Intravenous Opioid Agonists and Antagonists PDF
Document Details
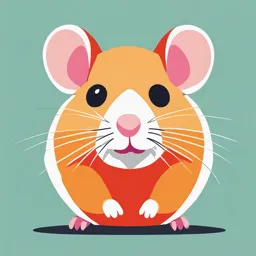
Uploaded by ComfortingMothman3162
University of Florida
Takahito Ogura and Talmage D. Egan
Tags
Summary
This chapter provides a review of the basic and clinical pharmacology of intravenous opioids, focusing on their use in anesthesiology and perioperative medicine. It explores the unique characteristics of commonly used opioid drugs and details the principles of rational drug selection and administration. Contemporary developments in the field are also discussed.
Full Transcript
17 Intravenous Opioid Agonists and Antagonists TAKAHIRO OGURA AND TALMAGE D. EGAN CHAPTER OUTLINE Historical Perspective Basic Pharmacology Structure-Activity Mechanism Metabolism Clinical Pharmacology Pharmacokinetics Pharmacodynamics Therapeutic Effects Adverse Effects Drug Interactions Special Po...
17 Intravenous Opioid Agonists and Antagonists TAKAHIRO OGURA AND TALMAGE D. EGAN CHAPTER OUTLINE Historical Perspective Basic Pharmacology Structure-Activity Mechanism Metabolism Clinical Pharmacology Pharmacokinetics Pharmacodynamics Therapeutic Effects Adverse Effects Drug Interactions Special Populations Hepatic Failure Kidney Failure Gender Age Obesity Hemorrhagic Shock Unique Features of Individual Agents Codeine Morphine Methadone Fentanyl Alfentanil Sufentanil Remifentanil Opioid Agonist-Antagonists and Pure Antagonists Nalbuphine and Others Naloxone and Naltrexone Clinical Application Common Clinical Indications Rational Drug Selection and Administration Computerized Delivery Methods Emerging Developments Opioids and Cancer Recurrence Novel µ-Opioid Agonists Opioid Antagonists for Ileus and Constipation Therapy Opioid-Induced Hyperalgesia and Acute Tolerance Opioid Overdose Epidemic Historical Perspective The white “latex” juice of the poppy plant is the source of more than 20 opiate alkaloids. Opium, a word derived from the Greek word for juice, is the brownish residue observed after the poppy’s juice is desiccated. Opiate is the older term classically used in pharmacology to mean a drug derived from opium. Opioid, a more modern term, is used to designate all substances, both natural and synthetic, that bind to opioid receptors (including antagonists). Opium and its derivatives have been known for millennia to relieve pain. Laudanum, or tincture of opium (a mixture of opium and alcohol), was used as early as the 1600s as an analgesic. Sir Christopher Wren, the acclaimed Englishman of arts and letters, was the first to inject opium into a living organism using a hollow feather quill as the delivery system in 1659. Sertürner, a German pharmacist, isolated morphine from opium in 1805. Having initially called morphine “somniferum” (after the Latin botanical name Papaver somniferum— the poppy that brings sleep), the name was later changed to morphine, alluding to Morpheus, the Greek god of dreams. Opium and its derivatives have sometimes been the basis of international conflict. The Opium Wars of the 1800s were fought between China and the Western powers in large part in response to Western importation of opium into China. The opium houses of 19th century China, where opium was freely available for sale and consumption, illustrated the frightening societal consequences of large-scale drug abuse. Recognition of these problems in the United States eventually culminated in the Harrison Narcotics Tax Act of 1914 that criminalized narcotic possession. The prevalence of addiction clinics and drug-related violence and crime in modern society emphasizes the chronicity of the societal difficulties that stem from this drug class and others. The ongoing opioid abuse epidemic in the United States is a recent reflection of the complexity and recalcitrance of the problems associated with opioid addiction despite nearly a century of research devoted to the conundrum. Despite their abuse potential, opioids play an indispensable role in the practice of medicine, especially anesthesiology, critical care, and pain management. A sound understanding of opioid pharmacology, including both basic science and clinical aspects, is critical for the safe and effective use of these important drugs. This chapter focuses almost exclusively on intravenous opioids used perioperatively. 332 Downloaded for Vicente Gonzalez ([email protected]) at Florida International University from ClinicalKey.com by Elsevier on April 29, 2024. For personal use only. No other uses without permission. Copyright ©2024. Elsevier Inc. All rights reserved. CHAPTER 17 Intravenous Opioid Agonists and Antagonists Abstract Keywords This chapter reviews the basic and clinical pharmacology of intravenous opioids in the context of anesthesiology and perioperative medicine. Unique features of commonly used opioids are discussed and the principles underlying rational drug selection and administration are outlined. Emerging developments of contermporary interest are briefly examined. Opioids morphine fentanyl remifentanil codeine naloxone naltrexone biased opioids opioid epidemic Downloaded for Vicente Gonzalez ([email protected]) at Florida International University from ClinicalKey.com by Elsevier on April 29, 2024. For personal use only. No other uses without permission. Copyright ©2024. Elsevier Inc. All rights reserved. 332.e1 CHAPTER 17 HO Oxymorphone (agonist) H3CO O N N CH3 H HO HO CH3 O O HO Codeine Morphine O OH Address CH3CH2OC CH3CH2C N O N CH3 Meperidine O 333 Naltrexone (antagonist) HO O H Intravenous Opioid Agonists and Antagonists N CH2CH2 Fentanyl NH+ CH3 O OH NH+ Message Fig. 17.2 Structures of prototypical opioid ligands (i.e., oxymorphone and naltrexone) highlighting regions involved in conferring selectivity (i.e., addressing) shaded in blue, and efficacy (i.e., messaging) shaded in pink. (Adapted with permission from Huang W, Manglik A, Venkatakrishnan AJ, et al. Structural insights into micro-opioid receptor activation. Nature 2015;524:315–321.) Fig. 17.1 The molecular structures of morphine, codeine, meperidine and fentanyl. Note that codeine is a simple modification of morphine (as are many other opiates); fentanyl and its congeners are more complex modifications of meperidine, a phenylpeperidine derivative. Basic Pharmacology Structure-Activity The opioids of clinical interest in anesthesiology share many structural features. Morphine, the principal active compound derived from opium, is a benzylisoquinoline alkaloid; the benzylisoquinoline structural backbone is present in many important naturally occurring drugs including papaverine, tubocurarine, and morphine. Morphine’s benzylisoquinoline based structure is shown in Fig. 17.1. Many commonly used semisynthetic opioids are created by simple modification of the morphine molecule. Codeine, for example, is the 3-methyl derivative of morphine. Similarly, hydromorphone, hydrocodone, and oxycodone are also synthesized by relatively simple modifications of morphine. More complex alteration of the morphine molecular skeleton results in mixed agonist-antagonists such as nalbuphine and even pure competitive antagonists such as naloxone. Some of the morphine derivatives have chiral centers and thus are typically synthesized as racemic mixtures of two enantiomers; only the levorotatory enantiomer is significantly active at the opioid receptor. The naturally occurring, stereospecific enzymatic machinery in the poppy plant produces morphine only in the levorotatory form. The fentanyl series of opioids are chemically related to meperidine. Meperidine is the first completely synthetic opioid and can be regarded as the prototype clinical phenylpiperidine. As shown in Fig. 17.1, fentanyl is a simple modification of the basic phenylpiperidine structure found within meperidine; the other commonly used fentanyl congeners such as alfentanil and sufentanil are somewhat more complex versions of the same phenylpiperidine skeleton. As these drugs have no chiral center and therefore exist in a single form, the pharmacologic complexities of stereochemistry do not apply.1 As shown in Fig. 17.2, evidence from x-ray crystallography investigations and other techniques suggests that distinct parts of the opioid agonist or antagonist molecule confer specificity (i.e., addressing) and efficacy (i.e., messaging).2 Agonists and antagonists have similar addressing segments in their structures; their action at the receptor is determined by the messaging portion of the molecule (see Chapter 1). As outlined in Table 17.1, opioids share many physicochemical features in common, although some individual drugs have unique features. In general, opioids are highly lipid soluble, weak bases that are highly protein bound and largely ionized (protonated) at physiologic pH. Opioid physicochemical properties are thought to have important implications on their clinical behavior. For example, highly lipid soluble, relatively unbound, un-ionized molecules such as alfentanil and remifentanil have a shorter latency to peak effect after bolus injection presumably because of their more rapid transfer across cellular membranes. Mechanism Opioids produce their main pharmacologic effects by interacting with opioid receptors. Investigation of opioid receptor genetics, structure, and function over the past several decades has greatly enhanced understanding of opioid pharmacology. Cloning of the opioid receptors, first accomplished in rodents and subsequently in humans, was an important first step in eventually elucidating opioid receptor structure and function. Using the rat µ-opioid receptor complementary DNA sequence as a guide, investigators were able to identify its human homolog, describing the amino acid sequence, strong binding affinity for an endogenous opioid ligand (i.e., enkephalin) and chromosomal assignment (i.e., chromosome 6).3 Opioid receptors are typical of the G-protein–coupled family of receptors widely found in biology (e.g., β-adrenergic, dopaminergic, among others). Like other G-protein–coupled receptors, opioid receptors have seven transmembrane portions, intracellular and extracellular loops, an extracellular N-terminus, and an intracellular C-terminus. There is considerable amino acid sequence homology among the various opioid receptor subtypes; most of the nonhomologous sequences occur in the extracellular portions of the protein. The extracellular domains are thought to be important in discriminating between the various receptor ligands. Expression of cloned opioid receptors in cultured cells has facilitated analysis of the intracellular signal transduction mechanisms activated by opioid receptors.4 As shown in Fig. 17.3, binding Downloaded for Vicente Gonzalez ([email protected]) at Florida International University from ClinicalKey.com by Elsevier on April 29, 2024. For personal use only. No other uses without permission. Copyright ©2024. Elsevier Inc. All rights reserved. 334 SE C T I O N II Nervous System TABLE Selected Opioid Physicochemical and Pharmacokinetic Parameters 17.1 Parameter Morphine pKa Fentanyl 8.0 % Un-ionized at pH 7.4 8.4 8.0 Remifentanil 6.5 7.1 20 90 67? 1.4 813 1778 145 17.9 20-40 84 93 92 Octanol-H2O partition coefficient Diffusible fraction (%) Alfentanil 65 years of age). These combined pharmacokinetic and pharmacodynamic changes mandate Downloaded for Vicente Gonzalez ([email protected]) at Florida International University from ClinicalKey.com by Elsevier on April 29, 2024. For personal use only. No other uses without permission. Copyright ©2024. Elsevier Inc. All rights reserved. 342 SE C T I O N II Nervous System Normal Kidneys Central clearance (L/min) 5 Plasma concentration (nmol/L) 1000 100 Morphine 3-glucuronide 3 2 1 0 10 20 40 Morphine 0 4 8 12 16 60 80 100 80 100 Age (yr) Morphine 6-glucuronide 0 30 20 24 EC50 (ng/mL) Time (hr) Kidney Failure 1000 Plasma concentration (nmol/L) 4 20 10 Morphine 3-glucuronide 0 100 40 60 Age (yr) 10 0 20 Morphine 6-glucuronide Morphine 0 4 8 12 16 20 24 Fig. 17.12 The influence of age on the clinical pharmacology of remifentanil. Although there is considerable variability, in general, older subjects have a lower central clearance and a higher potency (lower EC50). EC50, Effective concentration for 50% of maximal effect. (Adapted with permission from Minto CF, Schnider TW, Egan TD, et al. Influence of age and gender on the pharmacokinetics and pharmacodynamics of remifentanil. I. Model development. Anesthesiology. 1997;86:10–23.) Time (hr) a reduction in remifentanil dosage by at least 50% or more in seniors. Similar dosage reductions are also prudent for other µ-agonists. Obesity Although detailed information is unavailable for many commonly used opioids, evidence from the study of drugs introduced more recently suggests that body weight is an important factor influencing the clinical pharmacology of opioids. Opioid pharmacokinetic parameters, especially clearance, are more closely related to lean body mass than to total body weight (TBW). In practical terms, this means that morbidly obese patients do indeed require a higher dosage than lean patients to achieve the same target concentration, but the very obese patients do not need nearly as much as would be suggested by their TBW.51 For example, as illustrated in the pharmacokinetic simulation shown in Fig. 17.13, a TBW- based dosing scheme in an obese patient results in excessive remifentanil effect site concentrations 35 Remifentanil Ce (ng mL–1) Fig. 17.11 The pharmacokinetics of morphine and its metabolites in normal volunteers versus kidney failure patients. Note the significant accumulation of the metabolites in renal failure. (Adapted with permission from Osborne R, Joel S, Grebenik K, et al. The pharmacokinetics of morphine and morphine glucuronides in kidney failure. Clin Pharmacol Ther. 1993;54: 158–167.) 30 25 20 Obese patient - TBW dosing 15 Lean patient - TBW dosing 10 Lean patient - LBM dosing 5 Obese patient - LBM dosing 0 0 15 30 45 60 75 90 105 120 135 150 Time (min) Fig. 17.13 A pharmacokinetic simulation illustrating the consequences of calculating the remifentanil dosage based on total body weight (TBW) or lean body mass (LBM) in obese and lean patients (1-µg/kg bolus injection followed by an infusion of 0.5 µg/kg per minutes for 15 minutes and 0.25 µg/kg per minute for an additional 105 minutes). Note that TBWbased dosing in an obese patient results in dramatically higher concentrations. Ce, Effect site concentration. (Adapted with permission from Egan TD, Huizinga B, Gupta SK, et al. Remifentanil pharmacokinetics in obese versus lean patients. Anesthesiology. 1998;89:562–573.) Downloaded for Vicente Gonzalez ([email protected]) at Florida International University from ClinicalKey.com by Elsevier on April 29, 2024. For personal use only. No other uses without permission. Copyright ©2024. Elsevier Inc. All rights reserved. Intravenous Opioid Agonists and Antagonists 343 Reduction in Peak Pain compared with dosing calculation based on lean body mass.52 In contrast, for lean patients, the concentrations that result from TBW-based dosing regimens are not much greater than those based on lean body mass. Recent evidence based on a large pharmacokinetic modeling study has confirmed these general impressions regarding remifentanil dosing guidelines in obese patients.53 Hemorrhagic Shock Although the data are from animal studies, it is clear that severe hemorrhage significantly alters the pharmacokinetic behavior of opioids. Both the clearance and distribution parameters are affected. As shown in the pharmacokinetic simulation presented in Fig. 17.14, subjects experiencing severe hemorrhage can be expected to achieve blood concentrations that are approximately double those observed in control subjects when they receive identical doses.54 Even a drug that does not require delivery to a metabolic organ like remifentanil is affected, although the effect is not as dramatic.55 There is clear evidence from case reports that these observations apply to human pharmacology as well.56 Unique Features of Individual Agents Codeine Codeine, while not commonly used intraoperatively, has special importance among opioids because of a well-characterized pharmacogenomic nuance associated with it. Codeine is actually a prodrug that is metabolized in part by O-demethylation into morphine, a metabolic process mediated by the liver P450 isoform CYP 2D6.57 As demonstrated in Fig. 17.15, patients who lack CYP 2D6 because of deletion, frame shift or splice mutations (approximately 10% of the Caucasian population), or those whose CYP 2D6 is inhibited (e.g., patients taking quinidine) do not respond normally to codeine even though they exhibit a normal response to morphine.58,59 Interestingly, Asians have less CYP 2D6 activity and thus may be an ethnic group particularly resistant to codeine therapy.60 Conversely, certain ethnic groups (e.g., Ethiopians) are thought to be particularly susceptible to the effects of codeine because they P = 0.85 0 e A pharmacokinetic simulation illustrating the influence of severe hemorrhagic shock on the disposition of fentanyl. Animals in shock achieve substantially higher blood concentrations when receiving the same fentanyl dose. Cp, concentration in the plasma. (Adapted with permission from Egan TD, Kuramkote S, Gong G, et al. Fentanyl pharmacokinetics in hemorrhagic shock: a porcine model. Anesthesiology 1999;91:156–166.) in Fig. 17.14 2 or ph Shock ne Time (min) Control 4 M 90 ei 80 o 70 od 60 C 50 ac eb 40 Pl 30 e 20 in 10 or ph 0 ne 0.4 P = 0.048 6 ei 2.5 µg kg–1 hr –1 P = 0.018 M 1 P = 0.065 o 2 Poor metabolizers 8 od 5 Extensive metabolizers 10 C 50 µg ac eb 100 µg Pl 20 15 10 Reduction in peak visual analog pain (mm) Fentanyl Cp (ng/min) CHAPTER 17 Fig. 17.15 A volunteer study demonstrating that codeine is no more effective than placebo in patients who cannot convert codeine to morphine (i.e., poor metabolizers). Morphine retains its normal analgesic efficacy in such patients. Both codeine and morphine are effective in normal subjects (i.e., extensive metabolizers). The bars represent the median of the maximal reduction in peak pain as assessed by the cold pressor test using a visual analog scale. (Adapted with permission from Poulsen L, Brosen K, Arendt-Nielsen L, et al. Codeine and morphine in extensive and poor metabolizers of sparteine: pharmacokinetics, analgesic effect and side effects. Eur J Clin Pharmacol. 1996;51:289–295.) carry frequently duplicated, functional CYP 2D6 genes, resulting in the ultrarapid conversion of codeine to morphine.61 In addition to therapeutic failure, pharmacogenetic variants in codeine metabolism can result in tragic adverse drug reactions. Functional CYP 2D6 gene duplication has recently been implicated in fatalities among infants breastfed by mothers who are ultrarapid metabolizers.62 Deaths in children who received codeine after tonsillectomy and who, upon postmortem genotyping, were confirmed to be ultrarapid metabolizers have been reported; morphine levels in these children at autopsy were well beyond the toxic threshold.63 Ironically, codeine was presumably prescribed to these children to avoid the respiratory depression typically associated with more powerful opioids. More than 80 different alleles for CYP 2D6 have now been identified, creating numerous possible phenotypes for codeine metabolism. Consensus guidelines for the interpretation of CYP 2D6 diplotypes enable the clinician to categorize patients into one of four categories, reflecting a range of codeine conversion to morphine, from none to extensive.64 Unfortunately, for the vast majority of patients who are candidates for codeine therapy, their diplotype is unknown at the time of treatment. Until an era dawns when most patients have their entire genome sequenced so that the pharmacogenetic information is available to the clinician at the point of care as part of a “personalized medicine” approach to health care, codeine’s decline in popularity among prescribers is likely to continue. Morphine Morphine is the prototype opioid against which all newcomers are evaluated. Despite a century of opioid research, there is no Downloaded for Vicente Gonzalez ([email protected]) at Florida International University from ClinicalKey.com by Elsevier on April 29, 2024. For personal use only. No other uses without permission. Copyright ©2024. Elsevier Inc. All rights reserved. SE C T I O N II Nervous System evidence that any synthetic opioid is more effective in controlling pain than nature’s morphine. Were it not for the histamine release–mediated hypotension observed intraoperatively with high doses of morphine, it is conceivable that fentanyl would not have replaced morphine as the most commonly used opioid in anesthesia practice. An important detail relating to morphine’s clinical pharmacology is its slow onset time. Morphine’s pKa renders it almost completely ionized at physiologic pH. This property, combined with its low lipid solubility, presumably accounts for morphine’s prolonged latency to peak effect; morphine penetrates the CNS slowly. This feature has both advantages and disadvantages. The prolonged latency to peak effect (see Fig. 17.7A) means that morphine is less likely to cause acute respiratory depression after bolus injection of typical analgesic doses compared with the more rapid-acting drugs because the relationship between minute ventilation and the partial pressure of carbon dioxide changes more slowly (thus there is more time for carbon dioxide to rise and sustain ventilatory drive). On the other hand, the slow onset time means that clinicians are more likely to inappropriately “stack” multiple morphine doses in a patient experiencing severe pain, thus creating the potential for a toxic “overshoot.”65 Morphine’s active metabolite, M6G, also has very important clinical implications. Although conversion to M6G accounts for only 10% of morphine’s metabolism, M6G contributes to morphine’s analgesic effects even in patients with normal renal function, particularly with longer-term use. Because of morphine’s high hepatic extraction ratio, the bioavailability of orally administered morphine is significantly lower than after parenteral injection. The hepatic first-pass effect on orally administered morphine results in high M6G levels; in fact, it appears that M6G may be the primary active compound when morphine is administered orally.66 Accumulation of M6G to potentially toxic levels in dialysis patients is another important implication of this active metabolite. Methadone Available in both intravenous and oral forms, racemic methadone has long been a mainstay in the treatment of opioid addicts because its long-acting pharmacokinetic profile makes the development of an acute withdrawal syndrome less likely (i.e., methadone maintenance therapy). The N-methyl-D-aspartate (NMDA) receptor antagonism action of the dextrorotatory isomer of methadone may also serve to attenuate the effects of opioid tolerance and withdrawal syndrome.67,68 Methadone is also increasingly used in the treatment of patients with chronic pain, particularly cancer-related pain.69 Methadone’s propensity to prolong the QT interval of the electrocardiogram by blocking the delayed rectifier potassium ion channel may contribute to increased mortality in this patient population.70 Fentanyl As the original fentanyl congener, its clinical application is well entrenched and highly diverse. Perhaps the most unique aspect of fentanyl’s clinical application is the numerous ways in which fentanyl is delivered. In addition to the intravenous method, fentanyl has also been delivered by transdermal, transmucosal, transnasal, and transpulmonary routes. Oral transmucosal delivery of fentanyl citrate results in the faster achievement of higher peak levels than when the same dose is swallowed as seen in Fig. 17.16.71 It is the avoidance of the first-pass effect through transmucosal absorption that results in this significantly greater bioavailability. That oral transmucosal Fentanyl plasma concentration (ng/mL) 344 3 2 1 0 0 2 4 6 Time after administration (hr) OTFC Oral solution Fig. 17.16 A volunteer study contrasting the bioavailability and peak plasma concentrations of an identical dose (15 mcg/kg) of fentanyl delivered either orally or through the oral mucosa (i.e., oral transmucosal fentanyl [OTFC]). Note that the transmucosal approach yields higher peak concentrations earlier. (Adapted with permission from Streisand JB, Varvel JR, Stanski DR, et al. Absorption and bioavailability of oral transmucosal fentanyl citrate. Anesthesiology. 1991;75:223–229.) delivery of fentanyl citrate is noninvasive and rapid in onset has made it a successful therapy for breakthrough pain in opioid-tolerant cancer patients, often in combination with a transdermal fentanyl patch. Alfentanil Alfentanil was the first opioid to be administered almost exclusively by continuous infusion. Because of its relatively short terminal half-life, alfentanil was originally thought to be an opioid with a rapid offset of effect after termination of a continuous infusion.72 Subsequent advances in pharmacokinetic theory (i.e., the CSHT) proved this assertion to be false.14 However, alfentanil is in fact a short-acting drug after a single bolus injection because of its high diffusible fraction; it reaches peak effect site concentrations quickly and then begins to decline (see earlier “Pharmacokinetics” and Fig. 17.7). Alfentanil illustrates how a drug can exhibit different pharmacokinetic profiles depending on the method of administration (i.e., bolus vs. continuous infusion). Relative to fentanyl and sufentanil, hepatic metabolism of alfentanil is more unpredictable because of the significant interindividual variability of hepatic CYP3A4, the primary enzyme responsible for alfentanil biotransformation. Because alfentanil has a relatively low hepatic extraction ratio compared with fentanyl, it is more vulnerable to variations in CYP 3A4 activity.73 Sufentanil Sufentanil’s distinguishing feature is that it is the most potent opioid commonly used in anesthesia practice. Because it is more intrinsically efficacious at the opioid receptor, the absolute doses used are much smaller compared with other less potent drugs (e.g., 1000-fold less than morphine). Remifentanil Remifentanil is a prototype example of how specific clinical goals can be achieved by designing molecules with specialized Downloaded for Vicente Gonzalez ([email protected]) at Florida International University from ClinicalKey.com by Elsevier on April 29, 2024. For personal use only. No other uses without permission. Copyright ©2024. Elsevier Inc. All rights reserved. CHAPTER 17 O H3C CH3 O O N O Remifentanil CH3 Minor Major O N H3C O O CH3 O N H3C O CH3 O N 345 Opioid Agonist-Antagonists and Pure Antagonists O N Intravenous Opioid Agonists and Antagonists O N OH GI-90291 (inactive) H GI-94219 Fig. 17.17 Remifentanil metabolic pathway. De-esterification (i.e., ester hydrolysis) by nonspecific plasma and tissue esterases to an inactive acid metabolite (GI-90291) accounts for the vast majority of remifentanil’s metabolism. (Adapted with permission from Egan TD, Lemmens HJ, Fiset P, et al. The pharmacokinetics of the new short-acting opioid remifentanil (GI87084B) in healthy adult male volunteers. Anesthesiology. 1993;79: 881–892.) structure-activity (or structure-metabolism) relationships. As shown in Fig. 17.17, the medicinal chemists responsible for the development of remifentanil sought to produce a potent opioid that would lose its µ-receptor agonist activity upon ester hydrolysis, thereby creating an intravenous opioid with a very short-acting pharmacokinetic profile.74 The perceived unmet need driving remifentanil’s development was that the practice of anesthesia requires a degree of pharmacokinetic responsiveness unnecessary in most medical disciplines and that anesthetics (opioids included) therefore ought to be short-acting so that they can be titrated during the rapidly changing conditions of anesthesia and surgery. Compared with the currently marketed fentanyl congeners, remifentanil’s CSHT is short, on the order of about 5 minutes (Fig. 17.7C).75 Pharmacodynamically, remifentanil exhibits a short latency-to-peak effect similar to alfentanil and a potency slightly less than fentanyl (Fig. 17.7A).76 Remifentanil’s role in modern anesthesia practice is now relatively well established; its unique pharmacokinetic profile makes it possible to manipulate rapidly the degree of opioid effect in a way that could not be achieved with the previously marketed fentanyl congeners. Remifentanil is therefore perhaps best suited for cases where its responsive pharmacokinetic profile can be exploited to advantage (e.g., when rapid recovery is desirable, when the anesthetic requirement rapidly fluctuates, when opioid titration is unpredictable or difficult, when there is a substantial danger to opioid overdose, or when a “high-dose” opioid technique is advantageous but the patient is not going to be mechanically ventilated postoperatively).77 Today, remifentanil’s most common clinical application may be the provision of TIVA in combination with propofol. It is also commonly used by bolus injection when a brief pulse of opioid effect followed by rapid recovery is advantageous (e.g., in preparation for local anesthetic injection during Monitored Anesthesia Care). Because they bind to opioid receptors, the “mixed” agonistantagonists and the pure antagonists are also classified as opioids. Opioid agonist-antagonists, of which nalbuphine is perhaps the modern prototype, are structurally related to morphine but do not behave as full agonists. These drugs serve as analgesics with more limited ventilatory depression and a lower potential for dependence as they demonstrate a “ceiling effect,” producing less analgesia compared with pure agonists. The lower abuse potential was the primary perceived unmet need underlying the development of these drugs. Drugs in this category are used for the treatment of chronic pain, as well as the treatment of opioid addiction. These drugs cause some degree of competitive antagonism (i.e., withdrawal symptoms) when administered in the presence of ongoing full agonist activity (e.g., when administered after morphine and other pure agonists). Pure opioid antagonists, of which naloxone is the prototype, are complete competitive antagonists of the opioid receptor that are devoid of any intrinsic agonist activity. These pure antagonists are used in the management of acute opioid overdose and chronic abuse. Nalbuphine and Others This group of synthetic and semisynthetic opioids includes nalbuphine, pentazocine, and butorphanol; these drugs are partial agonists at the κ receptor and complete, competitive antagonists at the µ-receptor. Both the analgesic and ventilatory depressant effects of these drugs exhibit a ceiling effect when compared to morphine78 and they do not reduce MAC as powerfully as morphine or fentanyl.79 As a class, these opioid agonist-antagonists (or “partial agonists”) were developed with the hope that they would be effective analgesics but less subject to diversion and abuse. These drugs are indeed efficacious in the treatment of mild to moderate pain (and they do have less abuse potential), but the analgesic ceiling effect has limited their more widespread use. Perhaps their most common perioperative use is the reversal of excessive opioid-induced ventilatory depression (µ-antagonism) while maintaining some analgesic effect (partial κ agonism).80 As µ-antagonists, these drugs will of course precipitate a withdrawal syndrome in opioid-dependent patients81 and are thus sometimes used in the maintenance treatment of opioid addicts. Buprenorphine is a related drug that is an antagonist at the κ receptor but a partial agonist at the µ-receptor.82 Buprenorphine is increasingly used in the treatment of opioid addiction. Naloxone and Naltrexone Naloxone, a derivative of oxymorphone, is a competitive antagonist of all opioid receptor types, but its greatest affinity is for the µ-receptor.83 When administered in typical doses in the absence of ongoing opioid therapy (or abuse), naloxone has no discernable pharmacodynamic effects. When opioid effects are present, naloxone given in sufficient doses can reverse all effects mediated by opioid receptors.84 The most common clinical use of naloxone perioperatively is the reversal of excessive opioid-induced ventilatory depression.85 Its important role in this regard has merited naloxone’s inclusion on the World Health Organization’s List of Essential Medicines. Other uses include the emergency reversal of ventilatory depression in neonates whose mothers received opioids for labor analgesia, Downloaded for Vicente Gonzalez ([email protected]) at Florida International University from ClinicalKey.com by Elsevier on April 29, 2024. For personal use only. No other uses without permission. Copyright ©2024. Elsevier Inc. All rights reserved. 346 SE C T I O N II Nervous System and in cases of accidental or intended opioid overdose (e.g., heroin) in the emergency department. Naloxone is also sometimes used in much smaller doses during emergence from anesthesia to restore adequate ventilatory effort and thereby expedite extubation of the trachea. The treatment of opioid-induced pruritus using small doses is another common therapeutic application. A few nuances of naloxone’s clinical pharmacology are extremely important for safe use. Naloxone is rapidly metabolized in the liver and has a very high clearance; thus its duration of action is nearly always shorter than that of the opioid whose effects it is intended to reverse. Patients who receive naloxone for the emergency reversal of life-threatening ventilatory depression must be monitored for recurrence of the opioid effects. Repeated naloxone dosing may be necessary to sustain adequate ventilation. Also, rarely, naloxone administration has been complicated by severe adverse hemodynamic effects including pulmonary edema and even sudden death in previously healthy individuals (some tachycardia is to be expected).86 Other adverse effects include acute withdrawal syndrome, nausea, vomiting, and seizures, among others.84 In response to the opioid abuse epidemic in the United States, new naloxone delivery systems have been developed that are intended for emergency use by lay persons in the event of opioid overdose; these include nasal spray and autoinjector preparations.87,88 Several drugs closely related to naloxone have been developed for other purposes. Naltrexone, a longer-acting µ-opioid antagonist available in oral, injectable, and implantable forms, is used in the long-term management of previously detoxified opioid addicts in combination with other nonpharmacologic therapies. 89 Methylnaltrexone and alvimopan, permanently charged opioid antagonists that do not easily enter the CNS, were developed for the prevention and treatment of opioid-induced bowel dysfunction (see later text). Clinical Application Opioids play a vital role in virtually every area of anesthesia practice. In the treatment of postoperative pain, opioids are the primary therapeutic agent, whereas in most other settings in perioperative medicine opioids are therapeutic adjuncts used in combination with other drugs. Common Clinical Indications Postoperative analgesia is the longest standing indication for opioid therapy in anesthesia practice and of course dates back to the earliest days of the specialty. In the modern era, opioid administration via PCA devices is perhaps the most common mode of delivery. In recent years, opioids are increasingly combined postoperatively with various other agents such as nonsteroidal antiinflammatory drugs to increase efficacy and safety (see Chapter 16). Internationally, the most common clinical indication for opioids in anesthesia practice is their use as part of what came to be known as “balanced anesthesia.” The term connotes the use of multiple agents (e.g., inhalation agents, volatiles, neuromuscular blockers, and opioids) in smaller doses to produce the state of anesthesia. With this technique, the opioids are primarily used for their MAC reduction effects. A basic assumption underlying this balanced anesthesia approach is that the drugs used in combination mitigate the disadvantages of the individual drugs (i.e., the inhaled agents) used in higher doses as monotherapy. “High-dose opioid anesthesia,” a technique originally described for morphine in the early days of open heart surgery90 and later associated with the fentanyl congeners,91 is another common application of opioids. The original scientific underpinning of this approach was that high doses of opioids enabled the clinician to reduce the volatile agent to a minimum, thereby avoiding the direct myocardial depression and other untoward hemodynamic effects associated with high concentrations of volatile anesthetics in patients whose cardiovascular systems were already compromised. That the fentanyl drugs often produce a relative bradycardia is also thought to be helpful in mitigating against myocardial ischemia. Although the general concept is still applied, the opioid doses used today are more moderate. In this setting opioids are also administered for their possible beneficial effects in terms of cardioprotection (i.e., preconditioning). TIVA is a more recently developed and increasingly popular indication for opioids in anesthesia practice. As the name implies, this technique relies entirely on intravenous agents for the provision of general anesthesia. Most commonly, continuous infusions of remifentanil or alfentanil are combined with a propofol infusion. Both the opioid and the sedative are often delivered by targetcontrolled infusion (TCI)-enabled pumps. A clear advantage of this technique, perhaps among others, is the enhanced patient well-being in the early postoperative period, including less nausea and vomiting and often a feeling of euphoria.92 Rational Drug Selection and Administration In articulating a scientific foundation for rational opioid selection, it is most important to recognize that pharmacokinetic considerations are paramount. Indeed, the µ-agonists can be considered pharmacodynamic equals with important pharmacokinetic differences.93 In other words, for practical purposes all µ-agonists are essentially equally efficacious when equipotent concentrations are achieved, but they differ in terms of their pharmacokinetic behavior. Thus rational selection of one µ-agonist over another requires the clinician to identify the desired temporal profile of drug effect and then choose an opioid that best achieves it (within constraints such as pharmacoeconomic concerns). In selecting the appropriate opioid, among the key questions to address are as follows: How quickly must the desired opioid effect be achieved? How long must the opioid effect be maintained? How critical is it that opioid-induced ventilatory depression or sedation dissipate quickly (e.g., will the patient be mechanically ventilated postoperatively)? Is the capability to raise and lower the level of opioid effect quickly during the anesthetic critical? Will there be significant pain postoperatively that will require opioid treatment? All of these questions relate to the optimal temporal profile of opioid effect. The answers to these questions are addressed through the application of pharmacokinetic concepts. For example, when a brief pulse of opioid effect followed by rapid recovery is desired (e.g., to provide analgesia for a retrobulbar block), a bolus of remifentanil or alfentanil might be preferred. When long-lasting opioid effect is desired, such as when there will be significant postoperative pain or when the patient’s trachea will remain intubated, a fentanyl infusion is a prudent choice. In cases where it is critical that the patient be awake and alert shortly after the procedure is finished (e.g., a craniotomy in which the surgeons hope to perform a neurologic exam in the operating room immediately postoperatively), a remifentanil infusion might be advantageous. The formulation of a rational administration strategy also requires the proper application of pharmacokinetic principles. An important goal of any dosing scheme is to reach and maintain a steady-state Downloaded for Vicente Gonzalez ([email protected]) at Florida International University from ClinicalKey.com by Elsevier on April 29, 2024. For personal use only. No other uses without permission. Copyright ©2024. Elsevier Inc. All rights reserved. CHAPTER 17 level of opioid effect. To achieve a steady-state concentration in the site of action, opioids are frequently administered by continuous infusion. This is increasingly accomplished through the use of TCI technology (see later text), which requires familiarity with the appropriate pharmacokinetic model for the opioid of interest. When these systems are not available, the clinician must remember that infusions must be preceded by a bolus to come to a near–steady state in a timely fashion. Intravenous Opioid Agonists and Antagonists Emerging Developments Opioids and Cancer Recurrence Concerns have been raised recently that opioids administered as part of a general anesthetic for cancer surgery may have an adverse impact on cancer recurrence rates. This concern is supported by considerable in vitro and animal data demonstrating immunosuppressive and angiogenic effects of opioids (particularly morphine). Some early, retrospective data comparing prostate cancer recurrence rates in patients receiving standard postoperative opioid analgesia with those receiving alternative techniques (e.g., epidural pain management) generated the hypothesis that perioperative opioid use may promote cancer recurrence.96 Some more recent, larger, retrospective studies focused on other types of cancer have failed to corroborate these earlier findings,97,98 whereas other investigations have yielded supportive evidence.99 Confirmation of the hypothesis that perioperative opioid therapy promotes cancer recurrence would presumably have a major impact on the anesthesia techniques applied to cancer surgery patients, including a more prominent role for regional postoperative analgesia techniques to reduce the overall opioid load. At this stage, this hypothesis is unproven and the data must be interpreted with caution until more definitive information from ongoing controlled, randomized trials is available.100,101 Ferreting out this dilemma is a major goal of contemporary anesthesiology research. Computerized Delivery Methods Advances in pharmacologic modeling and infusion pump technology have now made it possible to administer injectable anesthetics via a computer-controlled infusion pump.94 By coding a pharmacokinetic model into a computer program and linking it to an electronic pump modified to accept computerized commands, delivery according to a drug’s specific pharmacokinetic parameters can be achieved. The physician operating a TCI system designates a target concentration to achieve rather than specifying an infusion rate. The TCI system then calculates the necessary infusion rates to achieve the targeted concentration. The system changes the infusion rates at frequent intervals (i.e., as often as every 10 seconds). Successful use of a TCI pump thus requires knowledge of the therapeutic concentrations appropriate for the specific clinical application. Computer-controlled drug delivery in the operating room is an exciting area with well-established clinical utility, although it is admittedly difficult to demonstrate obvious outcome improvements compared to traditional delivery methods. TCI administration of opioids is common for all of the fentanyl congeners. Although TCI systems are widely used in North America for research purposes, they are not yet commercially available for routine clinical use (unlike much of the rest of the world).95 Biased µ-Opioid Agonists There is evidence that the signaling pathways for opioid therapeutic and selected adverse effects may be distinct. As shown in Fig. 17.18, studies in animal models and in vitro techniques suggest Morphine K+ Adenylyl cyclase GIRK µOR Gi + ATP cAMP Analgesia 347 β-arrestin Respiratory depression Constipation Tolerance Fig. 17.18 The role of the β-arrestin pathway in µ-agonist adverse effects. Opiate-induced MOR signaling through Gi activates G-protein–gated inwardly rectifying potassium channels (GIRKs) and inhibits adenylyl cyclase, leading to analgesia. Conversely, recruitment of β-arrestin is implicated in tolerance, respiratory depression, and constipation. ATP, Adenosine triphosphate; cAMP, cyclic adenosine monophospate; Gi, G protein alpha subunit; MOR, µ-opioid receptor. (Adapted with permission from Manglik A, Lin H, Aryal DK, et al. Structure-based discovery of opioid analgesics with reduced side effects. Nature. 2016;537:185–190.) Downloaded for Vicente Gonzalez ([email protected]) at Florida International University from ClinicalKey.com by Elsevier on April 29, 2024. For personal use only. No other uses without permission. Copyright ©2024. Elsevier Inc. All rights reserved. 348 SE C T I O N II Nervous System that opioid-induced analgesia results from MOR signaling through G proteins, while many side effects, including respiratory depression, tolerance, and constipation, may be mediated by β-arrestin pathway signaling downstream of MOR agonism.102-104 That separate pathways may mediate analgesic and adverse effects of µ-agonists presents an opportunity to create molecules that are “biased” toward the G-protein pathway, thereby minimizing respiratory depression while maintaining analgesic efficacy. Using detailed knowledge of the crystal structures of the opioid receptors, medicinal chemists have taken a computational approach to identify potential candidate molecules that may have the desired biophysical properties in terms of how these molecules interact with the MOR (i.e., minimizing β-arrestin-2 signaling). In silico screening of millions of commercially available molecules resulted in identification of some candidate molecules structurally unlike currently available opioids that have intriguing clinical potential based on preclinical testing (e.g., PZM21).105 Although this drug development effort is in its infancy, there are exciting basic science and clinical implications if this line of research can be carried forward. Opioid-Induced Hyperalgesia and Acute Tolerance It is well known that long-term exposure to opioids produces tolerance to opioid effects; huge opioid doses may be needed to provide adequate perioperative analgesia in patients receiving chronic, high-dose opioid therapy.110 However, more recent evidence suggests that even brief exposure to opioids may result in an increased dose requirement. Acute tolerance and opioid-induced-hyperalgesia are pharmacologically distinct phenomena that can both result in the need for a dose escalation to maintain adequate analgesia. In general pharmacologic terms, tolerance is a decrease in the analgesic response to an opioid owing to its continued administration. Hyperalgesia, in contrast, is an increased response to a stimulus that is normally painful.111 Considered in terms of the dose-response relationship, opioid tolerance shifts the curve to the right, whereas opioid-induced hyperalgesia shifts the curve downward because of a change in pain sensitivity (Fig. 17.19).112 Both phenomena manifest clinically as a need for a higher dose to achieve the same analgesic effect. Distinguishing between the two can be therapeutically important because tolerance calls for an increased dosage, whereas opioidinduced hyperalgesia may be more prudently addressed with non-opioid analgesics and other adjuncts (i.e., because opioids exacerbate the problem). Whether acute tolerance and opioid-induced hyperalgesia play important roles in the management of perioperative patients is controversial.111 Although numerous animal studies have demonstrated the occurrence of opioid-induced hyperalgesia, the data in humans are more ambiguous.113-117 Current thinking suggests that both phenomena may coexist to some degree in certain perioperative settings.111 Risk factors for their occurrence include high opioid dose, prolonged use, and perhaps inhalation anesthesia (i.e., propofol may mitigate risk).118-120 There is increasing evidence that NMDA receptor antagonism mediated through drugs such Opioid Antagonists for Ileus and Constipation Therapy An important development in the treatment of opioid-induced adverse effects is the use of µ-receptor antagonists to treat opioidinduced postoperative ileus and constipation.106,107 Methylnaltrexone and alvimopan are µ-receptor antagonists whose physicochemical properties prevent them from crossing the blood-brain barrier in appreciable amounts. These drugs thus block the µ-receptors in the gut without antagonizing the therapeutic µ-agonist effects that are mediated in the CNS. Controlled, randomized trials have demonstrated their efficacy in the prevention and treatment of postoperative ileus and the constipation associated with chronic opioid use. A recent metaanalysis has confirmed their efficacy,108 but treatment guidelines regarding patient selection are still evolving.109 Tolerance Hyperalgesia 100 75 75 50 50 25 25 Analgesic effect 100 0 0 Rightward shift –25 Downward shift –25 –50 –50 0 1 2 Dose 3 4 0 1 2 Dose 3 4 Fig. 17.19 Tolerance versus opioid-induced-hyperalgesia in terms of the dose-effect relationship changes. Tolerance shifts the dose-response curve to the right (but does not alter pain sensitivity). Opioidinduced hyperalgesia shifts the dose-response curve downward because of increased pain sensitivity. The striped area represents the net increase in dose required to achieve 50% of the maximal analgesic effect. (Adapted with permission from Chu LF, Angst MS, Clark D. Opioid-induced hyperalgesia in humans: molecular mechanisms and clinical considerations. Clin J Pain. 2008;24:479–496.) Downloaded for Vicente Gonzalez ([email protected]) at Florida International University from ClinicalKey.com by Elsevier on April 29, 2024. For personal use only. No other uses without permission. Copyright ©2024. Elsevier Inc. All rights reserved. CHAPTER 17 11 Intravenous Opioid Agonists and Antagonists 349 Overdose Deaths Involving Opioids, by Type of Opioid, United States, 2000–2015 10 Deaths per 100,000 population 9 8 7 6 5 4 3 2 1 0 2000 2002 Any opioid 2004 2006 Heroin 2008 2010 2012 2014 Natural & semi-synthetic opioids... Other synthetic opioids (e.g., fentanyl, tramadol) Methadone Fig. 17.20 Overdose deaths involving opioids by type in the United States, 2000–2015. (Adapted from the Centers for Disease Control and Prevention National Vital Statistics database, 2016.) as ketamine may also mitigate the severity of opioid-induced hyperalgesia.121 The mechanism of opioid-induced hyperalgesia is unclear. It likely involves both central and peripheral nervous system adaptation including processes involving the NMDA receptor.112 It appears that endogenous opioid systems do not play a role in its pathogenesis,122 whereas neuroinflammation induced by activation of microglia and astrocytes may contribute to the phenomenon.123 Some evidence suggests that different opioids (i.e., morphine, fentanyl, and remifentanil) do not behave uniformly with regard to opioid-induced hyperalgesia, and that the mechanisms underlying the hyperalgesia may also involve spinal 5-hydroxytryptamine type 3 receptors.124 An especially intriguing recent observation is that opioid agonism at MOR expressed by peripheral nociceptors may trigger the pronociceptive, maladaptive changes that result in opioid-induced hyperalgesia.125 The administration of methylnaltrexone, a peripherally restricted opioid antagonist, was sufficient to block the development of opioid-induced hyperalgesia in this rodent model. Opioid Overdose Epidemic In recent years, deaths related to the abuse and diversion of both illicit and prescription opioids have skyrocketed in the United States and elsewhere as illustrated in Fig. 17.20.126 Opioid overdose now constitutes a major cause of death in certain demographic groups; opioid-related deaths now exceed deaths from motor vehicle accidents in the United States. In addition to fatalities, this pervasive pattern of prescription and illicit opioid abuse has resulted in a huge surge in admissions to opioid use disorder treatment facilities.127 The disturbing trend is at least in part thought to be due to opioid prescribing practices for acute and chronic pain conditions that may predispose some patients to addiction.128,129 It is increasingly clear that opioid prescribing patterns have been too liberal; over the decade from approximately 2000 to 2010, the number of opioid prescriptions written in the United States quadrupled.130 These copious prescribing patterns were likely driven in part by a greater emphasis on the treatment of pain by professional societies and accreditation bodies (e.g., pain as the “fifth vital sign”). There is increasing recognition that the risk of opioid addiction stems primarily from the drugs, rather than from some obviously identifiable risk factor in the patient.130 The epidemic is now termed a national emergency in the United States,131 prompting federal and state government authorities to enact legislation and set aside funding to support research, prevention, and treatment of the problem.132,133 State-approved, pharmacybased naloxone dispensing (without a physician’s prescription) for patients filling opioid prescriptions is a notable example of the efforts supported by such legislation.134 In addition, professional societies and the United States Centers for Disease Control and Prevention have produced new guidelines for opioid prescribing.135,136 This is currently an area of intense public discussion and medical investigation. Downloaded for Vicente Gonzalez ([email protected]) at Florida International University from ClinicalKey.com by Elsevier on April 29, 2024. For personal use only. No other uses without permission. Copyright ©2024. Elsevier Inc. All rights reserved. 350 SE C T I O N II Nervous System Key Points Most of the clinically useful opioids are chemical modifications of morphine (e.g., hydromorphone, codeine, oxycodone) or meperidine (e.g., the fentanyl family of opioids). Opioids produce their primary effects by interacting with opioid receptors. Existing in three distinct types (i.e., µ, κ, and δ), these receptors are coupled with G proteins that, when activated by drugs or endogenous ligands (e.g., β-endorphins), produce inhibitory effects that hyperpolarize the cell and thereby attenuate nociceptive impulses. For certain opioids, the metabolic pathway has very important clinical implications. M6G and normeperidine are active metabolites that accumulate in renal failure, potentially causing toxicity. Remifentanil’s high clearance because of esterase metabolism results in an extremely short duration of action. Opioids can be differentiated pharmacokinetically by their latency to peak effect after bolus administration, the time required to reach a steady state after beginning an infusion, and the time required to achieve a specified level of drug concentration decline after stopping a continuous infusion (i.e., CSHT). Pharmacodynamically, the primary therapeutic effects for the perioperative use of opioids are analgesia and sedation. The most dangerous adverse effect in nonventilated patients is depression of ventilation. Other opioid effects can be observed in virtually every organ system, in part because of the wide distribution of opioid receptors. The most important drug interaction for opioids is the synergism for both therapeutic and adverse effects that results when opioids Key References Egan TD, Lemmens HJ, Fiset P, et al. The pharmacokinetics of the new short-acting opioid remifentanil (GI87084B) in healthy adult male volunteers. Anesthesiology. 1993;79:881–892. This human volunteer study first described the unique pharmacokinetic profile of remifentanil, the esterase metabolized fentanyl congener that became the first true “ultrashort-acting” opioid. In combination with propofol, remifentanil revolutionized the practice of TIVA and has become a mainstay of modern anesthesia practice, particularly in regions where intravenous anesthesia techniques are popular. (Ref. 75). Matthes HW, Maldonado R, Simonin F, et al. Loss of morphine-induced analgesia, reward effect and withdrawal symptoms in mice lacking the mu-opioid-receptor gene. Nature. 1996;383:819–823. Using sitedirected mutagenesis techniques, these investigators demonstrated that µ-receptor knockout mice failed to exhibit therapeutic effects to morphine but were otherwise phenotypically normal, confirming the importance of the µ-receptor in opioid pharmacology. The µ-receptor knockouts also showed no reward or withdrawal behavior or respiratory depression with morphine administration. (Ref. 8). McEwan AI, Smith C, Dyar O, et al. Isoflurane minimum alveolar concentration reduction by fentanyl. Anesthesiology. 1993;78:864–869. This study quantitatively characterized the extent of isoflurane MAC reduction by fentanyl in humans, thus serving as a prototype pharmacodynamic drug interaction study. The concept of reducing the dose requirement of volatile anesthetics with opioids is one of the most important and widely applied concepts in anesthetic pharmacology. (Ref. 42). Osborne R, Joel S, Trew D, et al. Morphine and metabolite behavior after different routes of morphine administration: demonstration of the importance of the active metabolite morphine-6-glucuronide. Clin Pharmacol Ther. 1990;47:12–19. This human volunteer study confirmed are combined with sedatives (e.g., volatile anesthetics and propofol). These “MAC-reducing” effects are a fundamental concept underpinning modern anesthesia. When treating certain special populations (e.g., the elderly, patients with severe hepatic or renal disease, morbid obesity, severe bleeding), significant dosage adjustments are necessary. The most common clinical applications of opioids in perioperative medicine include postoperative pain therapy, “balanced anesthesia,” and total intravenous anesthesia. The rational selection of opioids for perioperative use focuses on pharmacokinetic considerations. The commonly used perioperative opioids can be considered pharmacodynamic equals with important pharmacokinetic differences (e.g., differences relating to the onset and offset of effect). TCI of opioids is an important new delivery model. The influence of perioperative opioid use on cancer recurrence after oncologic operations, the treatment and prevention of opioid-induced ileus with poorly absorbable opioid antagonists, the search for “biased” opioid agonists with less potential for ventilatory depression, and the phenomena of acute tolerance and opioid-induced hyperalgesia are among the most important emerging developments and areas of investigation in opioid pharmacology. Opioid addiction and abuse is a major public health problem that has reached epidemic proportions, particularly in the United States. This public health crisis is an area of intense public interest and research activity. the importance of M6G as an active morphine metabolite, particularly after oral administration. Subsequent investigation has established M6G’s role in patients with renal failure or in patients receiving longer-term morphine therapy. (Ref. 66). Poulsen L, Brosen K, Arendt-Nielsen L, et al. Codeine and morphine in extensive and poor metabolizers of sparteine: pharmacokinetics, analgesic effect and side effects. Eur J Clin Pharmacol. 1996;51:289–295. This human volunteer study demonstrated the importance of pharmacogenetic factors in determining the response to codeine, a prodrug of morphine. “Poor metabolizers” do not convert codeine to morphine in significant amounts and thus have no analgesic response to codeine, although they respond to morphine normally. This polymorphism relating to codeine metabolism is perhaps the best-described pharmacogenetic issue in this area of therapeutics and serves as a prototype in understanding the potential clinical impact of these genetic nuances. (Ref. 57). Shafer SL, Varvel JR. Pharmacokinetics, pharmacodynamics, and rational opioid selection. Anesthesiology. 1991;74:53–63. This simulation study demonstrated the importance of pharmacokinetic considerations in rational opioid selection for perioperative use. The context sensitive half-time was an outgrowth of this work. Two key points of this article are that opioid behavior is quite different for bolus administration compared with continuous infusion and that pharmacokinetic simulation is necessary to understand the implications of these pharmacokinetic differences. (Ref. 14). Wang JB, Johnson PS, Persico AM, et al. Human mu opiate receptor. cDNA and genomic clones, pharmacologic characterization and chromosomal assignment. FEBS Lett. 1994;338:217–222. These investigators first cloned the human µ-receptor and identified its chromosomal location, launching the era of genomic research in opioid pharmacology. (Ref. 3). Downloaded for Vicente Gonzalez ([email protected]) at Florida International University from ClinicalKey.com by Elsevier on April 29, 2024. For personal use only. No other uses without permission. Copyright ©2024. Elsevier Inc. All rights reserved. CHAPTER 17 References 1. Egan TD. Stereochemistry and anesthetic pharmacology: joining hands with the medicinal chemists. Anesth Analg. 1996;83:447–450. 2. Huang W, Manglik A, Venkatakrishnan AJ, et al. Structural insights into micro-opioid receptor activation. Nature. 2015;524:315–321. 3. Wang JB, Johnson PS, Persico AM, et al. Human mu opiate receptor. cDNA and genomic clones, pharmacologic characterization and chromosomal assignment. FEBS Lett. 1994;338:217–222. 4. Minami M, Satoh M. Molecular biology of the opioid receptors: structures, functions and distributions. Neurosci Res. 1995;23:121–145. 5. Pan L, Xu J, Yu R, et al. Identification and characterization of six new alternatively spliced variants of the human mu opioid receptor gene, Oprm. Neuroscience. 2005;133:209–220. 6. Matthies BK, Franklin KB. Formalin pain is expressed in decerebrate rats but not attenuated by morphine. Pain. 1992;51:199–206. 7. Becerra L, Harter K, Gonzalez RG, et al. Functional magnetic resonance imaging measures of the effects of morphine on central nervous system circuitry in opioid-naive healthy volunteers. Anesth Analg. 2006;103:208–216. 8. Matthes HW, Maldonado R, Simonin F, et al. Loss of morphineinduced analgesia, reward effect and withdrawal symptoms in mice lacking the mu-opioid-receptor gene. Nature. 1996;383:819–823. 9. Sora I, Takahashi N, Funada M, et al. Opiate receptor knockout mice define mu receptor roles in endogenous nociceptive responses and morphine-induced analgesia. Proc Natl Acad Sci USA. 1997;94:1544–1549. 10. Dahan A, Sarton E, Teppema L, et al. Anesthetic potency and influence of morphine and sevoflurane on respiration in mu-opioid receptor knockout mice. Anesthesiology. 2001;94:824–832. 11. Rubinstein M, Mogil JS, Japon M, et al. Absence of opioid stressinduced analgesia in mice lacking beta-endorphin by site-directed mutagenesis. Proc Natl Acad Sci USA. 1996;93:3995–4000. 12. Hutchinson MR, Northcutt AL, Hiranita T, et al. Opioid activation of toll-like receptor 4 contributes to drug reinforcement. J Neurosci. 2012;32:11187–11200. 13. Bosilkovska M, Walder B, Besson M, et al. Analgesics in patients with hepatic impairment: pharmacology and clinical implications. Drugs. 2012;72:1645–1669. 14. Shafer SL, Varvel JR. Pharmacokinetics, pharmacodynamics, and rational opioid selection. Anesthesiology. 1991;74:53–63. 15. Lotsch J, Skarke C, Schmidt H, et al. Pharmacokinetic modeling to predict morphine and morphine-6-glucuronide plasma concentrations in healthy young volunteers. Clin Pharmacol Ther. 2002;72:151–162. 16. Lotsch J, Skarke C, Schmidt H, et al. The transfer half-life of morphine6-glucuronide from plasma to effect site assessed by pupil size measurement in healthy volunteers. Anesthesiology. 2001;95:1329–1338. 17. Drover DR, Angst MS, Valle M, et al. Input characteristics and bioavailability after administration of immediate and a new extendedrelease formulation of hydromorphone in healthy volunteers. Anesthesiology. 2002;97:827–836. 18. Hill JL, Zacny JP. Comparing the subjective, psychomotor, and physiological effects of intravenous hydromorphone and morphine in healthy volunteers. Psychopharmacology (Berl). 2000;152:31–39. 19. Scott JC, Stanski DR. Decreased fentanyl and alfentanil dose requirements with age. A simultaneous pharmacokinetic and pharmacodynamic evaluation. J Pharmacol Exp Ther. 1987;240:159–166. 20. Hudson RJ, Bergstrom RG, Thomson IR, et al. Pharmacokinetics of sufentanil in patients undergoing abdominal aortic surgery. Anesthesiology. 1989;70:426–431. 21. Minto CF, Schnider TW, Egan TD, et al. Influence of age and gender on the pharmacokinetics and pharmacodynamics of remifentanil. I. Model development. Anesthesiology. 1997;86:10–23. 22. Hughes MA, Glass PS, Jacobs JR. Context-sensitive half-time in multicompartment pharmacokinetic models for intravenous anesthetic drugs [see comments]. Anesthesiology. 1992;76:334–341. 23. Youngs EJ, Shafer SL. Pharmacokinetic parameters relevant to recovery from opioids. Anesthesiology. 1994;81:833–842. Intravenous Opioid Agonists and Antagonists 351 24. Hug CC Jr. Does opioid “anesthesia” exist? Anesthesiology. 1990;73:1–4. 25. Romberg R, Sarton E, Teppema L, et al. Comparison of morphine6-glucuronide and morphine on respiratory depressant and antinociceptive responses in wild type and mu-opioid receptor deficient mice. Br J Anaesth. 2003;91:862–870. 26. Miller JR, Zuperku EJ, Stuth EAE, et al. A subregion of the parabrachial nucleus partially mediates respiratory rate depression from intravenous remifentanil in young and adult rabbits. Anesthesiology. 2017;127: 502–514. 27. Levitt ES, Abdala AP, Paton JFR, et al. µ-Opioid receptor activation hyperpolarizes respiratory-controlling Kölliker–Fuse neurons and suppresses post-inspiratory drive. J Physiol. 2015;593:4453–4469. 28. Gross JB. When you breathe IN you inspire, when you DON’T breathe, you…expire: new insights regarding opioid-induced ventilatory depression. Anesthesiology. 2003;99:767–770. 29. Pattinson KT. Opioids and the control of respiration. Br J Anaesth. 2008;100:747–758. 30. Forrest WH Jr, Bellville JW. The effect of sleep plus morphine on the respiratory response to carbon dioxide. Anesthesiology. 1964;25: 137–141. 31. Laubie M, Schmitt H, Vincent M. Vagal bradycardia produced by microinjections of morphine-like drugs into the nucleus ambiguus in anaesthetized dogs. Eur J Pharmacol. 1979;59:287–291. 32. Reitan JA, Stengert KB, Wymore ML, et al. Central vagal control of fentanyl-induced bradycardia during halothane anesthesia. Anesth Analg. 1978;57:31–36. 33. Bennett JA, Abrams JT, Van Riper DF, et al. Difficult or impossible ventilation after sufentanil-induced anesthesia is caused primarily by vocal cord closure. Anesthesiology. 1997;87:1070–1074. 34. Streisand JB, Bailey PL, LeMaire L, et al. Fentanyl-induced rigidity and unconsciousness in human volunteers. Incidence, duration, and plasma concentrations. Anesthesiology. 1993;78:629–634. 35. Apfel CC, Laara E, Koivuranta M, et al. A simplified risk score for predicting postoperative nausea and vomiting: conclusions from cross-validations between two centers. Anesthesiology. 1999;91:693–700. 36. Apfel CC, Korttila K, Abdalla M, et al. A factorial trial of six interventions for the prevention of postoperative nausea and vomiting. N Engl J Med. 2004;350:2441–2451. 37. Radnay PA, Duncalf D, Novakovic M, et al. Common bile duct pressure changes after fentanyl, morphine, meperidine, butorphanol, and naloxone. Anesth Analg. 1984;63:441–444. 38. Dray A, Metsch R. Inhibition of urinary bladder contractions by a spinal action of morphine and other opioids. J Pharmacol Exp Ther. 1984;231:254–260. 39. Dray A, Metsch R. Spinal opioid receptors and inhibition of urinary bladder motility in vivo. Neurosci Lett. 1984;47:81–84. 40. Borner C, Warnick B, Smida M, et al. Mechanisms of opioidmediated inhibition of human T cell receptor signaling. J Immunol. 2009;183:882–889. 41. Bouillon T, Bruhn J, Radu-Radulescu L, et al. Non-steady state analysis of the pharmacokinetic interaction between propofol and remifentanil. Anesthesiology. 2002;97:1350–1362. 42. McEwan AI, Smith C, Dyar O, et al. Isoflurane minimum alveolar concentration reduction by fentanyl. Anesthesiology. 1993;78:864–869. 43. Bailey PL, Pace NL, Ashburn MA, et al. Frequent hypoxemia and apnea after sedation with midazolam and fentanyl. Anesthesiology. 1990;73:826–830. 44. Vuyk J, Lim T, Engbers FH, et al. The pharmacodynamic interaction of propofol and alfentanil during lower abdominal surgery in women. Anesthesiology. 1995;83:8–22. 45. Kern SE, Xie G, White JL, et al. A response surface analysis of propofol-remifentanil pharmacodynamic interaction in volunteers. Anesthesiology. 2004;100:1373–1381. 46. Rudin A, Lundberg JF, Hammarlund-Udenaes M, et al. Morphine metabolism after major liver surgery. Anesth Analg. 2007;104:1409– 1414. Downloaded for Vicente Gonzalez ([email protected]) at Florida International University from ClinicalKey.com by Elsevier on April 29, 2024. For personal use only. No other uses without permission. Copyright ©2024. Elsevier Inc. All rights reserved. 352 SE C T I O N II Nervous System 47. Dershwitz M, Hoke JF, Rosow CE, et al. Pharmacokinetics and pharmacodynamics of remifentanil in volunteer subjects with severe liver disease. Anesthesiology. 1996;84:812–820. 48. Hoke JF, Shlugman D, Dershwitz M, et al. Pharmacokinetics and pharmacodynamics of remifentanil in persons with renal failure compared with healthy volunteers. Anesthesiology. 1997;87:533–541. 49. Osborne R, Joel S, Grebenik K, et al. The pharmacokinetics of morphine and morphine glucuronides in kidney failure. Clin Pharmacol Ther. 1993;54:158–167. 50. Sarton E, Olofsen E, Romberg R, et al. Sex differences in morphine analgesia: an experimental study in healthy volunteers. Anesthesiology. 2000;93:1245–1254, discussion 6A. 51. Bouillon T, Shafer SL. Does size matter? Anesthesiology. 1998;89: 557–560. 52. Egan TD, Huizinga B, Gupta SK, et al. Remifentanil pharmacokinetics in obese versus lean patients. Anesthesiology. 1998;89:562–573. 53. Kim TK, Obara S, Egan TD, et al. Disposition of remifentanil in obesity: a new pharmacokinetic model incorporating the influence of body mass. Anesthesiology. 2017;126:1019–1032. 54. Egan TD, Kuramkote S, Gong G, et al. Fentanyl pharmacokinetics in hemorrhagic shock: a porcine model. Anesthesiology. 1999;91:156–166. 55. Johnson KB, Kern SE, Hamber EA, et al. Influence of hemorrhagic shock on remifentanil: a pharmacokinetic and pharmacodynamic analysis. Anesthesiology. 2001;94:322–332. 56. Honan DM, Breen PJ, Boylan JF, et al. Decrease in bispectral index preceding intraoperative hemodynamic crisis: evidence of acute alteration of propofol pharmacokinetics. Anesthesiology. 2002;97: 1303–1305. 57. Poulsen L, Brosen K, Arendt-Nielsen L, et al. Codeine and morphine in extensive and poor metabolizers of sparteine: pharmacokinetics, analgesic effect and side effects. Eur J Clin Pharmacol. 1996;51:289–295. 58. Caraco Y, Sheller J, Wood AJ. Pharmacogenetic determination of the effects of codeine and prediction of drug interactions. J Pharmacol Exp Ther. 1996;278:1165–1174. 59. Eckhardt K, Li S, Ammon S, et al. Same incidence of adverse drug events after codeine administration irrespective of the genetically determined differences in morphine formation. Pain. 1998;76:27–33. 60. Caraco Y, Sheller J, Wood AJ. Impact of ethnic origin and quinidine coadministration on codeine’s disposition and pharmacodynamic effects. J Pharmacol Exp Ther. 1999;290:413–422. 61. Aklillu E, Persson I, Bertilsson L, et al. Frequent distribution of ultrarapid metabolizers of debrisoquine in an ethiopian population carrying duplicated and multiduplicated functional CYP2D6 alleles. J Pharmacol Exp Ther. 1996;278:441–446. 62. Madadi P, Ross CJ, Hayden MR, et al. Pharmacogenetics of neonatal opioid toxicity following maternal use of codeine during breastfeeding: a case-control study. Clin Pharmacol Ther. 2009;85:31–35. 63. Kelly LE, Rieder M, van den Anker J, et al. More codeine fatalities after tonsillectomy in North American children. Pediatrics. 2012;129:e1343–e1347. 64. Crews KR, Gaedigk A, Dunnenberger HM, Clinical Pharmacogenetics Implementation Consortium, et al. Clinical Pharmacogenetics Implementation Consortium guidelines for cytochrome P450 2D6 genotype and codeine therapy: 2014 update. Clin Pharmacol Ther. 2014;95:376–382. 65. Lotsch J, Dudziak R, Freynhagen R, et al. Fatal respiratory depression after multiple intravenous morphine injections. Clin Pharmacokinet. 2006;45:1051–1060. 66. Osborne R, Joel S, Trew D, et al. Morphine and metabolite behavior after different routes of morphine administration: demonstration of the importance of the active metabolite morphine-6-glucuronide. Clin Pharmacol Ther. 1990;47:12–19. 67. Price DD, Mayer DJ, Mao J, et al. NMDA-receptor antagonists and opioid receptor interactions as related to analgesia and tolerance. J Pain Symptom Manage. 2000;19:S7–S11. 68. Morgan RW, Nicholson KL. Characterization of the antinociceptive effects of the individual isomers of methadone after acute and chronic administrations. Behav Pharmacol. 2011;22:548–557. 69. Leppert W. The role of methadone in cancer pain treatment–a review. Int J Clin Pract. 2009;63:1095–1109. 70. Andrews CM, Krantz MJ, Wedam EF, et al. Methadone-induced mortality in the treatment of chronic pain: role of QT prolongation. Cardiol J. 2009;16:210–217. 71. Streisand JB, Varvel JR, Stanski DR, et al. Absorption and bioavailability of oral transmucosal fentanyl citrate. Anesthesiology. 1991;75:223–229. 72. Stanski DR, Hug CC Jr. Alfentanil–a kinetically predictable narcotic analgesic. Anesthesiology. 1982;57:435–438. 73. Ibrahim AE, Feldman J, Karim A, et al. Simultaneous assessment of drug interactions with low- and high-extraction opioids: application to parecoxib effects on the pharmacokinetics and pharmacodynamics of fentanyl and alfentanil. Anesthesiology. 2003;98:853–861. 74. Egan TD. Remifentanil pharmacokinetics and pharmacodynamics. A preliminary appraisal. Clin Pharmacokinet. 1995;29:80–94. 75. Egan TD, Lemmens HJ, Fiset P, et al. The pharmacokinetics of the new short-acting opioid remifentanil (GI87084B) in healthy adult male volunteers. Anesthesiology. 1993;79:881–892. 76. Egan TD, Minto CF, Hermann DJ, et al. Remifentanil versus alfentanil: comparative pharmacokinetics and pharmacodynamics in healthy adult male volunteers. Anesthesiology. 1996;84:821–833. [published erratum appears in Anesthesiology 1996 Sep;85(3):695]. 77. Egan TD. The clinical pharmacology of remifentanil: a brief review. J Anesth. 1998;12:195–204. 78. Gal TJ, DiFazio CA, Moscicki J. Analgesic and respiratory depressant activity of nalbuphine: a comparison with morphine. Anesthesiology. 1982;57:367–374. 79. Murphy MR, Hug CC Jr. The enflurane sparing effect of morphine, butorphanol, and nalbuphine. Anesthesiology. 1982;57:489–492. 80. Errick JK, Heel RC. Nalbuphine. A preliminary review of its pharmacological properties and therapeutic efficacy. Drugs. 1983;26:191–211. 81. Strain EC, Preston KL, Liebson IA, et al. Precipitated withdrawal by pentazocine in methadone-maintained volunteers. J Pharmacol Exp Ther. 1993;267:624–634. 82. Chen KY, Chen L, Mao J. Buprenorphine-naloxone therapy in pain management. Anesthesiology. 2014;120:1262–1274. 83. Jasinski DR, Martin WR, Haertzen CA. The human pharmacology and abuse potential of N-allylnoroxymorphone (naloxone). J Pharmacol Exp Ther. 1967;157:420–426. 84. Jasinski DR, Martin WR, Sapira JD. Antagonism of the subjective, behavioral, pupillary, and respiratory depressant effects of cyclazocine by naloxone. Clin Pharmacol Ther. 1968;9:215–222. 85. Olofsen E, van Dorp E, Teppema L, et al. Naloxone reversal of morphine- and morphine-6-glucuronide-induced respiratory depression in healthy volunteers: a mechanism-based pharmacokineticpharmacodynamic modeling study. Anesthesiology. 2010;112: 1417–1427. 86. Andree RA. Sudden death following naloxone administration. Anesth Analg. 1980;59:782–784. 87. Edwards ET, Edwards ES, Davis E, et al. Comparative usability study of a novel auto-injector and an intranasal system for naloxone delivery. Pain Ther. 2015;4:89–105. 88. Krieter P, Chiang N, Gyaw S, et al. Pharmacokinetic properties and human use characteristics of an FDA approved intranasal naloxone product for the treatment of opioid overdose. J Clin Pharmacol. 2016;56:1243–1253. 89. Kunoe N, Lobmaier P, Ngo H, et al. Injectable and implantable sustained release naltrexone in the treatment of opioid addiction. Br J Clin Pharmacol. 2014;77:264–271. 90. Lowenstein E, Hallowell P, Levine FH, et al. Cardiovascular response to large doses of intravenous morphine in man. N Engl J Med. 1969;281:1389–1393. 91. Lunn JK, Stanley TH, Eisele J, et al. High dose fentanyl anesthesia for coronary artery surgery: plasma fentanyl concentrations and influence of nitrous oxide on cardiovascular responses. Anesth Analg. 1979;58:390–395. Downloaded for Vicente Gonzalez ([email protected]) at Florida International University from ClinicalKey.com by Elsevier on April 29, 2024. For personal use only. No other uses without permission. Copyright ©2024. Elsevier Inc. All rights reserved. CHAPTER 17 92. Hofer CK, Zollinger A, Buchi S, et al. Patient well-being after general anaesthesia: a prospective, randomized, controlled multi-centre trial comparing intravenous and inhalation anaesthesia. Br J Anaesth. 2003;91:631–637. 93. Mather LE. Pharmacokinetic and pharmacodynamic profiles of opioid analgesics: a sameness amongst equals? Pain. 1990;43:3–6. 94. Egan TD. Target-controlled drug delivery: progress toward an intravenous “vaporizer” and automated anesthetic administration. Anesthesiology. 2003;99:1214–1219. 95. Egan TD, Shafer SL. Target-controlled infusions for intravenous anesthetics: surfing USA not! Anesthesiology. 2003;99:1039–1041. 96. Biki B, Mascha E, Moriarty DC, et al. Anesthetic technique for radical prostatectomy surgery affects cancer recurrence: a retrospective analysis. Anesthesiology. 2008;109:180–187. 97. Cronin-Fenton DP, Heide-Jorgensen U, Ahern TP, et al. Opioids and breast cancer recurrence: a Danish population-based cohort study. Cancer. 2015;121:3507–3514. 98. Cao L, Chang Y, Lin W, et al. Long-term survival after resection of hepatocelluar carcinoma: a potential risk associated with the choice of postoperative analgesia. Anesth Analg. 2014;118:1309–1316. 99. Wang K, Qu X, Wang Y, et al. Effect of mu agonists on long-term survival and recurrence in nonsmall cell lung cancer patients. Medicine (Baltimore). 2015;94:e1333. 100. Bovill JG. Surgery for cancer: does anesthesia matter? Anesth Analg. 2010;110:1524–1526. 101. Connolly C, Buggy DJ. Opioids and tumour metastasis: does the choice of the anesthetic-analgesic technique influence outcome after cancer surgery? Curr Opin Anaesthesiol. 2016;29:468–474. 102. Bohn LM, Gainetdinov RR, Lin FT, et al. Mu-opioid receptor desensitization by beta-arrestin-2 determines morphine tolerance but not dependence. Nature. 2000;408:720–723. 103. Bohn LM, Lefkowitz RJ, Gainetdinov RR, et al. Enhanced morphine analgesia in mice lacking beta-arrestin 2. Science. 1999;286:2495–2498. 104. Raehal KM, Walker JK, Bohn LM. Morphine side effects in beta-arrestin 2 knockout mice. J Pharmacol Exp Ther. 2005;314:1195–1201. 105. Manglik A, Lin H, Aryal DK, et al. Structure-based discovery of opioid analgesics with reduced side effects. Nature. 2016;537: 185–190. 106. Thomas J, Karver S, Cooney GA, et al. Methylnaltrexone for opioid-induced constipation in advanced illness. N Engl J Med. 2008;358:2332–2343. 107. Ludwig K, Enker WE, Delaney CP, et al. Gastrointestinal tract recovery in patients undergoing bowel resection: results of a randomized trial of alvimopan and placebo with a standardized accelerated postoperative care pathway. Arch Surg. 2008;143:1098–1105. 108. Ford AC, Brenner DM, Schoenfeld PS. Efficacy of pharmacological therapies for the treatment of opioid-induced constipation: systematic review and meta-analysis. Am J Gastroenterol. 2013;108:1566–1574. 109. Argoff CE, Brennan MJ, Camilleri M, et al. Consensus recommendations on initiating prescription therapies for opioid-induced constipation. Pain Med. 2015;16:2324–2337. 110. Davis JJ, Swenson JD, Hall RH, et al. Preoperative “fentanyl challenge” as a tool to estimate postoperative opioid dosing in chronic opioid-consuming patients. Anesth Analg. 2005;101:389–395. 111. Konopka KH, van Wijhe M. Opioid-induced hyperalgesia: pain hurts? Br J Anaesth. 2010;105:555–557. 112. Chu LF, Angst MS, Clark D. Opioid-induced hyperalgesia in humans: molecular mechanisms and clinical considerations. Clin J Pain. 2008;24:479–496. 113. Schmidt S, Bethge C, Forster MH, et al. Enhanced postoperative sensitivity to painful pressure stimulation after intraoperative high dose remifentanil in patients without significant surgical site pain. Clin J Pain. 2007;23:605–611. Intravenous Opioid Agonists and Antagonists 353 114. Guignard B, Bossard AE, Coste C, et al. Acute opioid tolerance: intraoperative remifentanil increases postoperative pain and morphine requirement. Anesthesiology. 2000;93:409–417. 115. Angst MS, Chu LF, Tingle MS, et al. No evidence for the development of acute tolerance to analgesic, respiratory depressant and sedative opioid effects in humans. Pain. 2009;142:17–26. 116. Cortinez LI, Brandes V, Munoz HR, et al. No clinical evidence of acute opioid tolerance after remifentanil-based anaesthesia. Br J Anaesth. 2001;87:866–869. 117. Lee LH, Irwin MG, Lui SK. Intraoperative remifentanil infusion does not increase postoperative opioid consumption compared with 70% nitrous oxide. Anesthesiology. 2005;102:398–402. 118. Shin SW, Cho AR, Lee HJ, et al. Maintenance anaesthetics during remifentanil-based anaesthesia might affect postoperative pain control after breast cancer surgery. Br J Anaesth. 2010;105:661–667. 119. Singler B, Troster A, Manering N, et al. Modulation of remifentanilinduced postinfusion hyperalgesia by propofol. Anesth Analg. 2007;104:1397–1403. 120. Yu EH, Tran DH, Lam SW, et al. Remifentanil tolerance and hyperalgesia: short-term gain, long-term pain? Anaesthesia. 2016;71:1347–1362. 121. Joly V, Richebe P, Guignard B, et al. Remifentanil-induced postoperative hyperalgesia and its prevention with small-dose ketamine. Anesthesiology. 2005;103:147–155. 122. Chu LF, Dairmont J, Zamora AK, et al. The endogenous opioid system is not involved in modulation of opioid-induced hyperalgesia. J Pain. 2011;12:108–115. 123. Trang T, Al-Hasani R, Salvemini D, et al. Pain and poppies: the good, the bad, and the ugly of opioid analgesics. J Neurosci. 2015;35: 13879–13888. 124. Heinl C, Drdla-Schutting R, Xanthos DN, et al. Distinct mechanisms underlying pronociceptive effects of opioids. J Neurosci. 2011;31: 16748–16756. 125. Corder G, Tawfik VL, Wang D, et al. Loss of mu opioid receptor signaling in nociceptors, but not microglia, abrogates morphine tolerance without disrupting analgesia. Nat Med. 2017;23:164–173. 126. Rudd RA, Aleshire N, Zibbell JE, et al. Increases in drug and opioid overdose deaths–United States, 2000-2014. MMWR Morb Mortal Wkly Rep. 2016;64:1378–1382. 127. Brady KT, McCauley JL, Back SE. Prescription opioid misuse, abuse, and treatment in the United States: an update. Am J Psychiatry. 2016;173:18–26. 128. Weisberg DF, Becker WC, Fiellin DA, et al. Prescription opioid misuse in the United States and the United Kingdom: cautionary lessons. Int J Drug Policy. 2014;25:1124–1130. 129. Johnson SR. The opioid abuse epidemic: how healthcare helped create a crisis. Mod Healthc. 2016;46:8–9. 130. Dowell D, Kunins HV, Farley TA. Opioid analgesics–risky drugs, not risky patients. JAMA. 2013;309:2219–2220. 131. Gostin LO, Hodge JG Jr, Noe SA. Reframing the opioid epidemic as a national emergency. JAMA. 2017;318:1539–1540. 132. Kharasch ED, Brunt LM. Perioperative opioids and public health. Anesthesiology. 2016;124:960–965. 133. Office Of The Press Secretary The White House. President Obama proposes $1.1 billion in new funding to address the prescription opioid abuse and heroin use epidemic. J Pain Palliat Care Pharmacother. 2016;30:134–137. 134. Bachyrycz A, Shrestha S, Bleske BE, et al. Opioid overdose prevention through pharmacy-based naloxone prescription program: innovations in healthcare delivery. Subst Abus. 2017;38:55–60. 135. Frieden TR, Houry D. Reducing the risks of relief–the CDC Opioid-Prescribing Guideline. N Engl J Med. 2016;374:1501–1504. 136. Murthy VH. Ending the opioid epidemic - a call to action. N Engl J Med. 2016;375:2413–2415. Downloaded for Vicente Gonzalez ([email protected]) at Florida International University from ClinicalKey.com by Elsevier on April 29, 2024. For personal use only. No other uses without permission. Copyright ©2024. Elsevier Inc. All rights reserved.