Spinal Cord PDF
Document Details
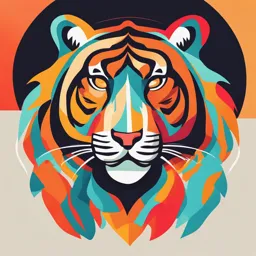
Uploaded by IngeniousFreesia3657
Tags
Summary
This document discusses the structure and function of the spinal cord. It details the cerebrospinal fluid system, the choroid plexus and related anatomy. The document also discusses hydrocephalus.
Full Transcript
Pietro Pillirone sbobinatore Junhuyk Song proofreader Neuroanatomy 06 Prof. Emmi 07/03/2023 THE SPINAL CORD The liquoral system The central canal of the spinal cord is connected to the inferior edge of the fourth ventricle of the brain, from which it receives cerebrospinal fluid and it continues dow...
Pietro Pillirone sbobinatore Junhuyk Song proofreader Neuroanatomy 06 Prof. Emmi 07/03/2023 THE SPINAL CORD The liquoral system The central canal of the spinal cord is connected to the inferior edge of the fourth ventricle of the brain, from which it receives cerebrospinal fluid and it continues downwards within the spinal cord. We then find two lateral cavities, the left lateral foramen of Luschka and the medial foramen of Magendie: these cavities allow communication between the ventricular system (fourth ventricle) and the subarachnoid space. Here we can appreciate a sagittal section of the nervous system and we can observe the ventricular cavities with the two foramina opening up towards the subarachnoid space, the space between the arachnoid and the pia mater, in which we find cerebrospinal fluid. Together with the ventricular system, the subarachnoid space forms the so-called liquoral system: the system in which the cerebrospinal fluid (or liquor) flows. The cerebrospinal fluid is a product of the filtration of blood plasma that, at the level of specific structures called choroid plexuses, is transformed into CSF. The choroid plexus is a structure within the ventricles, found at the level of the lateral third and fourth ventricles, that filtrates blood plasma to give rise to CSF. The CSF flows through the ventricular system, following the anatomical pathways we discussed previously: from the lateral ventricles it passes through the interventricular foramen of Monro, arrives at the third ventricle, where it joins the CSF produced here, it flows through the aqueduct of Sylvius, enters the fourth ventricle and joins the CSF produced here; it then continues downwards through the central canal or exits the fourth ventricle through the foramina of Luschka and Magendie, reaching the subarachnoid space1. Within this space, we find distinct regions in which CSF can accumulate, called cisterns: the CSF within the subarachnoid space flows mainly towards the superior margins of the brain and is reabsorbed within the dural venous sinuses. This happens at the level of arachnoid granulations, protrusions of the arachnoid membrane that allow the CSF to flow from the subarachnoidal space towards the venous blood contained in the superior sagittal sinus. 1 The flow of the CSF in the subarachnoidal space is represented by white arrows. 1 This flow, from the ventricular cavities to the subarachnoid space, to the dural sinuses, is called Liquoral circulation (or circulation of the CSF): its purpose is to carry away from the nervous tissue waste products that are produced during normal activity; it acts similarly to the lymphatic system in the rest of the body. Here you can see a representation of the choroid plexus, where CSF is produced from blood: we observe the bending of the vessels following ependymal cells of choroid epithelium. From the filtration of arterial blood, the choroid plexus gives rise to the CSF. The CSF produced in the ventricles flows in the subarachnoidal space, it’s reabsorbed in the venous system, returns in the heart and it’s reoxygenated in the lungs, then returning as arterial blood towards the choroid plexuses. There is continuous recycling of CSF: it’s a dynamic process of production and reabsorption occurring up to four times a day, carrying away metabolic waste products and inflammatory cells found in the nervous tissue. Here we see a representation of the arachnoid granulations at the level of the superior cervical sinuses, where the CSF is reabsorbed in the venous circulation, allowing the passage from subarachnoid space towards the venous blood. Hydrocephalus A correct clearance of CSF is crucial, and for this to happen, the ventricular system must be functional, and no occlusions can be present: in this case, the most frequent pathological condition is hydrocephalus, the symptomatic accumulation of cerebrospinal fluid (CSF) inside the cerebral ventricles. This accumulation may be due to obstruction in the normal flow of the CSF, to problems with absorption into the venous system by the Pacchionian arachnoid granulations, or to excessive production of CSF. Inflammatory processes can lead to the narrowing and obstruction of the aqueduct of Sylvius, leading to the continuous production of CSF in the lateral and third ventricles that can’t be reabsorbed, since it can’t flow through the aqueduct reaching the subarachnoid space: the accumulation of CSF in the ventricles leads to their enlargement. We can encounter different types of this condition: in the image, we see an example of triventricular hydrocephalus (affecting the two lateral and the third ventricle); we can also find a monoventricular hydrocephalus, affecting one of the two interventricular foramina of Monro; or occlusion of the foramina of Luschka and Magendie, which leads to the abnormal expansion of the ventricular cavities and compression of the brain. In newborns, thanks to the plasticity of bones of the cranial wall, it manifests as a functional deformation of the skull in response to the increased intracranial pressure. In adults, this deformation is not possible: hydrocephalus damages more severely the nervous tissue of the patient, because of the impossibility of the cranial walls to accommodate this increasing pressure. Another form of hydrocephalus is that occurring in elderly subjects, as in the clinical case mentioned before: it’s called hydrocephalus ex vacuo (or compensatory enlargement of the CSF spaces), a physiological, not 2 pathological enlargement of the ventricular cavity due to the shrinking (atrophy) of the surrounding nervous tissue. It’s a normal process occurring as we age since the brain loses consistency; to compensate for this encephalic volume loss, the ventricular cavities enlarge. The cerebrospinal fluid is an important indicator of the health of the nervous system: in clinical practice, lumbar puncture is performed to sample the CSF, which can give us useful information, mainly regarding ongoing infections (signaled by an increase of white blood cells in the CSF). It is now used also to diagnose neurodegenerative diseases since in these conditions neurons die and the cellular debris produced is absorbed within the liquor, and can be detected at higher levels compared to healthy subjects. Lumbar puncturing exploits the presence of the subarachnoid cisterns, and enlargements of the subarachnoid space. There are various minor enlargements at the level of the cranial cavity, in particular inferiorly and superiorly to the cerebellum and pons; however, the most important one is found at the level of the spinal cord: at the lower caudal extremity of the spinal cord, we find the Lumbar Cistern, a large reservoir of CSF. It’s an important anatomical structure because at this level we only find the rootlets of the nerves, but not the axial structure of the spinal cord itself: It’s thus a safe place where we can insert the needle in the subarachnoid space to sample the CSF, at the level of the lumbar vertebrae. The spinal cord This is a dorsal view showing us the structure of the spinal cord, following the sectioning of the lamina of the vertebrae forming the vertebral canal. We can observe the spinal cord in situ, with the vertebral canal of the vertebral column. 3 The spinal cord is the derivative of the neural tube that doesn’t undergo differential growth; it’s what is found below the three brain vesicles. It’s a cylindrical structure, approximately 44cm long and its average transverse diameter is 1 cm. The upper extremity continues indistinctly with the brainstem and we generally imagine a hypothetical plane passing through the last cranial nerve and the 1st spinal nerve. Inferiorly the spinal cord narrows down and becomes conical (conus medullaris): its length is shorter than that of the vertebral column (which is about 70-75cm); there is a big gap between the two lengths: at this level, where the vertebral canal continues and the spinal cord ends, we find the lumbar (spinal) cistern, a region at the level of the lower vertebral canal (starting from L2). The ascension of the spinal cord is the apparent rising of the spinal cord towards the cranial part of the body during development. It’s not a very accurate definition as it’s not rising, it just reflects a differential growth of the spinal cord when compared to that of the vertebral column. Only the upper ⅔ of the vertebral column is occupied by the spinal cord whereas the last ⅓ presents only the rootlets of the spinal nerves. The uppermost district is called the cervical district, below is the thoracic district (blue), followed by the lumbar, sacral, and coccygeal district of the spinal cord: it reflects the subdivision of the vertebrae. The vertebral column is a metameric structure: it’s formed by segments, each known as a single vertebra; this subdivision is reflected in the spinal cord, which also presents a segmental subdivision. Each vertebra has a correspondent segment in the spinal cord: at the level of the spinal cord these segments are called myelomeres, the functional units of the spinal cord controlling a part of the body. We can identify: 8 cervical myelomeres, 12 thoracic myelomeres, 5 lumbar myelomeres, 5 sacral myelomeres atrophic remains at the level of the coccygeal segment On the left is a representation of the segmental subdivision of the territories of the body, corresponding to the segments of the spinal cord. This organization is called the dermatomeric pattern of control: a dermatome is part of the skin controlled by a specific segment of the spinal cord (it reflects the segmental organization). The cervical regions are controlled by the cervical segments, the 4 thoracic region by the thoracic ones, the lumbar region by the lumbar segment, and the sacral region (perineum and lower body) by the sacral segments of the spinal cord. We also find enlargements: there are two main ones at the level of the cervical and lumbar myomeres. They are important from a functional perspective: it means that the average diameter of the spinal cord is actually 1.1-1.2 cm. The larger size of cervical and lumbar myelomeres reflects the presence of the plexuses, for the innervation of the upper and lower limbs. The spinal cord ends in a conical shape and below we find the lumbar cistern; it then continues downwards to the lower extremity of the vertebral canal as a small bundle of pia mater, called filum terminalis. The filum terminalis is the atrophic part of the spinal cord, that, present in other mammals, would be responsible for the nervous control of the tail. Within the dura mater and the subarachnoid space, in the lumbar cistern, we find the cauda equina (horse’s tail). The cauda equina is a system of nerves emerging from the spinal cord, and strongly verticalized within the spinal cord: the differential growth (ascension of the spinal cord) of the spinal cord and vertebral column mainly affects the lumbar region. Looking at the cervical and thoracic segments of the spinal cord, we see that each segment presents a pair of spinal nerves, used by these segments to ‘control’ specific parts of the body; these nerves exit from the intervertebral foramen of the corresponding vertebra. They have an almost transverse course and each spinal nerve exits from its corresponding intervertebral foramen. Early in development, we have perfect correspondence between segments of the spinal cord, spinal nerves, and the corresponding intervertebral foramen, from which its nerves emerge to go innervate the body. As growth progresses and the vertebral column grows inferiorly, the spinal cord is lifted: to accommodate for this apparent ascension, the nerves within the vertebral canal (at the level of the lumbar and sacral myelomeres), have to travel to the lower (empty) third of the spinal cord to reach the corresponding myelomeres. In the end, each pair of spinal nerves exits from their corresponding intervertebral foramen of the corresponding vertebra: what changes is the course of these nerves within the vertebral canal. In the upper segments (cervical and thoracic) it has a transverse course, going directly to the myelomeres, while in the lower segments (lumbar and sacral) there is a strong verticalization and the development of the bundle of nerves, known as cauda equina. When examining a spinal cord specimen, we can clearly identify the superior and inferior margins of the spinal cord by examining the cauda equina: it appears as a very consistent bundle of nerve fibers at the level of the caudal district of the vertebral canal. SQ: When you’re performing a lumbar puncture, isn’t it an issue if we perforate the nerves of the cauda equina? Yes, but when you perform it, you position the needle at the midline, so as the nerves move laterally exiting from the intervertebral foramina, you don’t risk hitting the nerves. SQ: Is the segmentation of the spinal cord visible morphologically? No, the myelomeres are not distinguishable morphologically, but they can be recognized thanks to the emergence of the spinal nerves. 5 Transverse section of the vertebral column, canal, and spinal cord within A) In image a), we’re looking at the section of a thoracic vertebra. We can observe the typical anatomy of these districts: the body of the vertebra, the lamina, and the spinous process: within these structures, we find the vertebral canal and the intervertebral foramen, through which spinal nerves exit the canal. Within the vertebral canal, we find the spinal cord lined by the three meningeal layers, the dura mater, the arachnoid, and the pia mater. There are however important anatomical differences between the spinal and the cranial meninges, in particular when referring to the dura mater: unlike in the cranial cavity, the dura mater doesn’t attach directly to the bone, but between the two there’s a layer of fat, the epidural fat, typical of the vertebral column. Within this layer, we find numerous venous plexuses, called Batson’s venous plexuses. The epidural fat is important in order to accommodate for the higher degree of movement allowed by the vertebral column; a direct attachment of the dura mater to the bone would lead to increased stress and consequent damage to the structure. The rest of the meninges display the same behavior as in the cranial cavity: below the dura mater, we find the arachnoid and the pia mater below it. In the transversal section above we can also observe the cylindrical spinal cord: each segment of the spinal cord (myelomeres) gives rise to a pair of spinal nerves. In image b on the left, we can see the transverse section of a lumbar vertebra. Consider the dramatic decrease in the diameter of the spinal cord: most of the space is occupied by the cauda equina: vertically oriented fibers, and rootlets of spinal nerves descending within the vertebral canal to exit from the intervertebral foramina. 6 This is another representation of the spinal cord, outside of the vertebral canal, where we can observe the meninges. It’s important to understand the segmental organization of the spinal cord: we don’t see any visible boundary between consequent segments, however, the segmentation is given by the emergence of the spinal nerves coming out of the spinal cord. When we talk about spinal nerves, we refer to structures allowing communication between CNS (spinal cord) and peripheral structures. Spinal nerves are mixed: they can have both motor and sensory functions; each myelomere gives rise to a pair of spinal nerves, characterized by two distinct roots (ventral and dorsal root). These roots join together to form the mixed spinal nerve directed towards the periphery. These roots are important because they underline the functionality of the spine: the ventral root is motor, while the dorsal root is sensory. At the level of the roots we distinguish motor and sensory neurons, but soon as they join it become mixed (both motor and sensory). The ventral root (motor) is given by axons derived from neurons coming from the ventral part of the grey matter of the spinal cord, exiting the spinal cord, and then joining the posterior root. At this level we find the ganglion annexed to the posterior horn: this is where we find the first-order sensory neurons of the spinal cord (neurons that first receive sensory information coming from the periphery and forward that to the spinal cord). Within this ganglion, we find a particular type of neuron, the pseudounipolar neuron: it has a roundish soma and a T-shaped axon (it bifurcates), with the centrifugal part going to the periphery and the centripetal part going towards the center. At this level, we find neurons that first receive sensory information: the centrifugal branch arrives at the periphery and receives sensory information (pressure, pain..), then the signals are forwarded to the soma and go through the centripetal branch that will reach the spinal cord. In the dorsal part of the spinal cord, we’ll find the second-order sensory neurons, receiving sensory info carried by the first-order sensory neurons. 7 Above is a specimen of the spinal cord, sampled from a cadaver: we can observe the opened dural sac, the upper extremity, and the lower extremity with the cauda equina. We can also see the emergence of the rootlets delimiting one myelomere from the other. Above is a transverse section of the neural tube and the spinal cord that shows which parts derived from the basal plate, becoming motor and which from the alar plate, becoming sensory. We can observe a hollow central cavity, the inner mantle layer and the outer marginal layer. This morphology is reflected in the spinal cord: a central canal, the inner grey matter (from the mantle layer), and the outer white matter (from the marginal layer). The typical shape of the grey matter at the level of the spinal cord is that of a butterfly or the letter H. We can identify several superficial landmarks, indicated by the black arrows in the picture above, important both for their function and pathologies related. We can identify the ventral surface thanks to the presence of a deep sulcus at the midline, the anterior median fissure (1), quite prominent, going from the outer edge towards the center of the spinal cord; it’s called a fissure because it presents a gap between its two lateral margins. It represents the midline surface landmark of the spinal cord. Behind it, we find a small bridge of white matter connecting the white matter of the two antimeres, known as anterior white commissure (2). Behind the white commissure, we find the grey commissure, connecting the grey matter of the two antimeres. As we move laterally we can identify two narrow sulci, the anterior lateral sulci (3) where spinal rootlets of the anterior or motor root of the spinal nerve emerge. Moving laterally and dorsally, we identify quite a large region of white matter that is delimited posteriorly by the posterior lateral sulci (5); at this level, we find the entrance of the sensory rootlets of the spinal nerve. Pay attention to the terminology: from the anterior lateral sulcus we have the emergence of the rootlets of the spinal nerves, while posterior lateral sulcus we have their entrance; this is an indication of the directionality of signals carried by these axons. Motor axons originate from the motor parts of the spinal cord and the signal is carried from the center to the periphery (for example, to induce muscle contraction). On the other hand, the 8 sensory information is received by the sensory axons that travel from the ganglion to the spinal cord and enter it reaching the sensory region deriving from the alar plate (blue). Moving to the posterior midline, we find a subtle sulcus, the posterior median sulcus (4): it’s less wide than the fissure and most of its profile is obliterated (not visible); the two edges are attached by the septum posterius, a septum of glial cells hiding the sulcus. It continues all the way to the grey commissure. These surface landmarks are important to recognize the anatomy of this district and to divide external white matter found in the spinal cord. Here we identify three pairs of funiculi (‘rows’) of white matter, fibers that move upwards (vertical course) from the spinal cord or, inversely, from the brain to the spinal cord. The term funiculus refers to the most vertically oriented fibers within the spinal cord. 1. anterior funiculi (a), extending between the anterior median fissure and the anterior lateral sulcus 2. lateral funiculi (b), between the anterior and posterior lateral sulcus 3. posterior funiculi (c), between the posterior lateral sulcus and posterior median sulcus In each funiculus, we find bundles of axons carrying specific signals from/to the spinal cord, either sensory or motor: knowing which bundle is bound to which funiculus, allows us to clearly localize specific functions within the white matter. The grey matter has a butterfly shape; the two halves of the myelomeres are perfectly symmetrical and each half is known as an antimere. Morphologically speaking, we can identify three main components: 1. anterior or ventral horn 2. posterior or dorsal horn 9 3. commissure, the bridge connecting one antimere to the other. In the anterior horns (red square), we find motor neurons, whereas in the posterior horns (blue square) we find sensory neurons. Motor axons emerge from the anterior horn, forming the ventral motor root of the spinal nerve; in the posterior horn, we find the neurons receiving sensory information, coming from the dorsal root entering at the level of the posterior lateral sulcus. At the level of the commissure, or bridge of grey matter, we find the remnant of the lumen of the neural tube, called the central canal, lined by ependymal cells, working as an interface between the cavity and the rest of the tissue. We can now observe how this morphology varies along the length of the spine: this is important because in the nervous system, structure, and function are strongly related, and the different morphologies reflect the different functions carried by each segment. In the upper cervical region, we find a consistent marginal layer, but as we move downwards it becomes less and less evident; the size of the white matter decreases progressively as we move inferiorly. This is because we mainly find vertical axons (ascending or descending): in the cervical myomere, we’ll find descending axons sending information from the brain to the cervical, thoracic, lumbar, sacral myelomeres and ascending axons coming from the sacral, lumbar and thoracic segment. At the level of the lumbar myomeres, we’ll only find descending axons sending information to the lumbar and sacral myelomeres and ascending axons from the sacral myelomere. Also, the morphology of the grey matter changes considerably through different sections: we always find the butterfly / H-shape but at the level of the cervical and lumbar myelomeres we see that the ventral horn is quite wide, whereas in thoracic myelomeres it's narrow. This difference is due to the innervation of the upper and lower limbs starting from the cervical and lumbar myelomeres. Closer to the midline of the ventral horns there’s mainly innervation of axial structures such as the thorax, neck and abdomen; the more lateral part of the ventral horns is reserved to the innervation of more distal structures, such as the limbs. Since there are no appendices to innervate in the thoracic region, we need fewer neurons, the ones innervating the axial district, so the ventral horn of thoracic myelomeres narrows down considerably. Starting from the lumbar myelomeres, we have the innervation of the lower limbs: we thus find a large lateral part of the ventral horn, innervating the distal parts of the body (lower limbs). In the sacral myelomeres, we find a wide ventral horn because of the innervation of the pelvic muscles. Even though there are no limbs to innervate in this region, the pelvic floor is a complex system of muscles that requires a large number of neurons, for the control of sphincters. 10 One last important feature is the presence of a third horn of grey matter, specifically found at the level of the thoracic myelomeres, in particular from T1 to T10. You can observe it represented in the image: it's actually a third horn of the matter found only in the thoracic region, between the posterior and the anterior horns. It's required for visceral innervation, particularly for orthosympathetic innervation of the viscera. The parasympathetic division, for the resting state of the body, is controlled mainly by the vagus nerve at the level of the brainstem. To distinguish between the cervical and the lumbar myelomeres, as the morphology of the grey matter is quite similar, we have to look at the white matter: upper myelomeres, such as the cervical ones, have much more white matter than the lower myelomeres. Thus, considering the morphology of the grey matter and the amount of white matter around it, we can understand what section we are examining. In the image, we can observe sections at the: cervical level (a), thoracic level (b), lumbar level (c), and sacral level (d). At this link, we can appreciate some sections of the spinal cord: https://pathpresenter.net/public/display?token=bca1eaba 11