Transmembrane Transport of Ions and Small Molecules - PDF
Document Details
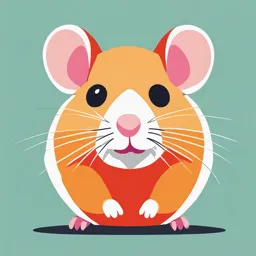
Uploaded by AffluentNovaculite2115
The University of Texas at Rio Grande Valley
Tobias Weinrich, PhD
Tags
Summary
These lecture notes cover transmembrane transport of ions and small molecules, differentiating between passive and active transport mechanisms like diffusion, facilitated diffusion, and various pumps. The material also delves into the specifics of ion channels, transporters, and membrane potential. It's intended for an undergraduate-level biology course.
Full Transcript
1 TRANSMEMBRANE TRANSPORT OF IONS AND SMALL MOLECULES 10/6/2024 Tobias Weinrich, PhD School of Integrative Biological and Chemical Sciences 2 Student Learning Outcomes ▪ Distinguish molecules that can diffuse thro...
1 TRANSMEMBRANE TRANSPORT OF IONS AND SMALL MOLECULES 10/6/2024 Tobias Weinrich, PhD School of Integrative Biological and Chemical Sciences 2 Student Learning Outcomes ▪ Distinguish molecules that can diffuse through a lipid bilayer from those that require transporters to cross a membrane. ▪ Explain the mechanism by which cells maintain the concentration differences of small molecules across biological membranes. ▪ Compare and contrast passive vs active transport (and between primary and secondary active transport). ▪ Compare and contrast different kinds of channels (including gated channels), transporters/carriers, and pumps. ▪ Explain the role of membrane potential and ion channels in action potentials. 3 Lecture Structure 1. Selective permeable membrane 1.A. Concentration and electrochemical gradients 1.B. Transport Mechanisms 2. Passive transport 2.1. (Diffusion) 2.2. Facilitated a) Transporters/carriers b) Channels & 3. Active transport 3.1. Primary Active Transport 3.2. Secondary Active Transport 4. Action Potential and synapse 4 1. Selective permeable membrane ▪ Impermeable to hydrophilic molecules ▪ Large, polar molecules ▪ Ions ▪ Permeable (simple diffusion) – small, non-polar molecules (gases) ▪ Slightly permeable – water Selective transport: ▪ Determines cytoplasm composition ▪ Transport rate: ▪ Differences in charge ▪ Differences in concentration 5 1.A. Concentration gradients vs electrochemical gradient Gradients = potential energy (stored energy) ▪ Concentration gradient – different concentration in two areas ▪ Determines direction of movement of non-charged molecules ▪ Electrochemical gradient – charge + concentration gradient Na+ ▪ Determines direction of movement of charged molecules ▪ Membrane potential voltage difference across the membrane (imbalance of electrical net charges) *Arrow thickness represents rate of transport 6 1.B. Transport mechanisms PASSIVE No energy ▪ Direction of transport → down concentration or electrochemical gradient requirement a) Simple diffusion b) Facilitated ▪ Channel – hydrophilic pore ▪ Transporter/Carrier mediated – binds solute ACTIVE Energy ▪ Direction of transport → against concentration or electrochemical gradient requirement a) Primary – ATP hydrolysis b) Secondary 7 2.1. Passive Transport – Simple Diffusion ▪ No membrane protein required for transport Permeable Impermeable Molecules: ▪ Small nonpolar – CO2, O2 ▪ Small polar molecules – ethanol, water ▪ Large nonpolar – oils, steroids Diffusion rate: ▪ Permeability coefficient ▪ Difference in concentration gradient Rate of diffusion [Solute to be transported] 8 2.2. Passive Transport – Facilitated Diffusion ▪ Multi-pass membrane protein required Protein: for transport ▪ Channels – hydrophilic pore Molecules: ▪ Charged ions – K+, H+, Cl-, Ca2+, Na+ ▪ Polar molecules – glucose, amino acids ▪ Transporters/carriers – solute binds to ▪ Charged ion – K+, H+, Cl-, Na+ transporter → conformational change → solute released on the other side Hydrophilic pore 9 2.2. Passive Transport – Facilitated Diffusion A. TRANSPORTERS AND CARRIERS Specificity: each transporter/carriers transport one molecule ▪ Specificity of carrier protein determined by amino acid sequence ▪ Passive – uniport ▪ Active – symport and cotransport Transport mechanisms – alternating conformational model ▪ Solute binds to transporter → conformational change → solute released on the other side ▪ One molecule at a time ▪ Direction of transport determined by concentration gradient 10 2.2. Passive Transport – Facilitated Diffusion A. TRANSPORTERS AND CARRIERS Saturation (all carrier proteins Transportation rate: are occupied) Rate of diffusion ▪ Concentration gradient Vmax ▪ Affinity of the carrier protein for the solute ▪ Carrier protein availability ▪ Only ‘so many’ molecules can be transported [Solute to be transported] at any given time Example – Glucose absorption by erythrocytes P Glucose-6-phosphate 11 2.2. Passive Transport – Facilitated Diffusion B. ION CHANNELS ▪ Multi-pass channel membrane protein required for transport Specificity: Na+ channel ▪ Central region of channel – selectivity filter ▪ Discriminate based on charge and size Transport mechanism: ▪ Hydrophilic pore that allows passing of ions ▪ Direction of transport determined by electrochemical gradient + K channel 12 2.2. Passive Transport – Facilitated Diffusion Vmax B. ION CHANNELS Types: Saturation Rate of diffusion (all channel ▪ Non-gate controlled (K+ leak channel) proteins are open) ▪ Gated (open and closed conformation) ▪ Voltage-gated [Solute to be transported] ▪ Ligand-gated ▪ Mechanically gated Transportation rate: ▪ Electrochemical gradient ▪ Ion channel conductivity – how fast can ions pass through the channel ▪ Open conformation of channel 13 2.2. Passive Transport – Facilitated Diffusion B. ION CHANNELS ▪ Voltage-gated channel - open and close in response to changes in the membrane potential ▪ Refractory period – period of time that prevent re-opening of channels ▪ Guarantees directionality of signal propagation ▪ Example: sodium channels in action potential – channel opens when membrane depolarizes 14 2.2. Passive Transport – Facilitated Diffusion B. ION CHANNELS ▪ Ligand-gated channel – ligand binding triggers opening of the channel ▪ Ligand is not transported! ▪ Intracellular ligand or extracellular ligand ▪ Example: nicotinic acetylcholine receptors ▪ Chemical signal → electrical signal 15 2.2. Passive Transport – Facilitated Diffusion B. ION CHANNELS ▪ Mechanically-gated channel - respond to mechanical forces that act on the membrane (e.g. waves) ▪ Example – auditory hair cells ▪ Silence – channels are closed ▪ Sound waves create vibrations that deflect the stereocilia on hair cells ▪ Open of mechanically-gated ions → depolarization and transmission of signal 16 3. Active transport Energy Direction of transport – against concentration or electrochemical gradient requirement 1. Primary active transport – maintain ionic gradients across membranes ▪ ATP powered pumps – ATP hydrolysis expense driven transport 2. Secondary active transport: ▪ Coupled transporters (transporter/carrier proteins) – symporter & antiporter ▪ Cotransport – gradient driven transport 3. Light driven pumps – bacteriorhodopsin Light driven pumps 17 3.1. Primary Active Transport - pumps ▪ Pumps – transmembrane proteins (some are multi subunit) + ATP hydrolysis P-type pump – H+, Na+, K+, Ca2+ pump ATP hydrolysis → phosphorylation of pump Location: ▪ Cell membrane of plants, fungi, bacteria (H+ pump) ▪ Cell membrane of higher eukaryotes (Na+/K+ pump) ▪ Cell membrane of eukaryotes (Ca2+ pump) ▪ Cell membrane of sarcoplasmic reticulum (Ca2+ pump) V-type proton pump – H+ pump Pump is not phosphorylated Location: ▪ Plasma membrane of osteoclasts and some kidney tubule cells ▪ Vacuolar membrane of plants and fungi ▪ Endosome/Lysosomes membranes 18 3.1. Primary Active Transport - pumps F-type ATPase (ATP synthase) – H+ pump Structure: ▪ F0 – transmembrane pore F0 ▪ F1 – ATP binding site Function: F1 ▪ Normal direction – H+ pump ▪ Reverse direction – ATP synthesis (ADP + Pi → ATP) Location: ▪ Cell membrane of bacteria ▪ Inner mitochondrial membrane (all eukaryotes) ▪ Thylakoid membranes (plants) 19 3.1. Primary Active Transport - pumps ABC transporter (ATP binding cassette) – ions and small molecules ▪ ABC protein is specific for a single substrate ▪ ATP binding and hydrolysis drives transport Structure: ▪ Two transmembrane domains ▪ Two cytosolic ATP-binding domains Location: ▪ Bacterial plasma membranes (amino acid, sugar, and peptide transporters) ▪ Mammalian plasma membranes (transporters of phospholipids, small lipophilic drugs, cholesterol, other small molecules) 20 3.1. Primary Active Transport - examples P-type ATPase – Na+/K+ Pump ▪ ATP hydrolysis ▪ 3x Na+ cytosol – extracellular ▪ 2x K+ extracellular – intracellular Function ▪ Generation and maintenance of resting membrane potential ▪ Na+ (higher extracellular) ▪ K+ (higher cytosol) ▪ Regulate osmotic pressure ▪ Secondary active transport 21 3.1. Primary Active Transport - examples P-type ATPase – Na+/K+ Pump ▪ Cyclic mode of operation of the Na+/K+ pump 22 3.1. Primary Active Transport - examples P-type ATPase – Ca2+ Pump ▪ ATP hydrolysis ▪ Import 2x Ca2+ from cytoplasm into lumen sarcoplasmic reticulum ▪ Sarcoplasmic reticulum (specialized ER in muscle cells) Function: ▪ Maintain intracellular Ca2+ levels at minimum ▪ Calcium – primarily involved in cell signaling 23 3.1. Primary Active Transport - examples P and V pumps – H+ pumps P-pump V-pump V-pump 24 3.1. Primary Active Transport - examples ABC-type – CFTR Cl- channel ▪ Cell membrane of epithelial cells (airways, intestines) Healthy individual ▪ CFTR exports chloride ions → followed by water ▪ Maintains hydrated mucus Cystic Fibrosis – disease caused by H2O dysfunctional CFTR ▪ Symptoms: reduced chloride ion transport ▪ This disrupts the balance of salt and water in various tissues CFTR ▪ Thick Mucus Production ▪ Recurrent respiratory infections CFTR – Cystic Fibrosis Transmembrane Conductance Regulator 25 3.2. Secondary Active Transport - cotransport ▪ Coupled transporters (transporter/carrier proteins) – symporter & antiporter ▪ Cotransport – gradient driven transport ▪ Animal cells – Na+ driven cotransport ▪ Na+/K+ pump generated Na+ gradient ▪ Plant cells – H+ driven cotransport ▪ H+ pump generates H+ gradient 26 3.2. Secondary Active Transport - examples Symport Na+-glucose ▪ Active transport of glucose coupled to Na+ electrochemical gradient 27 3.2. Secondary Active Transport - examples Co-transport examples Antiport Na+/Ca2+ Ca2+ Assists in maintaining Symport Ca2+ Glucose Na+/Glucose low intracellular Ca2+ 3 Na+ 2 Na+ Glucose Cytosol Na+ ADP+Pi 2 K+ H+ Antiport ATP Na+/H+ Na+/K+ ATPase (pump) Helps regulate H+ Na+ intracellular pH 3 Na+ 28 4. Action potential and synapse ▪ Each cell – own set of membrane transport ▪ Specific functions ▪ Multiple membrane proteins function together 29 4. Action potential and synapse MEMBRANE POTENTIAL ▪ Distribution of ions on either side of the lipid bilayer gives rise to the membrane potential ▪ K+ leak channels – maintain resting membrane potential (-60mV) 30 4. Action potential and synapse ▪ Messages are carried by action potentials ▪ Mediated by voltage-gated cation channels (Na+ and K+) 31 4. Action potential and synapse ACTION POTENTIAL ▪ Triggered by depolarization of nerve cell plasma membrane 32 4. Action potential and synapse ▪ Propagation of action potential 33 4. Action potential and synapse Nerve terminal - synapse ▪ Electrical signal is converted into a chemical signal ▪ Arriving action potential – Ca2+ voltage-gated channels – influx of Ca2+ stimulates exocytosis of synaptic vesicles (neurotransmitter) 34 Postsynaptic cell POSTSYNAPTIC CELL ▪ Neurotransmitter binds to a ligand-gated ion channel ▪ Chemical signal converted into electrical signal ▪ Example: neuromuscular junction ▪ Excited motor neuron releases acetylcholine → opens ligand-gated Na+ -> influx of Na+ → depolarization → voltage-gated Ca2+ channels open → Ca2+ in sarcoplasmic reticulum open too ▪ ↑↑↑↑↑Intracellular Ca2+ → muscle contraction