Plasma Membrane - Lipid Bilayer PDF
Document Details
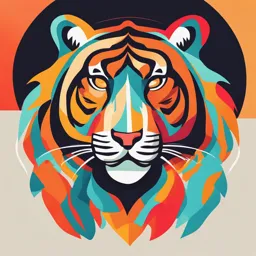
Uploaded by PerfectBowenite
Geisinger Commonwealth School of Medicine
Tags
Summary
This document provides an overview of the plasma membrane, focusing on its lipid bilayer structure and functions. It explains the roles of different lipids like phospholipids, sphingolipids, glycolipids, and cholesterol, and how they impact membrane fluidity and asymmetry. The different categories of plasma lipids and their features are detailed.
Full Transcript
Module 1: Plasma membrane - lipid bilayer Slide 2: The learning objectives are to explain the functions of the plasma membrane, describe how phospholipids, sphingolipids, glycolipids, and cholesterol are arranged in a bilayer to influence the membrane fluidity, understand the concept of cell membran...
Module 1: Plasma membrane - lipid bilayer Slide 2: The learning objectives are to explain the functions of the plasma membrane, describe how phospholipids, sphingolipids, glycolipids, and cholesterol are arranged in a bilayer to influence the membrane fluidity, understand the concept of cell membrane fluidity, describe the membrane asymmetry, and outline its importance, explain the organization and function of lipid rafts. Slide 3: What are the functions of the plasma membrane? 1. Protection: the analogy between the plasma membrane and a city fortress is self-explanatory and goes beyond the protective function. 2. Selectively permeable “filtration”: only selective cargo is allowed to pass through. 3. Structural role: the plasma membrane is attached to the cytoskeleton. 4. Communication function: it facilitates the interactions of a cell with its neighbors and the extracellular matrix. Slide 4: A foundational concept is the fluid mosaic model of the plasma membrane. In this model, there are two antiparallel sheets of phospholipids with proteins “floating” in them. The layer closer to the cytosol is the inner (cytosolic) leaflet. The layer closer to the exterior environment is the outer (non-cytosolic) leaflet. The membranes are fluid because the lipid bilayer is viscous with individual lipids that can move. The membranes are mosaic since proteins are embedded in the bilayer, resembling a mosaic. Depending upon the cell type, the proteins constitute 25-75% of the membrane mass. Slide 5: All lipids are hydrophobic. Hydrophilic molecules are water-soluble due to their polar groups, which form electrostatic and hydrogen bonds with water molecules. Hydrophobic molecules are water-insoluble. Hydrophobic (non-polar) molecules force the water molecules around them into cage-like formations, with hydrogen bonds between the surrounding water molecules (shown in Figure B). The formation of these structures decreases the entropy of the system, and is, therefore, energetically unfavorable. The entropy can increase, if the hydrophobic molecules aggregate and therefore, release some water molecules. Therefore, the clustering of the hydrophobic molecules is energetically favorable. This is why lipids in water spontaneously aggregate into micelles and bilayers. Figure A depicts that conical in shape phospholipids tend to make micelles, whereas phospholipids with cylindrical shapes tend to make a bilayer. Slide 6: There are three categories of plasma lipids: phospholipids, glycolipids, and sterols. Glycerol, sphingosine, or cholesterol are the backbone of these molecules. [Note: phospholipids have a phosphate bound to glycerol (glycerophospholipids) or choline (sphingolipids); glycolipids have a sugar group instead a phosphate group.] Slide 7: Phospholipids and glycolipids contain a polar part (head), and a nonpolar part (tail). Due to this duality, the molecules are called “amphipathic”. The most common membrane lipids, the phospholipids, have one head and two hydrophobic tails. Typically, the tails are fatty acids of approximately 14 to 24 carbon atoms. One of the two tails usually has one or more cis-double (unsaturated) bonds (see the figure on the side). The cis-double bond creates a bending (kink). This bending results in less packed molecules and a bilayer with increased fluidity (i.e., more space between the lipid molecules). The length of the tails also influences the fluidity of the membranes - the longer the tails, the lower the fluidity. Slide 8: In phospholipids, the polar head is frequently glycerol and a phosphate molecule. There are also two non- polar tails of fatty acid (hydrocarbon) chains. The four major types of phospholipids in plasma membranes are depicted on the slide. Notice that among these, only phosphatidylserine has a net negative charge at physiological pH (the others are neutral). The fourth phospholipid, sphingomyelin, has alcohol choline in its head group and contains sphingosine instead of glycerol as a backbone. Sphingomyelin is abundant in the myelin cover that surrounds the axons of nerve cells. (More on myelin: https://www.youtube.com/watch?v=eoVQ09W_Qlo&t=49s.) Slide 9: The most abundant phospholipids are those containing glycerol as a backbone. The figure demonstrates the variety of molecules that form the polar head of the glycerophospholipids. One of these molecules is inositol as a part of phosphatidylinositol, which although a minor component in the cytosolic (inner) leaflet, plays a major signaling role. Slide 10: The lipid bilayer behaves as a two-dimensional fluid. Within the two dimensions, the lipid molecules: (1) diffuse laterally, (2) rotate about their long axis, (3) their tails swing from side to side, and (4) contract. Phospholipids also migrate (albeit rarely) from one side to the other side of the bilayer facilitated by flippases, floppases, and scramblases (5). An example of such movement is the movement of phosphatidylserine which is usually confined to the inner (cytosolic) leaflet but is redistributed to the outer leaflet during apoptosis, a mechanism of programmed cell death. NB: Despite their names, flippases, floppases, and scramblases are not considered to be enzymes in some textbooks, whereas in some peer-reviewed literature, they are referred to as enzymes. Slide 11: The lipid bilayer can change from a fluid-like state to a gel-like state at a freezing point. The transition to a gel-like state is easier if the hydrocarbon chains (i.e., the tails) are long or do not have cis- double bonds (as in saturated chains). These features make the lipid bilayer tightly packed, less fluid, and less permeable to small molecules. A bilayer made only of hydrocarbon unsaturated chains would behave like a liquid because there will be more bending (of the tails) and more space between the molecules. The fluidity of a membrane is also affected by the levels of cholesterol in it. In the membrane, the hydroxyl groups of the cholesterol molecules are positioned close to the head groups of the phospholipids. The steroid rings are inserted between the tails and immobilize the hydrocarbon chains closest to the head groups. Cholesterol is a bidirectional regulator of membrane fluidity. The direction, in which cholesterol influences membrane fluidity depends upon the temperature. At low temperatures, the tails of the lipids would tend to cluster together (crystallize). However, the presence of cholesterol counteracts this clustering by preventing the lipid tails from packing close together. Therefore, at low temperatures, cholesterol increases membrane fluidity. At high temperatures, the lipid molecules and their tails tend to move more and increase their distance between each other. However, cholesterol with its rigid rings restricts such movements and therefore, decreases membrane fluidity. Due to the bidirectional effects of cholesterol, its presence inhibits the phase transition between a liquid-like state and a solid-like state; thus, cholesterol stabilizes the fluidity level of the membranes. Slide 12: In addition to phospholipids and cholesterol, the plasma membrane contains glycolipids. The glycolipids are on the outer leaflet, with their carbohydrate parts (moieties) facing the extracellular space. These molecules self-associate by hydrogen bonds between the sugars and by van der Waals forces between the hydrocarbon chains. The glycolipids perform different functions. One function is the protection against external low pH and degradation enzymes. Another function is cell recognition. For example, some glycolipids serve as receptors. The ganglioside GM1 is a cell surface receptor and is recognized by the bacterial toxin causing the diarrhea of cholera. Slide 13: The gangliosides are glycolipids that carry oligosaccharides with one or more sialic acids. The head groups have negative charges at a pH of 7. These molecules are most abundant in neurons. They are involved in cell-cell recognition, adhesion, and signaling. They are also connected to many pathologies. [Examples: in the Guillain-Barré syndrome that could lead to acute quadriplegia, there is an autoimmune response to cell surface gangliosides. Influenza A viruses recognize sialic acid residues on the surface of host cells as receptors and use these molecules to invade the host cells. Some lysosomal storage diseases result in the accumulation of glycosphingolipids, including gangliosides. Infantile-onset symptomatic epilepsy syndrome (with developmental stagnation and blindness) found in Old Order Amish pedigree, is caused by a mutation in GM3 synthase. GM3 in cell surface microdomains might be involved in insulin resistance in type 2 diabetes. Alzheimer’s disease might be initiated by aggregation of amyloid-β peptides caused by gangliosides.] Slide 14: A prominent characteristic of the plasma membrane is its asymmetry: the lipid and protein composition of the inner and outer layers differ. In most human cells, phosphatidylcholine and sphingomyelin are in the outer leaflet, whereas phosphatidylserine, phosphatidylethanolamine, and phosphatidylinositol are in the inner leaflet. The asymmetry is created through flippases, floppases, scramblases, and rare/slow non-assisted flip-flop movements of lipids. Glycoproteins and glycolipids in the plasma membrane are always oriented with their carbohydrates projecting into the extracellular environment. Peripheral membrane proteins are usually attached to the inner membrane leaflet. The importance of maintaining the plasma membrane asymmetry is demonstrated during apoptosis (i.e., cell death) when phosphatidylserine molecules translocate from the inner to the outer leaflet. The macrophages recognize this exposure as a signal to phagocytose (engulf) the dying cells. Additionally, the differentially distributed membrane molecules may provide binding sites for non-membrane molecules and thus, impact cell structure, recognition, and signaling. Slide 15: The asymmetry of the plasma membrane is also generated by lipid rafts. The lipid rafts are subdomains of the plasma membrane that contain high concentrations of cholesterol, sphingolipids, and specific proteins. The lipid rafts are held together by the long hydrocarbon chains of the sphingolipids. In lipid rafts, the two monolayers communicate through these long lipid tails. The proteins in the rafts also have longer transmembrane domains spanning the bilayers. Some proteins accumulate in the rafts for future transport in small vesicles. Other proteins function as receptors that respond to extracellular signals. Slide 16: Putting it together biological membranes are a continuous double layer of lipids with embedded proteins the lipid bilayer is a two-dimensional fluid: lipid molecules diffuse within the plane membrane lipid molecules are amphipathic and there are three major classes of membrane lipids: phospholipids, cholesterol, and glycolipids in water, phospholipids assemble into bilayers, which form sealed compartments that reseal if torn the lipid compositions of the inner and outer monolayers differ, reflecting their different functions the head groups of some lipids form docking sites for cytosolic proteins the fluidity of the membrane is affected by several factors, such as cholesterol content, length of the fatty acid tails and the number of double bonds in them, headgroup charge, and structure