Ch 10-11 Study Guide PDF
Document Details
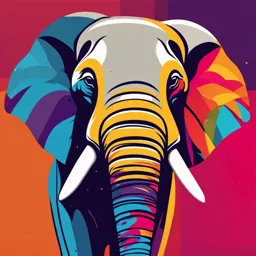
Uploaded by AmazedElectricOrgan
Tags
Summary
This study guide covers somatosensory sensations, proprioception, sensory transduction, and sensory areas of the cerebral cortex. It includes details on receptors and pathways, as well as the anatomy of the eye.
Full Transcript
Ch 10-11 Study Guide 1. Somesthetic sensations and proprioception (pg. 254) - Primary somatosensory cortex (somesthetic sensations and proprioception) - Sensory systems include: o Somesthetic sensations (somatosensation) (somatic): ▪ The sensations of the skin o Proprioception: ▪ Perception of limb...
Ch 10-11 Study Guide 1. Somesthetic sensations and proprioception (pg. 254) - Primary somatosensory cortex (somesthetic sensations and proprioception) - Sensory systems include: o Somesthetic sensations (somatosensation) (somatic): ▪ The sensations of the skin o Proprioception: ▪ Perception of limb and body positions. o Special senses o Vision o Hearing o Balance & equilibrium o Taste o Smell 2. Sensory Transduction (pg. 255; Figure 10.2) - Sensory Transduction: o This is one of the functions of sensory receptor, it is the conversion of stimulus energy into electrical energy. o The receptors convert the energy of a sensory stimulus into changes in membrane potential called: ▪ Receptor potentials or Generator potentials: It causes graded potentials. It causes the opening or closing of ion channels. It gets triggered by sensory stimuli. o If the receptor potential exceeds threshold, it can generate an action potential. 3. Sensory areas of cerebral cortex (Figure 10.4) - Sensory areas of cerebral cortex: o Vestibular cortex o Somatosensory cortex o Gustatory cortex (taste) o Olfactory cortex o Visual cortex o Auditory cortex 4. Generalized pathway for sensory information transmission (pg. 257-258; Figure 10.6) - First-order neuron: o They are afferent neurons that transmit information from the periphery to the CNS. - Second-order neuron: o They are interneurons that transmit information to the Thalamus. - Third-order neuron: o It transmits information to the cerebral cortex, where the perception of the information occurs. 5. Two-point discrimination (pg. 261) - Two-point discrimination: o This is the ability of a person to perceive two fine points pressed against the skin as two distinct points. o The minimum distance that must exist between two points for them to be perceived as separate is termed the two-point discrimination threshold. o The smaller the receptive fields, the greater the ability to discriminate two points, and the greater the tactile acuity. 6. Somatosensory receptors (pg. 262 - Somatosensory receptors: o Proprioceptors: ▪ These are the receptors in muscles, tendons, ligaments, and joints which provide proprioception or sense of the body’s position, movement, and orientation in space. o Mechanoreceptors: ▪ This receptor detects pressure, force, or vibration. ▪ Rapidly adapting receptor: Pacinian corpuscle Meissner's corpuscle Hair follicle receptor ▪ Slowly adapting receptor: Free nerve endings Merkel's disk Ruffini's ending o Thermoreceptors: ▪ This receptor detects skin temperature. ▪ Warm receptors: Free nerve endings Respond to temperatures in the range of 30–43°C. Increased frequency with increase in temperature ▪ Cold receptors: Possible free nerve endings Respond to temperatures in the range of 35–20°C. Increased frequency with decrease in temperature o Nociceptors: ▪ This receptor detects tissue-damaging stimuli (pain). ▪ Free nerve endings ▪ A-delta or C fibers ▪ Classes: Mechanical Thermal Polymodal 7. Somatosensory cortex (pg. 265) - Somatosensory cortex: o Columnar organization ▪ One column—one modality 8. Somatosensory pathways (pg. 265-266; Figure 10.15) - Dorsal Column-Medial Lemniscal pathway: o It transmits information from mechanoreceptors (touch & pressure) and proprioceptors (proprioception) to the thalamus (CNS). o (1) The first-order neurons (afferent) starts from the PNS then enters the dorsal horn of the spinal cord then meets with the second-order neuron in the medulla. o (2) The information from first-order neurons (afferent) is received by second-order neuron. o (3) The second-order neuron sends information to the Thalamus then connects/synapses with the third-order neuron. o (4) Then the third-order neuron transmits information from the Thalamus to the Primary Somatosensory Cortex. - Spinothalamic Tract: o It transmits information from thermoreceptors (temperature) and nociceptors (pain) to the thalamus. o (1) Sensory information from the periphery is detected by nociceptors and thermoreceptors and transmitted via first-order neurons to the dorsal horn of the spinal cord. o (2) First-order neurons may ascend or descend for a short distance (a few spinal segments) along Lissauer’s tract before reaching the dorsal horn. o (3) Eventually, first-order neurons form synapses with second-order neurons in the dorsal horn of the spinal cord. o (4) Second-order neurons cross over to the contralateral side of the spinal cord. o (5) Upon crossing over, second-order neurons ascend in the anterolateral quadrant of the spinal cord through the brainstem. o (6) The ascending second-order neurons terminate in the thalamus after traveling through the brainstem. o (7) In the thalamus, second-order neurons form synapses with third-order neurons. o (8) Third-order neurons ascend from the thalamus to the somatosensory cortex, specifically the primary somatosensory cortex (S1). 9. ** Review Anatomy of Eye: - Anatomy of the Eye: o The eye is filled with various fluids that help maintain its shape and provide nutrients to different structures. o Three layers: ▪ Outermost Sclera and cornea ▪ Middle Choroid, ciliary body, iris, zonular fibers, lens ▪ Inner Retina, photoreceptors, fovea Optic disk (blind spot) 10. Emmetropia, myopia, hyperopia (pg. 274) - Emmetropia: o normal vision Myopia: o near-sightedness Hyperopia: o far-sightedness 11. Regulation of the amount of light entering the eye (pg. 276, Figure 10.28) - What regulates the amount of light entering the eye: o The size of the pupil determines the amount of light that enters the eye. ▪ Hole opening in center: ▪ pupil o (a) The Iris which consists of two layers of smooth muscle: ▪ Inner circular muscle: Constrictor ▪ Outer circular muscle: Dilator o (b) Pupillary constriction: ▪ Which is caused by parasympathetic stimulation of the circular muscle layer of the iris. o (c) Pupillary dilation: ▪ Which is caused by sympathetic stimulation of the radial muscle layer of the iris. 12. Retina (pg. 276; Figure 10.29) - Retina: o It is composed of neural tissue, is the location of photoreceptors—that is, the rods and cones. o Three layers: ▪ Outer: Photoreceptors: Rods: o It provides the ability to see in black and white during relatively low light conditions, such as the light provided by the moon at night. Cones: o It provides us with color vision, but they are active only in relatively bright light, such as the sunlight during the day. ▪ Middle: Bipolar, amacrine, and horizontal cells ▪ Inner: Ganglion cells o Cells of the retina: ▪ Rods and cones are receptor cells. ▪ Rods and cones communicate with bipolar cells. ▪ Bipolar cells communicate with ganglion cells. ▪ Axons of ganglion cells form the optic nerve. ▪ Horizontal and amacrine cells provide lateral modulation. o Related with macular degeneration: ▪ This is when the vision in the center of the visual field is impaired as people age. o Note: The light must pass through the inner and middle layers of the retina before striking the photoreceptors in the outer layer. Deep within the retina is the retinal pigment epithelium, which absorbs light. 13. Phototransduction (pg. 277-279; Figure 10.32-10.33) - Phototransduction: o It’s the conversion of light energy into electrical signals—is carried out by the rods and cones. o First step of phototransduction: ▪ absorption of light o Photopigment: ▪ the molecule in the photoreceptors that absorbs light. o Rods: ▪ They are highly sensitive to dim light and are primarily responsible for vision in low-light conditions, such as night vision (scotopic vision). ▪ Photopigment made from opsin: Rhodopsin: o It consists of a protein called opsin and a lightsensitive molecule called retinal. o Cones: ▪ ▪ - They are less sensitive to light compared to rods but are responsible for color vision and high-acuity vision in bright light conditions (photopic vision). Photopigments made from opsin: L (red), M (green), and S (blue) opsins The specific details of phototransduction in rods (e.g. events in dark vs. events in light): o In rods in the dark: ▪ (1) Levels of second messenger cGMP are high. ▪ (2) cGMP opens Na+ channels on the membrane of the outer segment. ▪ (3) Na+ moves into cell. ▪ (4) Photoreceptor is depolarized, opening Ca2+ channels. ▪ (5) Ca2+ entry triggers neurotransmitter secretion. ▪ (6) Neurotransmitter acts on bipolar cells. o In rods in the light: ▪ (1) Rhodopsin within membrane of disks ▪ (2) Light absorbed by rhodopsin ▪ (3) Retinal and opsin dissociate ▪ (4) Bleached opsin activates transducin (G protein) ▪ (5) Transducin activates phosphodiesterase ▪ (6) Phosphodiesterase catalyzes cGMP ▪ (7) Without cGMP, Na+ channels close ▪ (8) Hyperpolarization of cell occurs by K+ leaving ▪ (9) Hyperpolarization closes Ca2+ channels and secretion stops 14. Neural pathway for vision – from photoreceptors to visual cortex (pg. 283) - Neural pathway for vision – from photoreceptors to visual cortex: o (1) Retina: ▪ Photoreceptors (rods and cones) in the retina detect light stimuli and convert them into neural signals. ▪ Bipolar cells receive signals from photoreceptors, and ganglion cells receive signals from bipolar cells. o (2) Ganglion Cells: ▪ Ganglion cells are the output neurons of the retina. They receive inputs from bipolar cells and transmit visual information to the brain via their axons. ▪ Ganglion cell axons converge to form the optic nerve. o (3) Optic Nerve: ▪ The optic nerve (cranial nerve II) carries visual information from the ganglion cells of the retina to the brain. ▪ It exits the eye at the optic disc and travels toward the optic chiasm. o (4) Optic Chiasm: ▪ At the optic chiasm, some of the optic nerve fibers from each eye cross over to the opposite side, while others continue on the same side. ▪ This crossover ensures that visual information from both eyes is processed in both cerebral hemispheres. o (5) Optic Tracts: ▪ After the optic chiasm, the fibers are organized into optic tracts. o (6) Lateral Geniculate Body (LGN) of Thalamus Synapses: ▪ The optic tracts synapse with neurons in the lateral geniculate nucleus (LGN) of the thalamus. ▪ The LGN serves as a relay station, processing and transmitting visual information to the visual cortex. o (7) Optic Radiations: ▪ Neurons in the LGN send visual information via the optic radiations (geniculocalcarine tract) to the visual cortex. o (8) Visual Cortex Synapses: ▪ Visual information is transmitted from the optic radiations to the primary visual cortex (V1) located in the occipital lobe of the brain. ▪ V1 is responsible for the initial processing and interpretation of visual stimuli. o (9) Representation of Visual Fields: ▪ Visual information from the right visual field is processed in the left hemisphere of the brain, and vice versa. 15. ** Review Anatomy of Ear - Anatomy of Ear: o Outer ear: ▪ entry of sound waves ▪ Pinna ▪ External auditory meatus o Middle ear: ▪ amplification of sound waves ▪ Tympanic membrane ▪ Ossicles: malleus, incus, stapes ▪ Oval window ▪ Round window o Inner ear: ▪ transduction of sound energy ▪ Cochlea ▪ Vestibular apparatus o Eustachian tube: ▪ Equilibrate pressure ▪ Also known as the auditory tube 16. Sound Transduction by inner hair cells (pg. 289-291; Figure 10.46) - Sound Transduction by inner hair cells: o The bending of stereocilia in response to sound waves triggers changes in membrane potential and neurotransmitter release in inner hair cells, facilitating the conversion of mechanical energy into neural signals for auditory perception. - The specific details of sound transduction in hair cells: o (a) The mechanically gated potassium channels in stereocilia are partially opened when the cell is at rest (stereocilia stand upright), and potassium ions enter the cell, producing a small depolarization that is sufficient to release transmitter that communicates to the afferent neuron; the result is a low frequency of action potentials. o (b) When the stereocilia bend toward the taller stereocilium, the potassium channels open more widely, and more potassium ions enter the cell, producing a greater depolarization and a higher frequency of action potentials in the afferent neuron. o (c) When the stereocilia bend away from the taller stereocilium, the potassium channels close, and little potassium can enter the cell. Less transmitter is released, and the frequency of action potentials in the afferent neuron decreases. 17. Neural pathway for sound – from external ear to auditory cortex (pg. 291-292) - Neural pathway for sound – from external ear to auditory cortex: o (1) Hair Cells: ▪ Hair cells, which are receptor cells and modified neurons, detect sound vibrations in the cochlea of the inner ear. o (2) Cochlear Nerve: ▪ Afferent axons of cranial nerve VIII (cochlear nerve) carry auditory information from hair cells to the brainstem. o (3) Brainstem to Thalamus: ▪ In the brainstem, cochlear nerve fibers synapse with second-order neurons, which then project to the medial geniculate nucleus (MGN) in the thalamus. o (4) Auditory Cortex: ▪ From the MGN, third-order neurons project to the auditory cortex in the temporal lobe. o (5) Frequency Representation: ▪ The auditory cortex has a frequency map, representing different pitches or frequencies of sound: The auditory cortex has topographical organization which is tonotopic which maps the frequency of sound. 18. Signal transduction and neural pathway for taste – from taste buds to gustatory cortex (pg. 296-297) - Signal Transduction in Taste: o Four primary tastes with different transduction mechanisms: ▪ Sour: due to H+ ▪ Salty: due to Na+ ▪ Sweet: due to ligands ▪ Bitter: due to ligands o Taste Transmitters: ▪ ATP & Serotonin - Neural pathway for taste – from taste buds to gustatory cortex: o (1) Taste Receptor Cells: ▪ Taste buds detect chemical stimuli. o (2) Taste Afferent Neurons: ▪ Sensory neurons, from cranial nerves VII, IX, and X, transmit taste information. o (3) Gustatory Nucleus in Brainstem: ▪ Taste afferent neurons terminate in the gustatory nucleus of the medulla, synapsing with second-order neurons. o (4) Thalamus: ▪ Second-order neurons relay taste information to the thalamus. o (5) Gustatory Cortex: ▪ Third-order neurons project from the thalamus to the gustatory cortex in the parietal lobe, near the mouth region of the somatosensory cortex. 19. Neural pathway for olfaction – from olfactory receptors to olfactory cortex (pg. 299) - Neural pathway for olfaction – from olfactory receptors to olfactory cortex: o (1) Olfactory Receptor Cells: ▪ Olfactory receptor cells are specialized endings of afferent neurons located in the nasal epithelium. o (2) Olfactory Nerve (Cranial Nerve I): ▪ The axons of olfactory receptor cells comprise the olfactory nerve, also known as cranial nerve I. o (3) Olfactory Bulb: ▪ The axons of the olfactory nerve terminate in the olfactory bulb, located at the base of the brain. o (4) Glomeruli: ▪ Communication between afferent neurons and second-order neurons, known as mitral cells, occurs in specialized structures called glomeruli within the olfactory bulb. o (5) Olfactory Tract: ▪ Second-order neurons (mitral cells) form the olfactory tract, which extends from the olfactory bulb to higher brain regions. o (6) Olfactory Tubercle and Cerebral Cortex: ▪ The olfactory tract relays olfactory information to the olfactory tubercle, a structure in the limbic system. ▪ From the olfactory tubercle, olfactory information is further processed and transmitted to the cerebral cortex, specifically the olfactory cortex. 20. Dual innervation in the autonomic nervous system (pg. 304-305, Figure 11.1) - - Innervate: o To supply with nerves Or o To supply nerves to an organ, structure, or body part. Dual innervation in the autonomic nervous system: o It involves both the parasympathetic and sympathetic branches innervating most effector organs. o Primary function: ▪ To regulate organs to maintain homeostasis. o Parasympathetic and sympathetic activities tend to be antagonistic. ▪ Parasympathetic nervous system: Referred to as “rest & digest.” ▪ Sympathetic nervous system: Initiates fight-or-flight response that prepares the body for physical exertion. 21. Anatomy of the sympathetic nervous system (pg. 306-307, Figures 11.3, 11.4, 11.5) - Anatomy of the sympathetic nervous system: o Origin of Preganglionic Neurons: ▪ Originate in the thoracolumbar spinal cord. ▪ Located in the lateral horn and intermediolateral cell column. o General Anatomy: ▪ Short preganglionic neurons project to the sympathetic chain. ▪ Long postganglionic neurons extend from the sympathetic chain to effector organs. ▪ Ganglia are linked together in the sympathetic chain. o Exceptions: ▪ Collateral ganglia located outside the sympathetic chain. ▪ Adrenal medulla contains modified sympathetic postganglionic cells (chromaffin cells). ▪ Chromaffin cells release catecholamines: 80% epinephrine 20% norepinephrine and a small amount of dopamine. o Sympathetic Pathways: ▪ Preganglionic: Exit via the ventral root of the spinal cord and enter the spinal nerve. Axons leave the spinal nerve as white rami and enter sympathetic ganglia to communicate with postganglionic neurons. ▪ - Postganglionic: Leave ganglia as gray rami and reenter the spinal nerve. Travel to the effector organ in the spinal nerve. Some postganglionic neurons project to collateral ganglia. Anatomical pathways of preganglionic and postganglionic neurons in the sympathetic nervous system: - The most common pathway of sympathetic fibers: - Sympathetic innervation of the adrenal gland: o (a) The adrenal glands, located within fat pads on top of each kidney. o (b) Sympathetic preganglionic neurons innervate chromaffin cells of the adrenal medulla, stimulating the release of epinephrine into the blood. 22. The 3 anatomical arrangements of the sympathetic fibers, especially the most common arrangement (pg. 306-307, Figures 11.3, 11.4, 11.5) - The sympathetic fibers exhibit three anatomical arrangements, with the most common arrangement being: o Sympathetic Chain: ▪ The most common arrangement: Most sympathetic fibers follow this arrangement. ▪ Preganglionic neurons originate in the thoracolumbar spinal cord. ▪ Short preganglionic neurons project to ganglia in the sympathetic chain. ▪ Long postganglionic neurons extend from the sympathetic chain to innervate effector organs. o Collateral Ganglia: ▪ Located outside the sympathetic chain. ▪ Some preganglionic neurons project to collateral ganglia instead of the sympathetic chain. ▪ Postganglionic neurons from collateral ganglia innervate specific target organs, such as those involved in the abdominal and pelvic regions. o Adrenal Medulla: ▪ a very important exception to the arrangements: a group of long preganglionic neurons innervate endocrine tissue. Each of the two adrenal glands, which are located in fat pads on top of each kidney is divided into an outer cortex and an inner medulla. The medulla consists of modified sympathetic postganglionic cells, called chromaffin cells. ▪ Chromaffin cells release catecholamines: 80% epinephrine 20% norepinephrine and a small amount of dopamine ▪ Function as endocrine glands, releasing hormones in response to sympathetic stimulation. 23. Anatomy of the parasympathetic nervous system (pg. 308, Figure 11.6) - Anatomy of the parasympathetic nervous system: o Origin of Preganglionic Neurons: ▪ Preganglionic neurons originate in the brainstem or sacral spinal cord. o Preganglionic Neurons: ▪ Long preganglionic neurons extend from the brainstem or sacral spinal cord to ganglia located near the effector organ. ▪ Preganglionic neurons communicate with postganglionic neurons within these ganglia. o Postganglionic Neurons: ▪ Short postganglionic neurons extend from ganglia to effector organs. o Parasympathetic Nerves: ▪ Parasympathetic nerves include cranial nerves and spinal nerves. ▪ Cranial nerves associated with the parasympathetic system include: Cranial nerve X (Vagus nerve) Cranial nerve III (Oculomotor nerve) Cranial nerve VII (Facial nerve) Cranial nerve IX (Glossopharyngeal nerve) ▪ Spinal nerves include pelvic nerves, which are distinct from somatic spinal nerves. 24. Neurotransmitters and receptors in the autonomic nervous system (pg. 309, Figure 11.7) - Neurotransmitters and receptors in the autonomic nervous system: o Neurotransmitters: ▪ Acetylcholine: Released from Preganglionic neurons & Parasympathetic postganglionic neurons. ▪ Epinephrine: Hormone of the adrenal medulla released by 80%. ▪ Norepinephrine: Released from Sympathetic postganglionic neurons. Hormone of the adrenal medulla released by 20%: o Receptors: ▪ Cholinergic Receptors ▪ Adrenergic Receptors - (a) Neurotransmitters and receptors for the three distinct anatomical pathways of the sympathetic nervous system. - (b) Neurotransmitters and receptors in the parasympathetic pathway. 25. Types of cholinergic receptors (pg. 309, Figure 11.7) - Types of Cholinergic Receptors: o Nicotinic Cholinergic Receptors (ionotropic): ▪ Cause cation channels to open, resulting in depolarization. o Muscarinic Cholinergic Receptors (metabotropic): ▪ G protein-coupled receptors whose effects depend on the target cell. 26. Types of adrenergic receptors (pg. 310-311, Figure 11.7, Table 11.1) - Types of Adrenergic Receptors: o Two main classes: ▪ alpha and beta. o Each class has subclasses and is coupled to G proteins. o Alpha Receptors: ▪ Alpha 1 (α1) and Alpha 2 (α2). ▪ Located in effector organs of the sympathetic nervous system. ▪ Usually excitatory, with a greater affinity for norepinephrine than epinephrine. o Beta Receptors: ▪ Beta 1 (β1), Beta 2 (β2), and Beta 3 (β3). ▪ All activate cAMP. ▪ Affinities for norepinephrine and epinephrine vary. o Properties of β1 Adrenergic Receptors: ▪ Located in cardiac muscle and kidneys. ▪ Usually excitatory, with equal affinity for norepinephrine and epinephrine. o Properties of β2 Adrenergic Receptors: ▪ Located in some blood vessels and smooth muscle. ▪ Usually inhibitory, with greater affinity for epinephrine than norepinephrine. ▪ Located in adipose tissue, usually excitatory, with equal affinity for norepinephrine and epinephrine. - Receptor type Table 11. 1 Adrenergic Receptors: Effector organ with receptor type Relative affinities* Effect on effector organ NE > Epi Signal transduction mechanism Activates IP3 α1 Most vascular smooth muscle, pupils α2 CNS, platelets, adrenergic nerve terminals (autoreceptors), some vascular smooth muscle, adipose tissue NE > Epi Inhibits cAMP Excitatory β1 CNS, cardiac muscle, kidney NE = Epi Activates cAMP Excitatory β2 Some blood vessels, respiratory tract, uterus Adipose tissue Epi >> NE Activates cAMP Inhibitory NE = Epi Activates cAMP Excitatory β3 Excitatory 27. Autonomic neuroeffector junctions (pg. 312, Figures 11.9, 11.10) - Neuroeffector junction: o The synapse between an efferent neuron and its effector organ. - Autonomic neuroeffector junctions: o It is the site where neurotransmitters are released from postganglionic neurons to exert their effects on effector organs, facilitating the regulation of various physiological processes by the autonomic nervous system. o Varicosities: ▪ This is where neurotransmitters are released from numerous swellings located at intervals along the axons of these neurons. o Neurotransmitters are stored in axon swellings known as varicosities. o These varicosities are distributed along the postganglionic neuron's axon, allowing for widespread release of neurotransmitters. - Events at the neuroeffector junction: o (1) Action potential arrives at varicosity o (2) Voltage-gated Ca2+ channels open o (3) Ca2+ triggers exocytosis of neurotransmitter o (4) Neurotransmitter binds with receptors on effector organ o (5) Response in effector organ occurs o (6) Neurotransmitter is degraded and diffuses away; reuptake occurs 28. Anatomy of the somatic nervous system, particularly the relation between a muscle fiber to a neuron and a neuron to muscle fibers (pg. 315, Figure 11.14) - The anatomy of the somatic nervous system involves the interaction between motor neurons and skeletal muscle fibers: o Motor Neurons: ▪ Motor neurons originate in the ventral horn of the spinal cord. ▪ They innervate skeletal muscle fibers, transmitting signals from the CNS to the muscles. ▪ The neurotransmitter that is released by motor neurons is acetylcholine. o Receptors in Skeletal Muscle: ▪ Skeletal muscle fibers contain nicotinic cholinergic receptors. ▪ These receptors are activated by acetylcholine released from motor neurons, leading to muscle contraction. o Motor Unit: ▪ A motor unit consists of one motor neuron and all of the muscle fibers it innervates. ▪ Somatic motor neurons innervate skeletal muscle. ▪ Each motor neuron innervates many muscle fibers, forming a motor unit. Conversely, each muscle fiber is innervated by only one motor neuron. 29. Neuromuscular junction (pg. 315-317) - Neuromuscular junction: o This is the synapse between a motor neuron and a skeletal muscle cell. o Anatomy of the neuromuscular junction: ▪ Terminal Bouton: Also known as the axon terminal, it is the end of the motor neuron that comes into contact with the muscle fiber. ▪ Motor End Plate: This is a specialized region of the muscle membrane at the junction with the motor neuron. It contains nicotinic cholinergic receptors. o Neurotransmitter Release: ▪ All motor neurons release acetylcholine as their neurotransmitter. ▪ Acetylcholine is released from the terminal bouton into the synaptic cleft. o Receptors: ▪ The motor end plate of the muscle fiber contains nicotinic cholinergic receptors. ▪ These receptors are activated by acetylcholine, leading to muscle contraction. o Excitatory Synapses: ▪ Synapses at the neuromuscular junction are excitatory, meaning they lead to muscle contraction upon activation. o Acetylcholinesterase: ▪ This enzyme is present in the synaptic cleft and rapidly breaks down acetylcholine after it has activated the receptors. o Activities of the neuromuscular junction: ▪ Activation of motor neuron depends on summation of EPSPs and IPSPs ▪ Action potential in motor neuron triggers release of acetylcholine at neuromuscular junction End-plate potential occurs at motor end plate. o Communication at the Neuromuscular Junction: ▪ (1) Action potential arrives at terminal bouton ▪ (2) Voltage-gated calcium channels open ▪ (3) Calcium enters cell, triggering release of ACh ▪ (4) ACh diffuses across cleft and binds to nicotinic receptors on motor end plate ▪ (5) ACh triggers opening of channels for the small cations sodium and potassium ▪ (6) Net movement of positive charge in → depolarization End-plate potential (EPP) EPP > EPSP ▪ (7) EPP causes action potential in muscle cell ▪ (8) Action potential spreads through muscle, causing contraction