Fundamentals of Clinical Pharmacology 2024-2025 PDF
Document Details
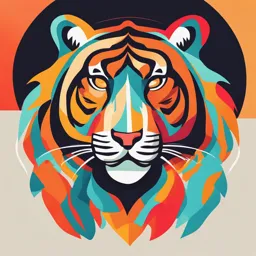
Uploaded by MemorableSurrealism
Case Western Reserve University School of Medicine
Michael D. Altose MD PhD
Tags
Related
- Clinical Pharmacology III Week 3 PDF
- Intravenous Opioid Agonists and Antagonists PDF
- Basic and Clinical Pharmacology 12th Ed. PDF
- Fundamentals of Clinical Pharmacology 2024-2025 PDF
- Kabarak University DCMED 228 Clinical Pharmacology II Past Paper PDF 2020/2021
- Basic & Clinical Pharmacology (2017) PDF
Summary
These are lecture notes on Fundamentals of Clinical Pharmacology from the Certified Anesthesiologist Assistant Program at the CWRU School of Medicine. The notes cover various aspects of the subject matter, including pharmacokinetics, distribution, and elimination related to drug mechanisms and their actions.
Full Transcript
ANES 475/476 – PHARMACOLOGY FOR ANESTHESIOLOGIST ASSISTANTS CWRU School of Medicine, Certified Anesthesiologist Assistant Program Michael D. Altose MD PhD, 2024-2025 Fundamentals of Clinical Pha...
ANES 475/476 – PHARMACOLOGY FOR ANESTHESIOLOGIST ASSISTANTS CWRU School of Medicine, Certified Anesthesiologist Assistant Program Michael D. Altose MD PhD, 2024-2025 Fundamentals of Clinical Pharmacology Suggested Reading: M&M Ch 7 PPAP Ch 2 1. Pharmacokinetics – what the body does to the drug How does concentration in blood vary with dose, time Absorption – How the drug gets in o Route and bioavailability ▪ Intravenous, intramuscular (reliable but slower), transcutaneous, subcutaneous (less reliable) ▪ Oral, rectal ▪ Sublingual – direct absorption into systemic venous system (NTG, fentanyl) Avoids portal circulation, no hepatic first-pass effect because it bypasses hepatic metabolism ▪ Intrathecal, epidural, perineural ▪ Inhalational o First-pass metabolism occurs before drug reaches systemic circulation / target site ▪ Liver: very significant for enteral (oral, rectal) administration Avoided with IV, IM, SL routes (see https://icp.org.nz/oral-availability/first-pass-metabolism)` ▪ Lung (some fentanyl uptake, propofol metabolism) ▪ Plasma esterases / pseudocholinesterase o Bioavailability = fraction of drug that reaches systemic circulation ▪ =1.0 (100%) for parenteral (non-GI, like IV, IM, SQ) drugs – no hepatic first-pass effect (see https://icp.org.nz/oral-availability/oral-availability) Distribution / redistribution Four Classic Tissue Groups o Distribution of cardiac output VRG Muscle Fat VP % Body Wt 10 50 20 20 ▪ VRG (vessel-rich group) gets most cardiac output even % CO 75 19 6 ~0 though it makes up a small percentage of body weight ▪ VP (vessel-poor group) gets almost no cardiac output even though it makes up about 20% of body weight ▪ Lipophilic drugs rapidly equilibrate into CNS tissue o Redistribution ▪ Single doses of lipophilic drugs have a short CNS duration of action as they redistribute to peripheral tissues ▪ Multiple doses → increasing peripheral tissue concentrations Longer apparent duration of action Decrease in plasma concentration becomes dependent on drug elimination from the body instead of redistribution o Drugs bound to protein – unavailable for uptake by an organ (or excretion) ▪ Albumin – binds most acidic / neutral drugs, some basic drugs (ex: benzodiazepines, SSRIs) ▪ Alpha-1-acid glycoprotein – binds most basic drugs ▪ Low protein levels (malnutrition, chronic illness) → higher free drug levels o Two-compartment model: ▪ Central compartment (Plasma + VRG) and peripheral compartment ▪ Initial spike in serum concentration after injection Quick decline – distribution phase (α phase) Slower decline – elimination phase (β phase) ▪ Some drugs are better modeled with a 3-compartment model (2 peripheral compartments) ANES 475/476 Fundamentals of Clinical Pharmacology ~ 2024-2025 Michael D. Altose – p. 2 Transfer/Transport – Drugs must get into the cell o Cell membrane is a lipid bilayer – small lipophilic drugs pass through easily o Nonspecific transmembrane proteins make a hydrophilic channel for hydrophilic drugs ▪ Except in CNS: hydrophilic drugs must undergo active transport o Passive transport (movement of drug down a concentration gradient) ▪ Generally limited by blood flow (not lipid solubility) ▪ This means the drug will diffuse across as quickly as it can be delivered to the tissue o Facilitated diffusion = need carrier proteins, but without energy requirement o Active transport = carrier proteins use energy to move drug, even against a concentration gradient ▪ Both lipophilic and hydrophilic drugs need active transport to deal with concentration gradients Quick Acid-Base refresher Acids: H+ + A– ↔ HA Acidic (H+) environments drive Bases: H+ + B ↔ HB+ these equations to the RIGHT - Why do we care? Because CHARGED (ionized) species don’t cross membranes very well. Most drugs work best when they are non-ionized - pKa tells you the pH where the ionization occurs (“50-50 point”) - If pKa = 6 and the pH is 2 (much more acidic) Then the “excess protons” will drive the equation to the RIGHT - If pKa = 6 and the pH is 7.4, the equation will be driven to the LEFT Example: Etomidate Base (H+ + B ↔ HB+) pKa = 4.2 (pH blood = 7.4) 99% non-ionized in the blood Example: Propofol Acid (H+ + A– ↔ HA) pKa = 11 (pH blood = 7.4) 99% non-ionized in the blood (also see https://icp.org.nz/ph-and-pharmacokinetics/effect-of-altering-ph) ANES 475/476 Fundamentals of Clinical Pharmacology ~ 2024-2025 Michael D. Altose – p. 3 Elimination o Biotransformation = alteration of the drug via a metabolic process (usually in liver) ▪ Most drugs need to be hydrophilic (water soluble) for excretion Some drugs do NOT require biotransformation in order to be excreted in the urine ▪ Phase I: oxidation, reduction, hydrolysis → increase polarity of molecule to make the drug water soluble for excretion in urine Cytochrome P-450 (CYP) enzyme system catalyzes most Phase I reactions Induction: CYP activity increases with ongoing drug exposure – can alter metabolism of other medications that are metabolized by the same CYP enzyme subtype o Ex: CYP 3A4: Acetaminophen, Bupivacaine, Diazepam, Digoxin, Diltiazem, Fentanyl, Lidocaine, Midazolam, Nicardipine, Omeprazole, Ropivacaine, Statins, Sufentanil, Verapamil, Warfarin o Ex: St John’s Wort induces CYP 3A4 causing diminished drug effect CYP activity can be inhibited when drugs compete for the same CYP subtype o Ex: cimetidine inhibits metabolism of meperidine, propranolol, diazepam o Ex: Grapefruit juice inhibits CYP 3A4 causing exaggerated drug effects ▪ Phase II: conjugation with a polar substance (ex: attachment of a glucuronate, acetate, glutathione group) → water soluble for excretion in urine ▪ Many CYP as well as other Phase I and Phase II polymorphisms exist. Ex: pseudocholinesterase deficiency ▪ Neonates thru 1 yr of age have diminished Phase I and Phase II activities o Hepatic drug clearance: volume of blood that the liver could cleanse of drug in a given amount of time ▪ Equals hepatic blood flow × extraction ratio Hepatic blood flow: depends on cardiac output, blood pressure Hepatic extraction ratio: fraction of drug removed from the blood as it passes through the liver o Some drugs are more easily “extracted” by the liver (and metabolized) than others – see table High extraction ratio = “flow limited” (ex: etomidate, propofol) o Hepatic clearance will be almost the same as hepatic blood flow. That is, a change in hepatic blood flow produces a nearly proportional change in clearance o Low CO states will show diminished hepatic elimination o Clearance remains unchanged unless metabolic capacity (Vm) is severely compromised Low extraction ratio = “capacity limited” (ex: thiopental, diazepam) o Hepatic clearance will not be significantly affected by changes in hepatic blood flow o Clearance is limited by the fact that the liver can only handle a fraction of the drug it sees, even under ideal conditions o Changes in metabolic capacity (Vm) will produce a nearly proportional effect on clearance ANES 475/476 Fundamentals of Clinical Pharmacology ~ 2024-2025 Michael D. Altose – p. 4 o Renal drug clearance ▪ Autoregulation maintains constant renal blood flow over a wide range of cardiac output ▪ But renal clearance is only a small fraction of renal blood flow, due to protein binding (only unbound drug will be filtered into the kidneys for excretion) ▪ Renal tubular active transporters allow renal clearance to approach RBF ▪ Pts with decreased renal function may need altered drug dosing to avoid accumulation of parent compounds and metabolites You should be aware of the drugs that have significant renal excretion (especially those with a *) Hepatic Extraction Ratios Drugs with significant renal excretion Low Intermediate High Aminoglycosides Pancuronium* Diazepam Alfentanil Alprenolol Atenolol Penicillins* Lorazepam Methohexital Bupivacaine Cephalosporins* Procainamide Methadone Midazolam Diltiazem Phenytoin Vecuronium Fentanyl Digoxin* Pyridostigmine Rocuronium Ketamine Edrophonium Quinolones Theophylline Lidocaine Nadolol Rocuronium* Thiopental Meperidine Neostigmine* Sugammadex* Metoprolol Morphine Naloxone Nifedipine Propofol Propranolol Sufentanil Other pharmacokinetic concepts o First-order kinetics: a fixed percentage (fraction, "k") of existing drug is removed Elimination per unit of time. 1 ▪ The AMOUNT removed depends on the 1st Order 0.9 serum levels. Zero Order 0.8 ▪ The FRACTION removed does NOT 0.7 depend on serum levels. Concentration 0.6 ▪ If 10% is removed per minute, 0.5 k = 0.1 min-1 0.4 o Zero-order kinetics: fixed amount of drug 0.3 removed per unit of time 0.2 ▪ The AMOUNT removed per unit time is 0.1 INDEPENDENT of serum levels. 0 ▪ The FRACTION removed per unit time 0 5 10 15 20 25 30 35 40 45 50 will VARY depending on serum levels. Time ▪ Found in certain drugs (ex: phenytoin, alcohol) ▪ “Saturation Kinetics” - Can occur when excess amounts of drug saturate the capacity of metabolic enzymes or transport systems. (ex: digoxin) (see https://icp.org.nz/saturable-drug-metabolism/cp-time-effect) o Half-life: time for serum concentration to change by a factor of 2 ▪ Drugs with shorter half-life require more frequent re-dosing ▪ (see https://icp.org.nz/the-half-life/the-half-life) ▪ Half-life (t1/2) = ln 2 / k so after 5 half-lives, the process is 97% complete o Volume of distribution (Vd): quantifies the extent of drug distribution ▪ Describes the capacity of tissues for absorbing a certain drug ▪ Depends on tissue mass and the affinity of that drug for the tissue ANES 475/476 Fundamentals of Clinical Pharmacology ~ 2024-2025 Michael D. Altose – p. 5 ▪ Numeric index of the extent of drug distribution, describing behavior of the drug in the body (see https://icp.org.nz/volume-of-distribution/simple-container-model) ▪ In general, lipophilic drugs have larger Vd than hydrophilic drugs ▪ Vd = amount of drug given / serum concentration So loading dose = Vd × target concentration o Elimination clearance (ClE)= theoretical volume of blood from which drug is completely and irreversibly removed in a unit of time ▪ Best describes the physiologic process of drug elimination ▪ Can be calculated from the declining blood levels observed after an IV injection ▪ Clearance = dose given / Area under the concentration vs time curve o Elimination half-life (t1/2β): amount of time it takes for the amount of drug in the body to decrease by a factor of 2 ▪ It depends on both distribution and elimination ▪ t1/2β = ln 2 × (Vd / ClE) Ex: midazolam has a prolonged t1/2β in elderly – clearance is unchanged but Vd is increased. It is NOT due to decreased metabolism Ex: pancuronium has prolonged t1/2β in renal insufficiency – clearance (renal elimination) is decreased, but no change in Vd ▪ Does not say anything about termination of EFFECT (which depends on serum concentration), only elimination of drug from the body (which includes all the other compartments and tissues) ▪ Effects of most IV anesthetics have waned long before even one elimination half-life has been completed 2. Pharmacodynamics = “What the drug does to the body” Defining Terms (see https://icp.org.nz/pharmacodynamics/linear-vs-log-graphs) o ED50: Dose required to produce a specific effect in 50% of the population (like MAC) o LD50: Dose required to cause death (or toxicity) in 50% of the population o Therapeutic Index (LD50 / ED50) – a measure of safety (room for error) ▪ Our drugs often have a two-tailed therapeutic index ▪ There are both low (awareness) and high (overdose) “toxicities” to avoid Note: the figures below are NOT dose-response curves, they are population curves ANES 475/476 Fundamentals of Clinical Pharmacology ~ 2024-2025 Michael D. Altose – p. 6 Drug-receptor interactions, dose-response curves o Receptor Model: Drug + Receptor ↔ Drug–Receptor → Effect ▪ Agonist (X): binds to a receptor (R) and produces an effect ▪ Remember there are billions of drug-receptor pairs continuously binding and unbinding thousands of times per second ▪ Potency: how much drug you need to get an effect. Usually due to differing affinity for receptor On the dose-response curve, drug X is more potent than drug Z ▪ Efficacy: max degree of effect a drug can cause On the dose-response curve, drug X has more efficacy than drug Y ▪ Partial agonist: produces a lower maximal response than a full agonist That is, lower efficacy Competitively inhibits the response produced by a full agonist ▪ Redundancy: there is generally an excess of receptors Maximum effect typically occurs at far below maximum receptor binding Ex: Neuromuscular junction only needs 25% of receptors to be bound by ACh for clinical strength o That’s why you need >75% blockage to get clinical weakness! ▪ Tolerance: diminished response to a drug due to chronic exposure Cellular tolerance (adaptation), Enzyme induction (change in metabolism) Depletion of neurotransmitters ▪ Tachyphylaxis: Acute tolerance after only a few doses o Antagonists: bind to receptor without producing an effect Competitive antagonist (Y) Binds reversibly to the same binding site Effect can be overcome by increasing concentration of agonist. Decreases agonist potency, but maximum effect (efficacy) is not changed. ▪ Non-competitive antagonist (Z) Some bind irreversibly to the receptor Some bind to a separate binding site on the same receptor (allosteric). Reduce both potency and efficacy Cannot be completely overcome by increasing concentration of agonist See https://icp.org.nz/drug-interactions/pharmacodynamic ANES 475/476 Fundamentals of Clinical Pharmacology ~ 2024-2025 Michael D. Altose – p. 7 CLINICAL MODULES FOR SELF STUDY Module #1 An eight-year-old boy presents for elective tonsillectomy. He is healthy and his past medical history is unremarkable. He is crying and clinging to his mother as you do your preoperative evaluation. You plan to administer midazolam as a sedative prior to taking him back to the operating room. 1. How can midazolam be adminstered? What are the +’s and –’s of each route? 2. Why does a dose of IV midazolam start to work so quickly? 3. How long does its effect last? Why? Where does the drug go? 4. In an 80 kg adult, midazolam has Vd of ~80 L. But total body water (TBW) is only 42 L! How is this explained? ◼ If “light sedation” occurs at a serum concentration of 50 ng/mL, what loading dose should be given to the patient? (Dose / Volume = concentration) ◼ Is this the dose you would expect to use for light sedation? Why or why not? 5. Midazolam redistributes to other compartments via first-order kinetics. What does that mean? ◼ How can we understand kredist = 0.07 min-1? What does this mean? ◼ When do we see zero-order kinetics in pharmacology? ◼ The half-life of midazolam is 10 minutes. What does this mean? 6. Midazolam is water-soluble (in the vial) but becomes lipid-soluble after injection ◼ Show this, knowing that midazolam is a BASE and its pKa = 6.2 ◼ How does this compare with propofol, an ACID with pKa = 11? ◼ Why would we want drugs to be lipid-soluble in the bloodstream? Module #2 Consider a patient who is having general anesthesia for lumbar spine surgery. Anesthesia is maintained with propofol and rocuronium infusions. 1. What is the role of the liver in elimination of drugs? 2. How could you maintain a constant level of anesthesia (plasma conc. of 4 mcg/mL) for 8 hours using propofol? ◼ Single bolus? Multiple boluses? Constant infusion? Variable infusion? 3. Would you approach rocuronium the same way as propofol? What might be different? Module #3 A patient under your care receives a 10-fold overdose of IV fentanyl. 1. Is fentanyl a “safe” drug? How can this be described using pharmacodynamic terms? 2. On one set of labeled axes, draw the dose response curves for (1) fentanyl, (2) morphine, (3) acetaminophen, and (4) ketorolac. You can assume: ◼ 100 mcg fentanyl = 10 mg morphine = 1000 mg acetaminophen = 10 mg ketorolac ◼ (1) and (2) can achieve the same maximum clinical effect ◼ (3) and (4) can achieve the same maximum clinical effect, which is smaller than (1) and (2) 3. A patient in PACU has been given too much fentanyl, and he is treated with naloxone (Narcan), a competitive antagonist. Now he is in pain and needs more fentanyl. ◼ Would the additional fentanyl still work to control his pain?