L4 Cardiac Electrophysiology and Mechanics Lecture PDF
Document Details
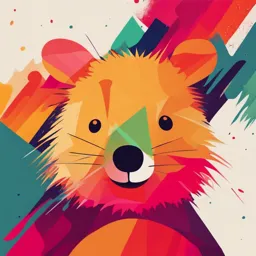
Uploaded by UnquestionableKremlin
Rutgers University
2024
Krista N. Blackwell, Ph.D.
Tags
Summary
Lecture notes on cardiac electrophysiology and mechanics, covering topics such as cardiac action potentials, refractory periods, conduction pathways, and the electrocardiogram (ECG).
Full Transcript
Electrical and Mechanical Activity of the Heart Krista N. Blackwell, Ph.D. Email: [email protected] Helpful Websites Cardiovascular Physiology Concepts: http://cvphysiology.com/ At the end of this lecture you should be able to: Identify the components of the cardiovascular system and the des...
Electrical and Mechanical Activity of the Heart Krista N. Blackwell, Ph.D. Email: [email protected] Helpful Websites Cardiovascular Physiology Concepts: http://cvphysiology.com/ At the end of this lecture you should be able to: Identify the components of the cardiovascular system and the describe their major functions Define the three properties that determine normal electrical activity of the heart Describe the differences between pacemaker and non-pacemaker action potentials and identify the areas in which they are present Distinguish and describe the differences between the sodium, calcium and potassium channels in the heart Define the cardiac refractory period and its phases, understanding its importance to normal contraction of the heart Characterize the variations in the conduction velocity in various regions of the heart Identify the cardiac pacemakers and understand why overdrive suppression regulates the primary pacemaker Distinguish the effects of sympathetic and parasympathetic innervation on heart rate, and conduction velocity At the end of this lecture you should be able to: Describe the conduction pathways and the wave of depolarization that moves throughout the heart Understand the relationship between the action potential and the electrocardiogram (ECG) Describe, label and explain the waves, complexes, segments and intervals on a normal ECG Compare the conduction velocities at the AV node and Purkinje fibers and identify why these differences exist Define and calculate the cardiac output List the factors that control cardiac output – Identify which factors are cardiac factors – Identify which factors are coupling factors Demonstrate how changes in preload and afterload affect the pressure-volume loop Describe the relationship between preload and the Frank-Starling relationship of the heart How do we study Cardiovascular Physiology? Cardiovascular electrophysiology is the study of the electrical activity of the heart Cardiovascular mechanics is the study of the contractile properties of the heart Cardiovascular hemodynamics is the study of blood flow and the factors that control it The Cardiovascular System consists of a systemic circuit and a pulmonary circuit The heart is a hollow muscular pump that pumps the blood throughout the vasculature Internal Anatomy of the Heart and Cardiac Valves Valves of the Left Heart Semilunar Valves Atrioventricular (AV) Valves Aortic (left) and Pulmonic (right) valves Prevent a backflow of blood into the ventricles Mitral (bicuspid) and tricuspid valves Prevent the backflow of blood into the atria Distribution of Cardiac Output to the Body Systems Normal Venous Return - 5L/min Normal Cardiac Output - 5L/min Cardiac Electrophysiology Cardiac Electrophysiology: Electrical Events of the Heart Cardiac Action Potential Cardiac Refractory Period Conduction of the Action Potential Modulation of electrical activity by the autonomic nervous system – Heart rate and conduction velocity The Electrocardiogram (ECG) Heart function is characterized into electrical and mechanical events The mechanical activity of the heart (contraction) is triggered by an electrical stimulus (action potential) that is generated within the heart. Three properties that determine the Normal Electrical Activity Automaticity – The ability of the heart to spontaneously fire action potentials Excitability (responsiveness) – The ability of the cardiac cells to respond to electrical activity (under normal conditions) Conductivity – The ability of the heart to conduct the action potential from its site of origin to all cardiac cells Pacemaker Cells Refractory Period Cardiac muscle anatomy (functional syncytium) Heart cell (cardiac myocyte) ion concentrations are important to action potential generation The resting membrane potential of cardiac cells, usually between -60 and -85 mV, is determined by the concentration differences of the ions across the cell membrane and the conductance of each ion to the membrane. There are two types of cardiac muscle cells that generate different action potentials Contractile Cell Action Potential Pacemaker (Autorhythmic) Cell Action Potential 0 3 4 Contractile cells make up 95-99% of the of the myocardial cells of the heart Atrial and Ventricular Muscle Cells Pacemaker cells make up 1-5% of the myocardial cells and are responsible for initiating heart action potentials Sinoatrial (SA) Node and Atrioventricular (AV) Node There are two types of cardiac muscle cells that generate different action potentials Contractile Cell Action Potential Pacemaker (Autorhythmic) Cell Action Potential 0 3 4 Phase 0 = Depolarization Phase 1 = Transient (early) repolarization Phase 2 = Plateau phase Phase 3 = Repolarization Phase 4 = Resting potential Phase 0 = Depolarization Phase 3 = Repolarization Phase 4 = Pacemaker potential Contractile Cell Action Potential Phases of the Contractile Cell Action Potential Phase 0 = Depolarization: voltage-gated sodium channels open Phase 1 = Transient (early) repolarization: transient-outward channels open and sodium channels close Phase 2 = Plateau phase: balance between open calcium and potassium channels Phase 3 = Repolarization: opening of delayed rectifier potassium channels Phase 4 = Resting membrane potential: opening of inward-rectifying potassium channels Pacemaker cells initiate the action potentials necessary for cardiac muscle contraction Figure 14.12 ACTION POTENTIALS IN CARDIAC AUTORHYTHMIC CELLS Autorhythmic cells have unstable membrane potentials called pacemaker potentials. The pacemaker potential gradually becomes less negative until it reaches threshold, triggering an action potential. Ion movements during an action and pacemaker potential State of various ion channels Membrane potential (mV) 20 Ca2+ channels close, K+ channels open 0 0 3 −20 −40 4 Ca2+ in −60 Action potential Time Lots of Ca2+ channels open Threshold Pacemaker potential K+ out Ca2+ in Net Na+ in If channels open Time Some Ca2+ channels open, If channels close If channels open K+ channels close Time Phase 4 (Pacemaker potential): If, funny channels (permeable to sodium ions) and ICa,T, transient calcium channels (allows entry of calcium ions) Phase 0 (Depolarization): ICa,L, L-type (DHP) calcium channels (allow the entry of calcium ions into the cell) Phase 3 (Repolarization): IK: delayed rectifier potassium channel (allows potassium ions to move out of the cell) Summary of Contractile and Pacemaker Cell Action Potentials PRACTICE QUESTION Properties that determine the normal electrical activity of the heart Automaticity – The ability of the heart to spontaneously fire action potentials Excitability (responsiveness) – The ability of the cardiac cells to respond to electrical activity (under normal conditions) Conductivity – The ability of the heart to conduct the action potential from its site of origin to all cardiac cells Pacemaker Cells Functional Refractory Period Cardiac muscle anatomy (functional syncytium) Cardiac Functional Refractory Period The functional refractory period allow the ion channels to recover from activation, before the next normal stimulus is applied The refractory period is divided into three phases – Absolute refractory period (ARP) The cell is unable to fire an action potential (regardless of the size of the stimulus) – Relative refractory period (RRP) An action potential can be fired with a larger-than-normal stimulus, but the shape of the action potential will be abnormal – The effective refractory period (ERP) consists of the absolute and relative refractory periods A normal action potential cannot occur during this phase – Supranormal phase the cell is transiently hyperexcitable and can fire a normal action potential Prevents tetanic contraction of the cardiac muscle Functional Refractory Period The duration of the action potential & refractory period prevents tetanus from developing in cardiac muscle Properties that determine the normal electrical activity of the heart Automaticity – The ability of the heart to spontaneously fire action potentials Excitability (responsiveness) – The ability of the cardiac cells to respond to electrical activity (under normal conditions) Conductivity – The ability of the heart to conduct the action potential from its site of origin to all cardiac cells Pacemaker Cells Functional Refractory Period Cardiac muscle anatomy (functional syncytium) Intercalated disks allow cardiac myocytes to operate as a functional syncytium www.trincoll.edu/.../Jason_Final-Muscle-Med.jpg Conduction of Cardiac Action Potentials The presence of gap junctions between cardiac muscle cells, allows a flow of current from the depolarized cell to a neighboring cell that has not been electrically stimulated Once ion movement depolarizes the cell membrane of the neighboring cell, an action potential will be elicited in this cell Cardiac Pacemakers and their Normal Firing Rates Tissue Action Potentials (beats) per minute SA Node (normal pacemaker) 60-100 AV Node 40 - 60 Purkinje Fibers 20 - 40 Overdrive suppression: results in the SA node serving as the normal pacemaker Sinoatrial (SA) Node Atrioventricul ar (AV) Node Purkinje Fibers PRACTICE QUESTION Sympathetic and parasympathetic innervation modulate the heart rate The intrinsic property of automaticity or spontaneous firing does not depend on stimulation from the nervous system to fire action potentials and cause muscle contraction The autonomic nervous system serves to modulate the rate of firing of cardiac action potentials. – Parasympathetic activation reduces the action potential firing rate – Sympathetic activation increases the action potential firing rate Sympathetic and Parasympathetic modulation of heart rate demonstrates antagonistic control of heart function http://emedicine.medscape.com/article/1923077overview The Cardiac Conduction System The conduction velocity varies in different areas of the heart 0.5 m/sec 0.5 m/sec 0.05 m/sec 2.0 m/sec 2.0 m/sec 2.0 m/sec 0.5 m/sec 0.5 m/sec 0.5 m/sec 4.0 m/sec The cardiac conduction system allows the electrical impulse to travel from the SA node through the ventricles Sample EKG Report – Male Patient An action potential represents the electrical activity in one cell, while the electrocardiogram (ECG) represents multiple action potentials in the heart within period of time The Electrocardiogram (ECG) provides information on the heart rate and rhythm, conduction velocity and condition of the heart tissue The key electrical events are represented by waves, segments and intervals on the ECG Recording Wave = an upward or downward deflection in the isoelectric line P wave: atrial depolarization QRS Complex: ventricular depolarization T wave: ventricular repolarization Segment = the isoelectric line only PR segment: goes from the end of the P wave to the beginning of the QRS Complex ST segment: goes from the end of the QRS complex to the beginning of the T wave The key electrical events are represented by waves, segments and intervals on the ECG Recording Intervals are composed of both waves and segments PR interval(Duration: 0.12 to 0.2 secs): goes from the beginning of the P wave to the beginning of the QRS complex and represents the time it takes for action potentials to travel from the SA node through the AV node QRS Interval (Duration: 0.06 to 0.1 secs) Goes from the beginning to the end of the QRS complex and represents the time it takes for ventricular depolarization QT interval (Duration: < 0.42 secs): Goes from the beginning of the QRS complex to the end of the T wave and represents the time between the initiation of the QRS complex and the end of the T wave Summary of the key electrical events recorded on the ECG ECG Paper Calibration The 12-Lead ECG Recording 10 Leads (surface electrodes) are placed on the body: limbs and the chest 12 electrical vectors are recorded – 9 are direct measurements: Leads I-III and V1-V6 – 3 are augmented measurements: aVL, aVR and aVF www.bigcscottsboro.com/ekg.html ECG Limb Leads: Bipolar and Augmented Unipolar ECG Leads Negative Electrode Positive Electrode I Right Arm Left Arm II Right Arm Left Leg III Left Arm Left Leg LEAD LEAD Positive Electrode aVR Right Arm aVL Left Arm aVF Left Leg ECG Precordial (Chest) Leads LEAD Positive Electrode V1 Fourth intercostal space, right sternal border V2 Fourth intercostal space, left sternal border V3 Equidistant between V2 and V4 V4 Fifth intercostals space, midclavicular line V5 Lateral to V4, anterior axillary line V6 Lateral to V5, midaxillary line Cardiac Contractile Function Cardiac Mechanics: Contractile Events of the Heart Cardiac Muscle Contraction Length-tension relationship Force velocity curves The Cardiac Cycle Regulation of Cardiac Output Modulation by the autonomic nervous system PRACTICE QUESTION Heart function is characterized into electrical and mechanical events The mechanical activity of the heart (contraction) is triggered by an electrical stimulus (action potential) that is generated within the heart. Cardiac Cycle and the Wiggers Diagram Cardiovascular events recorded during the cardiac cycle – Pressures: Atrial Ventricular Aortic – Ventricular Volumes – Electrocardiogram (ECG) – Phonocardiogram (Heart Sounds) Note: Isovolumic ventricular relaxation is the same as the phase identified as early ventricular diastole Cardiac Cycle and the Wiggers Diagram Phases of the cardiac cycle 1. Atrial Systole 2. Isovolumic (isovolumetric) ventricular contraction 3. Ventricular Systole (Rapid and slow phases) 4. Isovolumic (isovolumetric) Ventricular Relaxation 5. Ventricular Filling (Rapid and slow phases) Note: Isovolumic ventricular relaxation is the same as the phase identified as early ventricular diastole Cardiac Cycle and the Wiggers Diagram Ventricular Systole – Isovolumic Ventricular Contraction – Ventricular Ejection (Rapid & Reduced) Ventricular Diastole – Isovolumic Ventricular Relaxation – Ventricular Filling (Rapid & Reduced) Atrial Contraction Internal Anatomy of the Heart and Cardiac Valves Valves of the Left Heart Semilunar Valves Atrioventricular (AV) Valves Aortic (left) and Pulmonic (right) valves Prevent a backflow of blood into the ventricles Mitral (bicuspid) and tricuspid valves Prevent the backflow of blood into the atria Changes in Pressure Gradients can cause Cardiac Valves to Open and Close Valve opens Higher pressure at the rear of the valve Valve closes Higher pressure at the front of the valve Left Atrial Pressure Curve The Left Atrial Pressure Curve has three main peaks which are represented on the Wiggers’ Diagram; the a wave, the v wave and the c wave. The a wave represents atrial contraction. As the atria contract, the pressure within this chamber increases, driving the blood into the ventricles through the AV valves. The c wave occurs during isovolumic ventricular contraction. The rapid closing of the valves causes a rise in pressure in the atria which transmits a pressure wave to the atria and the large veins (causes a bulging of the mitral valve). The v wave occurs with atrial filling. The AV valves are closed and the atria are filling with blood Left Ventricular Pressure Curve The ventricular pressure curve demonstrates changes in ventricular pressure during systole and diastole At the beginning of isovolumic ventricular contraction there is closure of the AV valves because the ventricular pressure exceeds atrial pressure. Once the AV valves closes the heart muscle begins to contract isometrically and the pressure quickly rises. Left Ventricular Pressure Curve Once the ventricular pressure exceeds the aortic (or pulmonary artery) pressure, semilunar valves (aortic) open resulting in the ejection of blood Ejection can be divided into two phases: rapid and slower ejection When the arterial (aortic) pressure exceeds the ventricular pressure, the semilunar valves close and isovolumic ventricular relaxation begins. During this phase there is a large drop in ventricular pressure. Aortic Pressure Curve The aortic pressure curve represents the changes in aortic (arterial pressure) during one cardiac cycle. At the end of isovolumic ventricular contraction, the left ventricular pressure exceeds aortic pressure and the semilunar (aortic) valves open. Prior to the valve opening the lowest point of the aortic pressure curve is the diastolic blood pressure. As blood is ejected, the LV and aortic pressures are similar. As the blood reaches its peak/highest pressure, this value is known as the systolic blood pressure. Once ejection of the blood into the vessels is complete, the dicrotic notch (incisura) results from the closure of the aortic valves, marking the end of ventricular systole. Left Ventricular Volume Curve The filling phase of the heart ends with atrial contraction, which “kicks” the blood in the atria into the ventricles. The volume of blood in the ventricle during isovolumic ventricular contraction is the enddiastolic volume (EDV)(Point E). Once the aortic valve opens, blood is ejected from the ventricles. Left Ventricular Volume Curve During rapid ejection 2/3rd of the ventricular volume is emptied into the aorta, while during slower ejection the ventricles begin to relax and blood flows out at a slower rate. The volume of blood remaining in the ventricle after ejection is the end-systolic volume (ESV) (Point F) and can be determined during isovolumic ventricular relaxation. When the AV valves open, rapid filling begins. During this phase the majority (2/3) of blood enters the ventricle and with slower filling (diastasis) the remaining blood enters at a much slower rate. Heart Sounds (Phonocardiogram) Two audible sounds and abnormal sounds (S3 and S4) that can occur (typically not audible). The audible heart sounds are S1 and S2. The first heart sound (S1) is caused by the vibration of the chamber walls with the closure of the AV valves. The second heart sound (S2) results with the closure of the semilunar valves during isovolumic ventricular relaxation Summary of the phases & events of the Cardiac cycle PRACTICE QUESTION Cardiac Cycle Calculations and Normal Values Stroke volume (SV) = EDV (end-diastolic volume) – ESV (end-systolic volume) Ejection Fraction = Stroke volume (SV) ÷ EDV (end-diastolic volume) * 100% Pulse Pressure (PP) = Systolic Blood Pressure (SBP) – Diastolic Blood Pressure (DBP) Mean Arterial Blood Pressure (MABP) = Diastolic Blood Pressure (DBP) + 1/3 (PP) Cardiac Parameter Normal Value (range) Heart Rate (HR) 70 (60 – 100) beats/minute Stroke Volume (SV) 70 mL/beat Cardiac Output (CO) 5 – 7 Liters/minute Blood Pressure (BP) 120/80 mmHg Ejection Fraction (EF) ~55% (55 – 70%) or 0.55 The pressure-volume loop depicts the changes in ventricular pressure and volume that in one cardiac cycle Cardiac Output Cardiac output (CO) is the volume of blood pumped by the ventricle per unit of time (mL/minute) CO = Stroke Volume (ml/beat) X Heart Rate (beats/minute) Stroke volume (SV) = the volume of blood pumped per ventricle per contraction SV = EDV - ESV Heart rate (HR) = the total number of cardiac cycles (beats) per minute Changes in Stroke Volume and Heart Rate Regulate Cardiac Output Regulation of Cardiac Output Cardiac output is regulated by the interplay of four factors – Coupling factors: involve the functional coupling of the heart and blood vessels Preload (the degree of myocardial stretch before contraction begins) » Is due to the blood filling the ventricles (i.e. venous return) Afterload (the force that the ventricles must generate in order to eject blood out of the heart) » Mainly due to the arterial resistance – Cardiac Factors: intrinsic to the heart and are modulated by neural and hormonal stimulation Heart Rate (number of heart beats per unit of time) Contractility (intrinsic ability of the heart to contract - independent of preload and afterload) Contractility and inotropy (the level of calcium in the cytoplasm of the muscle cell) are synonymous The pressure-volume (PV) loop can demonstrate how changes in preload, afterload and contractility can affect the cardiac cycle Preload determines the force of contraction needed to eject the blood out of the ventricle Preload is the degree of myocardial stretch before contraction begins – Is determined by filling of the left ventricle or the end-diastolic volume (EDV) of the ventricle – Determined by the venous return Each ventricular contraction is to maintain the following relationship: – Venous Return (VR) = Cardiac Output (CO) Changes in Preload on the PV Loop are indicated by EDV The main factor that affects preload is the venous return Venous return: the volume of blood that enters the right atrium from the venous circulation Factors that can affect the preload and venous return include: – Changes in the venous vascular resistance – venous capacitance (volume of blood stored in the venous circulation) – ventricular filling time Preload can be measured clinically by determination of the following parameters Right atrial pressure Thoracic venae cava pressure Central venous Pressure Preload and the Frank-Starling Law of the Heart Frank-Starling Law of the Heart = Preload of the Heart Frank-Starling relationship – States that the volume of blood ejected by the ventricle depends on the volume of blood present in the ventricle at the end of diastole. – When venous return increases End diastolic volume increases, and Stroke volume increases – This relationship between EDV and stroke volume remains linear, until very high levels of EDV are reached Frank-Starling Relationship Afterload is the force required by the ventricle to overcome the resistance of the arterial system Afterload results from many factors that can impede the ejection of blood from the ventricles Changes in the afterload can result from alterations in the – – – – The volume of blood in the arterial circulation (arterial blood volume) The pressure in the aorta at the onset of ejection (MABP) The compliance of the aorta (ability of the aorta to expand) The size of the aortic valve (normal or narrowed) Changes in Afterload on the PV Loop are depicted by the point that represents the aortic valve opening When the afterload (aortic pressure) is increased – The pressure against which the ventricle contracts is much greater – The larger pressure increases the resistance to the ejection of blood, results in more blood remaining in the left ventricle and a higher endsystolic volume (ESV) When an increased afterload persists, the heart compensates under these condition by increasing the EDV to maintain a normal stroke volume.