The Electrocardiogram 2024 PDF
Document Details
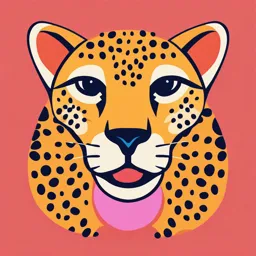
Uploaded by ProductiveRegionalism5444
University of Iowa
2024
Christopher J. Benson, M.D.
Tags
Related
Summary
This handout provides an introduction to the electrocardiogram (ECG), including its basis, learning objectives, key terms, waveforms, intervals, and a discussion of its clinical relevance. It is a lecture handout for a medical physiology course likely at the undergraduate level.
Full Transcript
THE ELECTROCARDIOGRAM 2024 Christopher J. Benson, M.D. Department of Internal Medicine, Division of Cardiovascular Medicine 3184 ML, UIHC; 335-8412; [email protected] Suggested Reading Assignment: Boron and Boulpaep, Medic...
THE ELECTROCARDIOGRAM 2024 Christopher J. Benson, M.D. Department of Internal Medicine, Division of Cardiovascular Medicine 3184 ML, UIHC; 335-8412; [email protected] Suggested Reading Assignment: Boron and Boulpaep, Medical Physiology, Chapter 21. Learning Objectives: 1. the basis of local electrograms, how they generate the specific waveforms of the ECG, and why waveforms appear different in different leads 2. the electrode conventions for standard ECG recording including electrode placement and standard calibrations of amplitude and paper speed 3. important wave forms, intervals, and their significance 4. determination of the mean QRS axis, normal range, and significance 5. you will learn to read ECG’s from a clinical standpoint later in your training! Key Terms: Electrogram Cardiac vector P wave Standard limb leads QRS complex Augmented limb leads T wave Hexaxial coordinate system ST segment Precordial leads PR interval Mean QRS axis QRS interval MOHD1 The Electrocardiogram page 1 Christopher J. Benson, M.D. I. ELECTROCARDIOGRAM (ECG or EKG): INTRODUCTION A. A series of indirect recordings of the average electrical activity of the heart (waves of action potentials) recorded from electrodes placed on the surface of different regions of the body. It provides a representation of the timing, direction, and magnitude of cardiac action potential propagation through the heart as projected onto 2 orthogonal planes. Figure 1 B. Provides information about: 1) anatomical orientation of the heart; 2) size of the chambers; 3) rhythm and conduction disturbances; 4) cardiac injury; and the 5) effects of drug or electrolyte disturbances C. Conventions of the electrocardiogram (Fig. 2) 1. time scale (horizontal): each large box (5 mm) = 0.2 sec; each small box (1 mm) = 0.04 sec 2. voltage scale (vertical): each large box (5 mm) = 0.5 mvolt; each small box (1 mm) = 0.1 mvolt Voltage (mV) Time (sec) D. Important waves, segments, and intervals (Figs. 2 and 3) Figure 2 MOHD1 The Electrocardiogram page 2 Christopher J. Benson, M.D. 1. P wave – atrial depolarization 2. QRS complex – ventricular depolarization 3. T wave – ventricular repolarization 4. ST segment - time between completion of ventricular depolarization and before ventricular repolarization; usually isoelectric (0 mV). During this period almost all ventricular myocytes are depolarized, and none have begun repolarization (phase 2 of the action potential – see Figure 1). 5. P-R interval (normal: 0.12 to 0.20 sec) - time from beginning of atrial depolarization to beginning of ventricular depolarization - most of this time is due to slow conduction through the AV node 6. QRS interval (normal: 0.07 to 0.12 sec) - total length of time necessary to depolarize the entire ventricular muscle; a good indication of whether the ventricle is activated normally through the His-Purkinje system 7. QT interval (normal: ~0.4 sec, but varies inversely with heart rate) – correlates closely with mean action potential duration of the ventricular myocytes E. Calculation of heart rate: rate (beats per minute) = 60 sec/min R-R interval (sec/beat) Figure 3. Relationship between action potentials and ECG intervals MOHD1 The Electrocardiogram page 3 Christopher J. Benson, M.D. II. BASIS OF ELECTROCARDIOGRAPHY A. Records extracellular voltage differences produced by waves of action potentials propagating through the heart 1. Figure 4A represents a piece cardiac tissue sitting in a bath. If the left side is electrically stimulated, a wave of depolarization (red line) will proceed from left to right. During this time, the right side of the bath surrounding the tissue is positively charged compared to the left side. 2. Figure 4B represents the same set up, except that now we have a recording electrode (E) on the right side and the stimulation electrode (S) on the left. In this situation a positive voltage potential (upward deflection) is recorded (the extracellular bath on the right is positively charged compared to the left). 3. In Figure 4C the polarity of the recording electrodes has been reversed, and a negative potential is recorded because the left side of the extracellular solution is relatively negative. 4. Figure 4D represents the effect of increasing the tissue mass. Note that the recorded potential is larger. 5. Figure 4E represents simultaneous depolarization of tissue of varying mass, and in opposite directions. This example simulates what happens when the larger left ventricle and smaller right ventricles are simultaneously depolarized. The recorded potential represents the sum of the two waves of depolarization. A Direction of depolarization depolarized At rest B C Depolarization toward electrode Depolarization away from electrode - - + D Depolarization of larger tissue E - + + - - + Figure 4 MOHD1 The Electrocardiogram page 4 Christopher J. Benson, M.D. III. VECTORS OF CARDIAC DEPOLARIZATION AND REPOLARIZATION A. Waves of depolarization Figure 5 reviews the sequence of depolarization through the heart (recall the timing of this sequence in Figure 1 of the first lecture handout). Atrial depolarization spreads from the SA node downward toward the AV node, and leftward to the left atrium. After a pause in the AV node, depolarization proceeds rapidly down the His-Purkinjie system through the septum, then the rest of the ventricular myocardium depolarizes from the endocardium to the epicardium. B. The electrocardiogram records voltage changes generated by extracellular currents The wave of depolarization generates flow of current outside the heart, which is what is measured with an ECG (Figure 6A). In the intact heart the wave of depolarization spreads throughout the tissue in many directions at once, however the instantaneous sum of these currents is represented by a vector (black arrow) having both direction and magnitude (Figure 6B). By convention, the cardiac vector points from the negative pole (activated tissue, relatively negative extracellular potential) to the positive pole (resting tissue, relatively positive extracellular potential) – i.e., the vector points in the direction of travel of the wave of depolarization. Figure 5 MOHD1 The Electrocardiogram page 5 Christopher J. Benson, M.D. A B Figure 6 C. The direction and magnitude of the resultant vector changes throughout the cardiac cycle as the electrical impulses travel through the various cardiac tissues. Figure 7A represents the mean QRS vectors at three time points in ventricular depolarization. A recording electrode “looking” up from the bottom-right of a two- dimensional plane (apex of the heart) would record the resultant QRS complex based upon the vectors (Figure 7B). At the early time point, the septum is depolarizing from left to right, resulting in a small vector pointing away from the electrode, thus a negative Q wave is recorded. At the middle time point, the bulk of the ventricle is depolarizing toward the apex, resulting in the large positive R wave. At the late time point, the posterior portion of the base is the last to depolarize, resulting in a small negative S wave. The panel on the far right of the figure represents the mean vector averaged throughout ventricular depolarization. Note that left and right ventricles are activated almost simultaneously, but the larger mass of the left ventricle dominates, and so the resultant mean vector points to the left. early QRS vector middle QRS vector late QRS vector mean QRS vector A B Interventricular R Septum S Q Figure 7 recordi MOHD1 The Electrocardiogram ng page 6 Christopher J. Benson, M.D. electrod e IV. RECORDING THE ELECTROCARDIOGRAM A. An indirect recording – individual electrodes attached to limbs and chest (the body acts as the bath). Consist of 3 sets of leads (standard limb leads, augmented limb leads, and precordial leads). B. Standard limb leads: I, II, and III; record in the frontal plane – bipolar recordings because voltage is measured as the difference between two electrodes (Fig. 8) Lead I Lead II Lead III Figure 8 1. Connections: by convention, the direction of the lead (yellow vector) is toward the positive electrode. a) Lead I – right arm (- electrode) versus left arm (+ electrode); b) Lead II – right arm (-) versus left foot (+); c) Lead III – left arm (-) versus left foot (+); 2. The axis’s of the limb leads are shifted 60 from each other. 3. EACH LEAD RECORDS THE MAGNITUDE OF THE VECTOR IN THE SPECIFIC DIRECTION OF THE LEAD'S INDIVIDUAL AXIS - As noted earlier, the spreading wave of depolarization can be depicted by vectors. Figure 9 shows the average vectors at 5 successive time points during ventricular depolarization. To determine the sign (+ or -) and magnitude of a recording in any given lead at any given time, drop a perpendicular line from the arrow of the vector of interest to that lead's axis line. The direction of the vector (+ or -) is as follows: a) When the vector is in the same direction as the lead = positive deflection b) When the vector is in the opposite direction as the lead = negative deflection c) When the vector is perpendicular to the direction of the lead = isoelectric (no deflection) MOHD1 The Electrocardiogram page 7 Christopher J. Benson, M.D. Figure 9 C. Augmented limb leads: aVR, aVL, and aVF; also in the frontal plane – measured between one limb and the average of the other two limb leads (Fig. 10) aVR aVL aVF Figure 10 1. Connections a) aVR – right arm (+) versus sum of aVL and aVF (-) b) aVL – left arm (+) versus sum of aVR and aVF (-) c) aVF – left foot (+) versus sum of aVR and aVL (-) 2. orientation is shifted midway (30) between the standard limb leads (I, II, and III) MOHD1 The Electrocardiogram page 8 Christopher J. Benson, M.D. D. Einthoven’s triangle and the hexaxial coordinate system (frontal plane) (Fig. 11) EINTHOVENS TRIANGLE HEXAXIAL CIRCLE Figure 11 1. Leads on arms are electrically equivalent to shoulders, and lead on left leg is equivalent to a connection at the groin. This defines Einthoven’s Triangle. Lead on right leg is a ground. 2. The positive and negative ends of these six leads define axes every 30 degrees in the frontal plane (hexaxial circle). E. Mean QRS axis – a measure of direction of mean ventricular depolarization vector (see Figure 7). Calculated from the average voltage of the QRS complex in the frontal leads. 1. The normal mean QRS axis is from -30 to +90 degrees (Fig. 12A); thus if the QRS is positive in both Lead I and Lead II, the axis is in the normal range (be sure to understand this). 2. Isoelectric method (also see examples in Fig. 21-12 in Boron and Boulpaep) – First, choose a near isoelectric lead (equal positive and negative deflections – lead III in Fig. 12B. The mean QRS vector will be perpendicular to that lead (leaves you two choices – either +30 or -150 degrees). Next, choose a lead that is highly positive or highly negative to determine the correct mean QRS vector (note that in this example the arrows denote the net voltage of the entire QRS complex for each lead). Since leads I and II are positive, then mean QRS vector is +30 degrees. 3. abnormal mean QRS vector a) physiological: heart position - dependent upon posture (erect or supine) as well as body build (tall and thin vs. short and obese) b) pathological: ventricular hypertrophy, myocardial damage, or bundle branch block MOHD1 The Electrocardiogram page 9 Christopher J. Benson, M.D. A B I III -120o -60o- III + o - o - 180 I I+ 0 +30o + IIIo +120 +60 o Figure 12 F. Precordial leads: V1 through V6 – record in the horizontal plane; the positive connection is one of the six locations on the chest wall, and the negative connection is electrically defined in the middle of the heart by averaging the three limb electrodes (Fig. 13) Figure 13 MOHD1 The Electrocardiogram page 10 Christopher J. Benson, M.D. V. Genetic mutations in cardiac ion channels can predispose to rhythm abnormalities (arrhythmias) A. Figure 14 is a more detailed description of the ionic currents (left) and their underlying channels (right) that contribute to the formation action potentials and electrical excitation of myocytes. We will focus on the delayed rectifier (IKr) current that contributes to repolarization. Figure 14 B. Multiple different mutations in KCNH2 (hERG) – see green dots Fig. 15A – cause a delayed activation of the hERG channel, which delays repolarization and prolongs the action potential of the myocytes (Fig. 15B). This prolongation of the action potential can allow for Ca2+ channels to recover from inactivation before the tissue has completely repolarized, which can allow for them to be activated again – causing an early depolarization, or early after-depolarization (EAD) (Fig 15C). These EADs can perpetuate and cause sustained arrhythmia, syncope, and sudden cardiac death. Several drugs, including the popular antihistamine, terfenadine, have been removed from the market because they were found to block hERG, and thus mimic the genetic mutations that delay hERG activation. The FDA now requires all new drugs to be tested for their effect on hERG channels. The prolonged action potentials manifest as a prolongation of the QT interval (Fig. 15D). The QT interval can be prolonged by mutation of many other ion channels (collectively termed congenital long QT syndromes), drugs, as well as metabolic and electrolyte disturbances, and is monitored by clinicians as a marker for potentially fatal arrhythmias. MOHD1 The Electrocardiogram page 11 Christopher J. Benson, M.D. A KCNH2 (hERG) C LQT2 B D Long ___ QT WT ---- LQT2 interval Figure 15 MOHD1 The Electrocardiogram page 12 Christopher J. Benson, M.D.