blood sugar which is the orange region (in diabetic patients the sugar level doesn’t go down even if they’re fasting) ▪ The sugar level shouldn’t go lower than the orange zone because the person will lose consciousness and that’s because the brain is exclusively dependent on glucose as a source of energy, so while the brain is consuming glucose, we have to supply it with enough glucose instead of the consumed concentration ▪ Notice that at the orange concentration the hexokinase is at the Vmax even though we’re fasting, however the glucokinase is working at 20% or less of its total activity at fasting conditions Glucokinase regulation ▪ Glucokinase as a molecule is found in the nucleus when it’s inactive, the Glucokinase regulatory protein (GKRP) is attached to it, so they form a complex together that is present inside the nucleus, this attachment makes the glucokinase inactive and keeps it in the nucleus ▪ Once glucose levels go up after a meal, insulin is secreted, so more expression of GLUTs especially GLUT 4, and as a result more uptake of the glucose into the cells ▪ A high concentration of glucose inside the cells is going to activate the enzymes (hexokinase and glucokinase) ▪ This happens because the glucose activated the dissociation of glucokinase away from this regulatory protein, when the glucokinase dissociates it can be transported from the nucleus to the cytoplasm where it can act on the glucose ▪ If the fructose 6-phosphate (one of the intermediates) increases a lot, this indicates that we’re breaking down a lot of the glucose, Sequestration: you move the molecule so it’s going to activate the return of the away from where it can function glucokinase back to the nucleus, this is called sequestration, when it’s in the nucleus it’s going to bind to the regulatory protein again so it gets inactivated Regulation of ATP and AMP ▪ As we said ATP and AMP indicate different energy states, and their levels are different between rest and exercise (ATP levels are low at the exercise state because it’s being consumed because we need energy, whereas the ADP and AMP levels go up while exercising) End of sheet 13 14 Celine Alslaim Asma’a Abu-Qtaish Diala Abu-Hassan Regulation of glycolysis → Regulation of glycolysis occurs at three steps (the irreversible steps): 1. Step-1 (catalyzed by Glucokinase or Hexokinase) 2. Step-3 (catalyzed by phosphofructokinase 1) 3. Step-10 (catalyzed by pyruvate kinase) ▪ Second regulation step phosphofructokinase 1 (step-3 Glycolysis): - PFK 1 has multiple allosteric regulators, positive and negative, the positive ones are AMP & Fructose 2,6 bisphosphate. - This diagram shows the relationship between substrate conc. and velocity, the major substrate of this enzyme is F6p - The red curve represents the reaction without any activator or inhibitor, it is sigmoidal in shape, indicates we need to increase conc. of substrate to increase velocity (to make the curve steeper). - The blue curve represents the reaction when either added AMP or F2,6Bp as an activator, the curve shifted to the left and become Hyperbolic in shape. - Conc. of substrate at 1mM: Without activator → the velocity 10% of Vmax With activator → the velocity 50% of Vmax - Considering the same Reaction, looking at the curve of the second substrate which is ATP. - Again, the curve shows relation between (conc./ velocity) - At a low concentration of ATP, the curve going up steep (high increase in velocity) then it’s going down steep, why? Because ATP has a dual function in this reaction (substrate + negative regulator). low conc. of ATP → acts as a substrate and it increase the rate of the reaction really quickly High cons. of ATP → acts as an inhibitor (negative allosteric regulator) - When added AMP or F2,6Bp, the curve declines but at a slower rate, means ATP works as an inhibitor but with these activators the decline in rate is going to be slower than that in absence of these molecules. ▪ Last regulation step Pyruvate kinase (step-10 Glycolysis): → It’s regulated by multiple allosteric regulators: 1. Fructose1,6Bisphosphate: act as an activator and It’s produced by the irreversible step (step-3 glycolysis), this is a feed-forward regulation. 2. ATP: indicates a high energy state that’s why inhibits the pyruvate kinase activity 3. Alanine: an amino acid and a source of pyruvate, that’s why alanine inhibits glycolysis specifically at this step because it’s the step that produces pyruvate. - Pyruvate doesn’t differ if it comes from Glucose or Alanine or other amino acids, it’s all the same, so when pyruvate present in a high conc. there is no need to break down the Glucose to produce pyruvate. *The Regulators we talked about are allosteric regulators, they bind to certain sites on the enzyme surface to activate or inhibit its function. ▪ Hormonal Regulation → The two main hormones that regulate glycolysis are: 1. insulin: secreted in A. well-fed state B. high conc. of glucose in blood 2. Glucagon: secreted in A. fasting state B. low conc. of glucose in blood - Insulin acts as activator, because when insulin is high, glucose is high and as long as the body has high conc. of sugar it could be used as a source of energy, so it’ll be degraded in Glycolysis. - When the body has a low conc. of sugar, the use of sugar will be limited and we keep it just for priority, for that reason glucagon inhibits glycolysis. - Hormones bind to the cell surface receptors because they can’t enter the cell to reach the enzymes, so they affect indirectly through signaling pathways. - Insulin is bound to receptor tyrosine kinase and glucagon to GPCR on the cell surface, they would affect intracellular proteins and enzymes to activate or inhibit Glycolysis. Figure A in the next page represents well-fed state, Insulin levels increase and bind to its receptor, unlike glucagon that’s found in low levels, so there is no activation of some sequent pathways (pale color). ▪ Bifunctional Enzyme: - A target protein has two sides, one acts as a kinase (PFK-2) and the other acts as phosphatase (FBP-2), they don’t activate at the same time. Figure A - When the bifunctional enzyme dephosphorylates, the kinase part will be active, and the phosphatase will be inactive. - Phosphofructokinase-2 (PFK-2) convert F-6-P to F-2,6-BP which will activate PFK-1 - When Glucagon levels increase → Glucagon will bind to adenylate cyclase → convert ATP to cAMP → cAMP activate PkA → PkA phosphorylate bifunctional enzyme → PFK-2 (inactive) → FBP-2 (active) ▪ Hormonal Regulation of pyruvate kinase Another target protein of PkA, under fasting state there is high level of Glucagon → PkA (active) → phosphorylate pyruvate kinase (inactive) → inhibits Glycolysis. ▪ Clinical Hint: pyruvate kinase deficiency - Pyruvate kinase deficiency is the most common among glycolytic enzyme deficiencies Alterations observed with various mutant forms of pyruvate kinase - Pyruvate kinase (last step in glycolysis) converts phosphoenolpyruvate to pyruvate and produces ATP, so in the case of deficiency in pyruvate kinase we lose the two ATP molecules, the net energy of glycolysis will be Zero. - RBCs are the most common affected cells because they rely on glycolysis as a source of energy - RBCs utilize ATP to run Na+/K+, and by maintaining ion distribution across the membrane, that’s help in maintain the shape of the cell (biconcave), this shape helps in flexibility of the cell so the RBCs can pass through the blood capillaries. - Low ATP → premature death of RBC (short life span) because RBCs lose its fixable shape, that will lead to “mild to severe chronic hemolytic anemia”. - Abnormal enzyme, mostly altered kinetic properties. ❖ External Inhibitor of Glycolysis They are not present in your cells, but if you are exposed to them, they will affect Glycolysis, for example the inorganic inhibitors that we will discuss in this topic. ▪ Inorganic inhibitors of Glycolysis: 1. Fluoride Act on Glycolysis of bacterial cells, specifically inhibits enzyme Enolase (step-9), when inhibit this step, all Glycolysis inhibited → reduce providing energy for this bacteria → the bacteria ability to divide reduced → prevents Dental Caries. 2. Arsenic Poisoning A. Pentavalent Arsenic (Arsenate): Act on glycolysis, by act as a competitive inhibitor to inorganic phosphate as a substrate for GA3PDH, so Arsenate will affect the production of ATP in Glycolysis (decrease ATP) (GA3PDH) B. Trivalent Arsenic (Arsenite): More dangerous, because it doesn’t affect Glycolysis directly, it affects the reactions after Glycolysis (TCA cycle & pyruvate dehydrogenase complex (PDH)). - PDH complex contains multiple enzymes and use multiple Co-enzymes, one of them is lipoic acid, which has SH group, Arsenite do stable complex with SH group, and interferes with oxidation reduction of this group, reduce the activity of pyruvate dehydrogenase, no AcetyCoA, which will affect the supply of Acetyl-CoA to TCA cycle. - Also, Arsenite affect alfa ketoglutarate Dehydrogenase complex, which used in TCA cycle - Neurological disturbances may lead to DEATH ……………………………………………………………………………………………………………………………………………………………………… Gluconeogenesis - Gluconeogenesis is the opposite pathway of Glycolysis, it is a production of glucose from non-carbohydrate precursors. - In well-fed state → high blood glucose levels → insulin secreted → more uptake of sugar to the cells, the blood glucose levels drop after about two hours enter in fasting state. Gluconeogenesis: Gluco → Glucose Neo → New Genesis → Production/Formation In these 2 hours glucose goes to cells: - For glycogen synthesis (the storage of glucose) - For synthesis of glucuronic acid to make GAGs - For glycolysis - For pentose phosphate pathway - In fasting state → the body depends on the second source of glucose (GLYCOGEN), but glycogen doesn’t last for a long period of time (12h-18h), when glycogen consumed, we enter the GLUCONEOGENESIS. → Gluconeogenesis synthesis glucose for two reasons: 1. Provides glucose to tissues that exclusively depend on glucose as source of energy, like: brain, RBCs, renal medulla. o The brain is dependent on glucose 120g/day. NOTE: Hypoglycemia may lead to brain death if it last to long period of time. 2. To provide glucose to blood stream to maintain fasting blood sugar levels. o Maintaining blood sugar levels is very important for survival, as tissues that depend on it, such as the brain, will be damaged when the sugar level drops too low. - Glucose is a solute, when its concentration goes down, it’s affect (osmotic pressure → water movement → blood pressure), so glucose helps in homeostasis. - But the cons. of glucose is dynamic, that’s why we have to compensate the decrease by glycogen (in short term) and by Gluconeogenesis (in long term) Body glucose reserve is limited (less than 500g) ≈20g (extra cellular fluid mainly in GAGs) ≈70g (liver glycogen); enough for 16hours. ≈400g (muscle glycogen); for muscle use only. *Main source of energy for resting muscle in post-absorptive state. → Why do we store fats in high amounts, and not carbohydrates? 1. The amount of energy stored in fat is much more than in carbohydrates, so if we store energy in carbs, it leads to weight gain. - 1g of Carbs → 4 Kcal - 1g of Fats → 9 Kcal 2. Carbs are hydrophilic molecules, so if we stored it, it’s going to attract a high amount of water molecules with it, unlike fats, which are hydrophobic molecules, so they won’t attract water molecules. 70Kg man has≈ 15Kg fat (stored as triacylglycerol) - Fatty acids can’t be converted to glucose. - Utilization of FA is increased 4-5 X in prolonged fasting. - In prolonged fasting; FA → ketone bodies at high rate. - In fasting state, the dominating hormone is Glucagon which has many target cells, one of them adipocytes. - We stored lipids in adipocytes as Triacylglycerol, so by the hormonal signal (Glucagon) → activation of enzymes that hydrolysis the bond between FA and Glycerol → Free FA → transported from adipocyte to blood stream to cells → breakdown and use as a source of energy. - FA synthesis ATP molecules more than Glucose, and it also synthesis acetate which enters TCA cycle (too long process). Conversely, Glycogen is present in the cells, just breakdown glucose and enter it in Glycolysis, that’s why athletes in marathon prefer to store Glycogen, because lipolysis is longer. َ َ ّ ّ ّ ّ وأهلكت َ إال وقد وعدوهم عدونا نَصتهم ينقض رمضان ال رب يا ي ال تنسوا أهلنا يف غزة من دعائكم THE END OF SHEET #14 GOOD LUCK TEEJAN :)